DOI: https://doi.org/10.56669/LALQ5306
ABSTRACT
Bacillus species are well known for their beneficial effects on plant growth promotion and biocontrol of plant diseases. Since they are able to produce endospores, they are allowed to resist adverse environments, and make them easily be formulated as a stable dry powder with a long shelf life for manufacturing into commercial products. Bacillus velezensis strain EN01 was isolated from green onion, which showed a number of beneficial characteristics for biocontrol applications. It especially presented excellent antibacterial activity on Acidovorax citrulli (Aac), the causal agent of bacterial fruit blotch (BFB) of cucurbit plants. The volatile organic compounds (VOCs) of EN01 could effectively reduce the infection of BFB in melon via decreasing the biofilm formation and twitching ability of Aac. While EN01 was formulated with the proper amount of Asteraceae plant extract (AP) showed synergistic biocontrol activity for BFB on muskmelon seedlings. Both the disease severity and population of Aac significantly declined compared with those of the control. According to the results of enzymatic and histochemical staining assays, EN01 formulated with AP was capable to attach root surface and decrease the disease index of BFB on melon seedlings through inducing the accumulation of plant defense enzymes, such as peroxidase and phenylalanine ammonia-lyase (PAL). Overall, B. velezensis EN01 formulated with AP could serve as a promising biological control agent for sustainable agriculture application.
Keywords: Bacillus velezensis, biological control, biofilm formation, twitching ability, plant probiotics, Asteraceae, bacterial fruit blotch (BFB), plant defense, hydrogen peroxide, lignin.
INTRODUCTION
Melon belongs to cucurbit crops and is a popular fruit grown commercially throughout the world. On the other hand, they are very susceptible to several abiotic stress (e.g. drastic temperature or injury) or biotic stress caused by pathogens. A number of diseases have been associated with melon, including bacterial fruit blotch (BFB) caused by Acidovorax citrulli (Aac, formerly called Acidovorax avenae subsp. citrulli), bacterial wilt caused by Erwinia tracheiphila, downy mildew caused by Pseudoperonospora cubensis, gummy stem blight caused by Didymella bryoniae, and powdery mildew caused by Podosphaera xanthii or Sphearotheca fuliginea (Pan and More, 1996, Bokshi et al., 2007, Zhang et al., 1997, Rojas and Gleason, 2012, Burdman and Walcott, 2012).
Among these diseases, BFB is a serious threat to melons worldwide(Bahar et al., 2009, Schaad et al., 2008). The pathogen A. citrulli is mainly transmitted by seeds and infects the cucurbit plant at all stages of growth, resulting in seedling lesions, blight or fruit rot (Hopkins and Thompson, 2002, Popović and Ivanović, 2015). There is only one recommended bactericide for the treatment of BFB in Taiwan (40% of oxine and copper with cupric hydroxide) by the Bureau of Animal and Plant Health Inspection and Quarantine (http://portal.tactri.gov.tw/) (Institute of Drugs and Toxicology, Council of Agriculture, Executive Yuan, 2020), and some studies also found that pre-treatment of seeds with kasugamycin-copper oxychloride, streptomycin with tetracycline, or 1% HCl could effectively reduce seed-borne Aac(Cheng et al., 2000, Hopkins et al., 1996). However, even after treating seeds with chemicals, the pathogen Aac cannot be completely eliminated from seeds, which means pre-treated seeds remain susceptible to infection to some extent. Considering the difficulties associated with controlling BFB and its highly destructive potential, it is essential to adopt alternative strategies to prevent and cure BFB.
The microbial inoculants of plant growth-promoting rhizobacteria (PGPR) have been widely used in agriculture as biological control agents or biofertilizers (Alori and Babalola, 2018). This strategy is being expanded and has the potential to replace the chemical methods for preventing pests or pathogens. In addition, these biocontrol agents can also enhance crop growth, increase crop yields, and fertilize soils (Beneduzi et al., 2012). A wide variety of antagonistic microorganisms, such as Bacillus species, Streptomyces species, and Pseudomonas species have been reported to possess promising potential against plant pathogens (Fan et al., 2017, Yaeram et al., 2006).
Bacillus spp. are gram-positive, spore-forming, and rod-shaped bacteria, which can produce many kinds of active secondary metabolites (Ahmad et al., 2008). A broad spectrum of structurally unrelated antimicrobial compounds, such as lipopeptides iturin, surfactin, fengycins, and bacteriocins are synthesized by these bacteria, which are capable of against pathogens (Ongena et al., 2007). On the other hand, Bacillus spp. are easily formulated and commercialized as biocontrol agents because of their fast growth, and ability to grow and sporulate under undesirable conditions.
An edible Asteraceae plant (AP) is traditionally used as herbal medicine and beverage called cool tea for its ability to clear away heat, stop thirst, and relieve fever (Li et al., 2014). In medical science, it has demonstrated several benefits, including anti-diabetic, anti-virus, anti-inflammatory, anti-cancer, and anti-microbe properties
(Chiang et al., 2003, Chang et al., 2005, Shandukani et al., 2018, Sundararajan et al., 2006, Yang, 2014). In addition, it inhibits the growth of plant pathogens, such as Sclerotium rolfsii, which causes southern blight; Fusarium solani, which causes crown and root rot; Fusarium oxysporum, which causes Fusarium wilt; and Xanthomonas campestris pv. vesicatoria, which causes bacterial leaf spot on peppers and tomatoes (Deba et al., 2008, Emitaro, 2017) It has also been used in agriculture as an environmentally friendly disease and pest control agent, decreasing protozoan parasite populations, which are responsible for 12.5-fold eimeriosis in broilers, killing arthropod pests specifically, and increasing the yield of pigeon peas and cowpeas by 65% to 230% compared to control(Sundararajan et al., 2006, Chang et al., 2016, Tembo et al., 2018).
In recent years, B. velezensis has been studied for its possible use as biopesticides(Grady et al., 2019). We previously isolated a B. velezensis strain EN01 from green onion, and found that it possessed the potential to solubilize phosphate and against plant pathogens. In this study, we would like to evaluate its plant growth-promoting and antimicrobial activities on bacteria fruit blotch diseases (BFB), and elucidate the associated mechanisms.
MATERIALS AND METHODS
Preparation of Bacillus spp. strains
Bacterial strains were cultured in Luria-Bertani broth (LB). The pH of the medium was adjusted to 7.0 with NaOH and the bacteria were precultured at 28°C at 200 rpm for 24 hours. In the following step, 1.0% inoculum was added to Erlenmeyer flask that containing 50ml LB broth. After overnight incubation, the individual bacterial broth was diluted and adjust with spectrophotometer, OD600=1.0 and 0.3 for Bacillus spp. strains and pathogenic bacteria, respectively.
Preparation of edible Asteraceae plant extract (AP)
An edible Asteraceae plant extract was prepared in Dr. Wen Chin Yang's lab. A sterilized ddH2O solution was used to dissolve the crude extract, which was then shaken at 150 rpm for 10 minutes at room temperature. Insoluble compounds were removed by filtering through 22 µm filters and centrifuging at 10,000 g for 5 minutes at room temperature. Lastly, the supernatant (AP) was sterilized by passing through a 0.22 µm PES membrane filter and transferred to a new centrifuge tube. The AP stock was diluted in sterile ddH2O for further usage.
Identification of a newly isolated bacterial strain EN01
The bacteria strain EN01 was isolated from Allium fistulosum at Yonghe, New Taipei City, Taiwan (R.O.C). For traditional method, the bacterial strains were grown on LA medium for 3 days and observed through a dissecting microscope. In addition, the genomic DNA of bacterial strain was extracted by Genomic DNA mini kit (Geneaid Biotech Ltd., Taiwan) according to the instruction manual. The 16S rDNA (1375 bp) and gyrB (1326 bp) were amplified by primer 27F, 1492R, gyrB-1730R, respectively
(Huang et al., 2016, Lane, 1996, Yamamoto and Harayama, 1995). PCR products were prepared for Sanger sequencing. Individual 16S rDNA gene of the bacterial strains was compared against National Center for Biotechnology Information (NCBI) database reported sequences with the Basic Local Alignment Search Tool (BLAST) hosted at the website of NCBI (https://blast.ncbi.nlm.nih.gov/Blast.cgi). A phylogenetic tree was then drawn using the neighbor-joining method. Phylogenetic and molecular evolutionary analyses were conducted at 1000 bootstrap value using Molecular Evolutionary Genetics Analysis (MEGA) version X software (Kumar et al., 2018).
Characterization of the plant growth-promoting, antimicrobial and other beneficial traits of Bacillus spp. strains (in-vitro)
The enzyme assays presented below were conducted according to the published methods. Paper discs with a diameter of 6 mm were used. A minimum of two and three technical replicates were conducted for each experiment.
Amylase assay was conducted by using a starch agar plate (Adhikari et al., 2017). Three percentages of iodine were added to the plate and the clear zone formed on the medium was measured after incubation. The lipase assay was conducted according to the procedure developed by Hankin and Anagnostakis (1975) and the white precipitation around the inoculant was observed after incubation. Proteinase assay was conducted with Skim agar plate (Adhikari et al., 2017). A clear zone around the inoculant appeared, indicating the proteinase was produced by EN01. Cellulase assay was conducted and the yellow zone around the inoculant was formed, indicating the bacteria can produce cellulose (Peristiwati et al., 2018). Phosphate solubilization ability was examined according to the procedure developed by (Mehta and Nautiyal, 2001). Ca3(PO4)2 were changed as to AlPO4 in order to test different types of insoluble phosphate existed in natural environments. When the color around the inoculant changed from purple to white or yellow after 7 days of incubation, the strain was determined to be capable of secreting phosphatase. The phosphate solubilization ability was calculated through the following formula as described by Jayadi (2013): The phosphate solubilization ability (%) = The diameter of the solubilization halo zone ÷ The diameter of the colony x 100. Urease assay was conducted according to the procedure developed by Li et al. (2018). While the color of broth medium change from yellow to pink presents a positive reaction. Indole-3-Acetic Acid (IAA) production ability of bacteria was measured by colorimetric analysis and record the optical density at 530nm following the method described by (Mohite, 2013). Pectinase and proteinase assay was conducted according to the procedure developed by Jacob et al. (2008).
Siderophore production assay was experimented in Chrome azurol S (CAS) agar plate (Arora and Verma, 2017). Siderophore production was considered positive when a yellow-orange halo developed around the inoculant. Chitinase assay was conducted according to the procedure developed by (Saima and RoohiI, 2013). The clear zones around the colonies on a cream background indicate that the bacteria is capable of producing chitinase.
The antimicrobial activities of Bacillus spp. strains (in-vitro)
The antagonistic activities were evaluated by dual culture test using a paper disc method (6 mm diameter of paper discs). The ampicillin (100 μg per paper disc) and cycloheximide (250 μg per paper disc) were chosen as a positive control for bacterial and fungal pathogens, respectively. The LB was used as a negative control. The antimicrobial activities against bacterial pathogen A. citrulli (Aac) was measured by the diameter of the clear zone. A minimum of two and three technical replicates were conducted for each experiment.
The antimicrobial activities of volatile organic compounds (VOCs) derived from Bacillus spp. strains
A small petri dish (6 cm in diameter) containing LA medium was placed into a larger dish (9 cm in diameter) that also containing LA medium. Two µL of EN01 inoculum was amended with 300 ppm AP (final concentration) or not, and then spotted on the LA in the 9 cm petri dish. On the other hand, 2 µL of Aac was spotted on the LA in the 6 cm petri dish. In order to avoid the emission of VOCs, the lid of the container was wrapped with double layers of parafilm M and the container was incubated at 28°C. After that, the colony width and the twitching motility were observed under an bright-field microscope and measured by ImageJ (Schneider et al., 2012). The population of Aac was determined by serial dilution. Later, a modification protocol was used to test the biofilm formation of bacterial pathogens to verify the effect of VOCs. A minimum of two and three technical replicates were conducted for each experiment.
Root colonization abilities of B. velezensis strain EN01 and mixture
In the in vitro assay, muskmelon seeds were sterilized with 5% NaOCl for 15 minutes before being rinsed three times with sterilized ddH2O. Seeds were sterilized and placed on 1/2 MS medium with 0.6% agar, and then incubated in darkness at 28°C for 2-3 days until germinating. The 2 µL bacterial cultured broth (OD600=0.1) was amended with 300 ppm AP (final concentration in broth) was dropped below the root tip approximately 0.5 cm (I.J.Misaghi, 1990). After 1 day of incubation, colonies were observed by a bright field microscope and the Gram staining method was performed to verify the colonization ability on the root surface.
In the in-vivo assay, roots were sampled from the individual plants at 1, 6, and 13 days after the inoculation of EN01, EN01+AP, respectively. The cultured broth (OD600=1.0) and 300 ppm AP (final concentration in broth) were used. In brief, the roots were cleaned with tap water in order to remove any attached matrix. The roots were transferred into a 50 mL tube containing 30 mL sterilized ddH2O and then shaken for 5 minutes at 120 rpm. The roots were gently wiped with sterilized paper towels to remove water and were then weighted. Lastly, the roots were ground up with a mortar and pestle and the mixture was prepared for serial dilutions and bacterial counts. There were at least two replications of the experiment and three technical replicates.
Biocontrol efficacy of bacterial suspension in plant with pot evaluation
(i) Irrigation
The melon seedlings (day 14 after sowing) were pre-treated with the suspension derived from respective Bacillus strain EN01 (20 mL/plant, OD600=1.0) contained 300 ppm AP (final concentration in broth) or not by irrigation, which was 5 days prior to the foliar spray of the pathogen (Aac; OD600=0.3). On the other hand, a chemical bactericide treatment with oxine-copper + cupric hydroxide according to manual instruction (Ruei-Fang plant protection Co., Ltd., Taiwan) was applied on leaves after 3 days post pathogen treatment. Plant traits (aboveground parts) were determined after 7 days of treatment. Meanwhile, disease index (also known as disease severity) and biocontrol efficacy were determined. Disease index was determined according to the percentage of symptomatic leaves. A scale from 0 to 6 was set: 0=no symptom; 1=10% or less symptomatic leaves; 2–5=11–25%, 26–50%, 51–75%, and 76–90% symptomatic leaves, respectively; and 6= more than 90% symptomatic leave (Fig. 8)
(Bahar et al., 2009)
. Furthermore, we calculated the disease index (called disease severity also) and biocontrol efficacy based on previous study
(Shi et al., 2012)
. Each treatment was undergoing at least three biological replicates and this experiment replicated at least three times.
(ii) Volatile organic compounds
After 7 days of planting, the muskmelon seedling was transferred to 3 inch pots. A pot and sterilized container were combined with petri dishes with a diameter of 6 cm containing 100µl of EN01. To avoid the emission of VOCs, at least double layer parafilm M was used to seal the junction. Upon exposure to VOCs for five days, the aboveground of plant was inoculated with AAC, and the results were harvested after 7 days of inoculation. The calculation of disease index and biocontrol efficacy was similar to the irrigation assay.
Quantification of defense-related compounds and enzymes
The 3,3’-diaminobenzidine tetrahydrochloride and toluidine blue O were used to visualize H2O2 and phenolic compounds accumulation in leaves, respectively (O'Brien et al., 1964, Christensen et al., 1997). The activities of peroxidase (POD) and phenylalanine ammonia-lyase (PAL) were determined following the procedures described by Ge et al. (2013). All experiments were repeated twice with three biological replicates.
Statistical analysis
All data were analyzed by ANOVA and significant differences at p<0.05 among treatments were determined by Scheffe’s test using SAS® 9.4 software (SAS Institute Inc., USA).
RESULTS AND DISCUSSION
Identification and characterization of EN01
The morphologies of EN01 were observed by stereomicroscope and optical microscope. EN01 formed a flat colony, and its cell length was in the range of 3.0-7.0 µm (Fig. 1). This strain belonged to Gram-positive bacteria through the Gram staining method and was able to form endospores (Fig. 1). Further, genomic DNA of EN01 strain was extracted and a molecular method with marker gene was performed to identify this isolate. Two housekeeping gene 16S rRNA and gyrB were chosen.
Based on the partial sequences of 16S rRNA shown in Fig. 2A, EN01 belonged to B. amyloliquefaciens subsp. amyloliquefaciens, B. siamensis, and B. velezensis clusters. In order to further distinguish EN01 from B. amyloliquefaciens subsp amyloliquefaciens, the partial sequence of the gyrB gene was used to further distinguish the species among the Bacillus genus. As shown in Fig. 2B, the group with the type strain of B. velezensis (i.e FZB42T) was obviously separated from the type strain of B. amyloliquefaciens (i.e DSM7T), accordingly, we deduced the species name of strain EN01 should be designated as B. velezensis rather than B. amyloliquefaciens. In addition, although B. subtilus Y1336 and B. amyloliquefaciens WF02 were included in the B. velezensis group, their species name should be revised in according to the new phylogenetic identification.
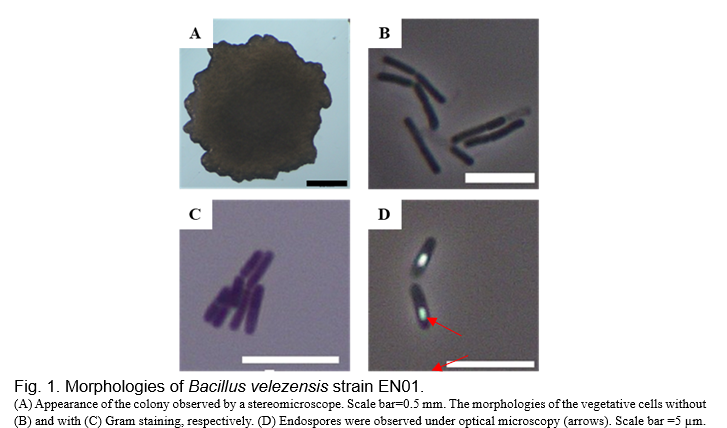
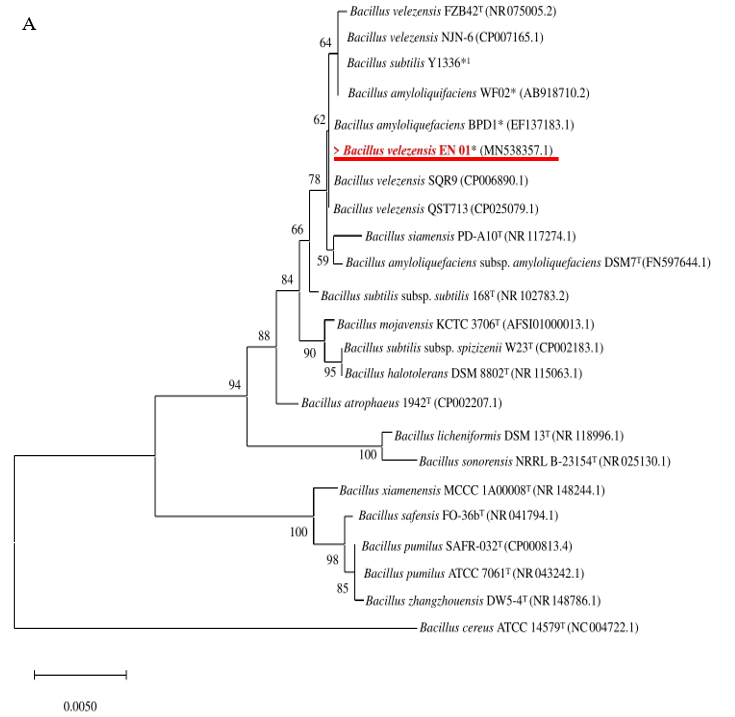
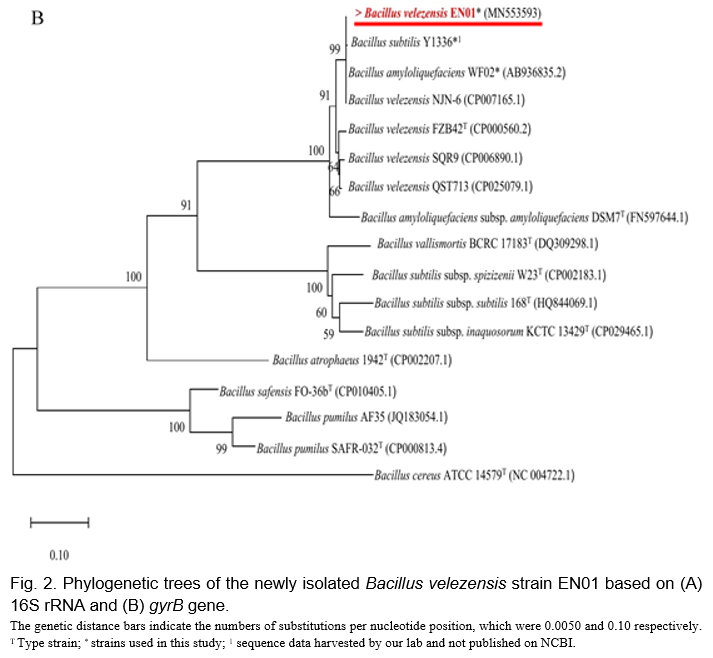
In general, phosphate solubilization, siderophore production, hydrolytic enzyme activity, etc., have been considered as the in vitro plant growth-promoting (PGP) traits. We conducted a series of assays to determine the PGP traits of EN01. As shown in Table 1, EN01 showed higher degrees of activities in most PGP traits, such as amylase, proteinase, lipase, and phosphatase production. Besides, this bacterium has also shown the ability of siderophore production.
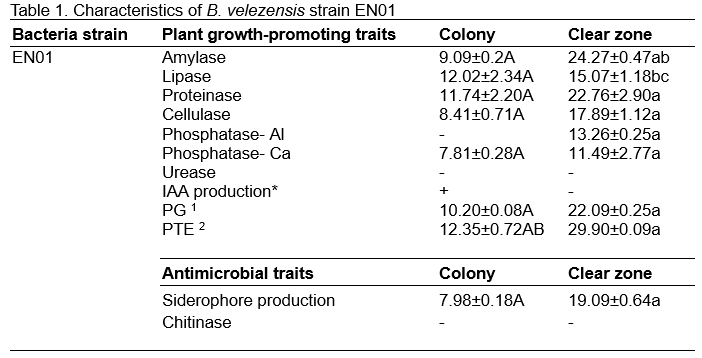
The diameter of the paper discs was 6 mm and the final concentration of B. velezensis strain EN01 for this experiment was OD600=1.0. +: Mean the positive reaction. -: Mean the colony did not grow outside the paper disc or negative reaction respectively. Unit: millimeter (mm). *: Mean the bacterial strain can produce IAA but the concentration below 5 ppm. 1: Polygalacturonase. 2: Pectate transeliminase. Superscript uppercase letters mean the statistic of the colony width; lowercase means the statistic of the clear zone width; All statistics result according to Scheffe’s test with p<0.05. Numbers represented the mean ± standard deviation (S.D.).
Antagonistic activities against plant pathogenic bacteria
To verify whether strain EN01 was able to antagonize A. citrulli (Aac), the antimicrobial activities were evaluated by a dual culture test using a paper disc method (6 mm diameter of paper discs) and measured by the diameter of the clear zone. As shown in Fig. 3, the vegetative cells of EN01 showed the ability to against pathogen Aac and the effectiveness was similar to chemical drug treatment.
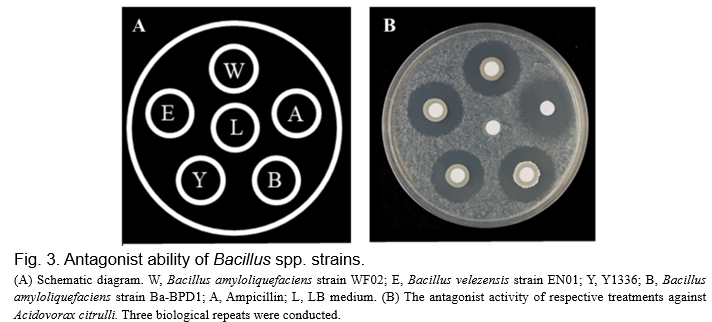
In addition to the active compounds secreted by the vegetative cells to antagonize the bacteria pathogen, previous literature also revealed that some of the volatile organic compounds (VOCs) produced by Bacillus subtilis strain (GB03) could aid plants in resisting pathogen attacks (Ryu et al., 2004), such as 2,4-di-tert-butylphenol, 1-octanol, benzothiazole, albuterol, and 1,3-propanediole
(Gao et al., 2018, Fincheira and Quiroz, 2018). Therefore, we set up the dual culture plate assay for evaluating the antibacterial activities of the VOCs derived from EN01 strains against Aac. As shown in Fig. 4, we found that the colony size as well as the population of Aac were not affected by the VOCs of EN01. However, there are two features that are considered to be related to the pathogenicity of gram-negative pathogens, such as biofilm and twitching ability
(Tahir et al., 2017, Shrestha et al., 2013). We noticed that the biofilm formation ability of Aac was inhibited by the VOCs derived from EN01 (Fig. 5). Moreover, the addition of AP to EN01 could further decrease the Aac biofilm formation by 44.4% (Fig. 5). Notably, the VOCs derived from EN01 in the presence of AP showed a higher inhibitory effect on the twitching motility of Aac (88%) than those derived from EN01 without AP addition (94%) (Fig. 6).
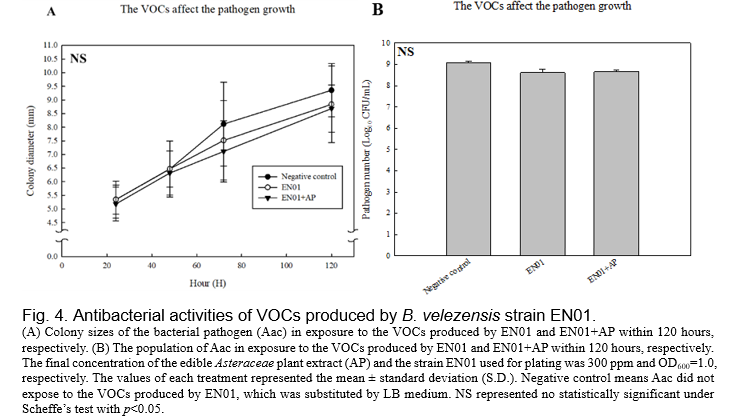
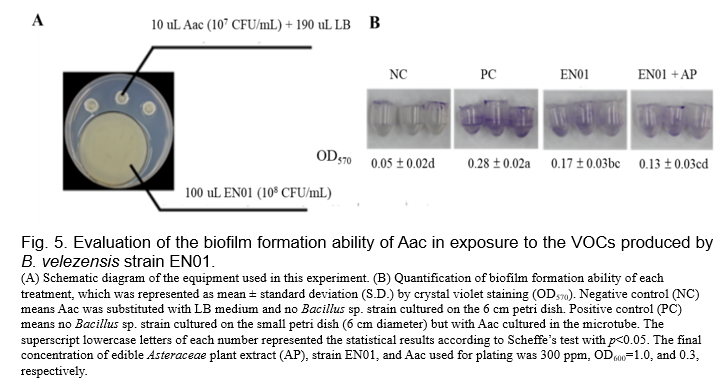
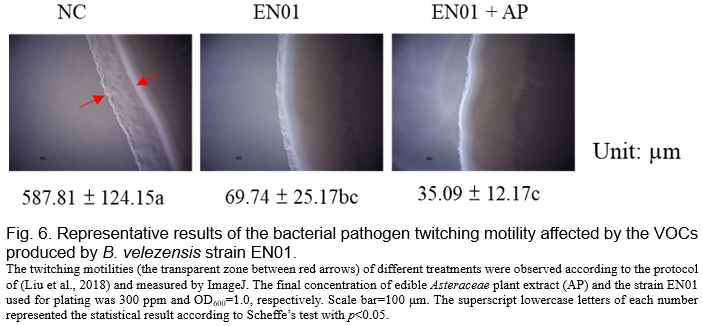
We deduced that the growth of Aac was directly inhibited by the compounds secreted by EN01's vegetative cells and volatile organic compounds, resulting in the decrease of biofilm formation and twitching ability. Notably, we considered that the contents or the amounts of the effective compounds in the VOCs were changed after the addition of AP. Whether there were novel compounds subsequently synthesized in EN01 due to the addition of AP remains to be elucidated.
Root colonization ability of EN01
There are many studies indicating that the beneficial effects of PGPR on plant protection or plant growth promotion are caused by the plant-microbe interactions (Romano et al., 2020). These interactions occurred through microbes living in the surrounding soil and colonizing the surface of the roots or entering into root tissues (Romano et al., 2020). To evaluate the root colonization abilities of strain EN01, an in vitro assay was conducted by inoculating with strain EN01 and observing before and after Gram staining (Fig 7A-B). EN01 cells were accumulated on the surrounding of the root surface of the muskmelon young seedlings (Fig. 7A-B). Likewise, we enumerated EN01 cells colonized on the oriental melon roots. As shown in Fig. 7C, the population of EN01 was gradually increased after the inoculation of the 1st day (7.6x104 CFU/g) to that of the 5th day (7.6x106 CFU/g). It might be due to the nutrient input at 1 dpi and the EN01 cells needed time to adapt to the new environment. That of the 13th day (3.7x106 CFU/g) was not significantly changed compared with that of the 5th day. By adding AP to the broth, the population of the strain did not change remarkably, indicating that AP did not have an adverse effect on EN01. On the other hand, at 13th day, the population of Aac pathogen in the aboveground plant among treatments was determined by the plating method. While treating EN01 with or without AP in plant roots could effectively suppress the population of Aac at least 1 log CFU/g as compared to the control (Fig. 7D). As mentioned above, AP was suggested as a safe additive for EN01 growth, and we hypothesized that EN01 formulated with AP could attach to root surfaces and reduce the severity of BFB by suppressing the population of the pathogen.
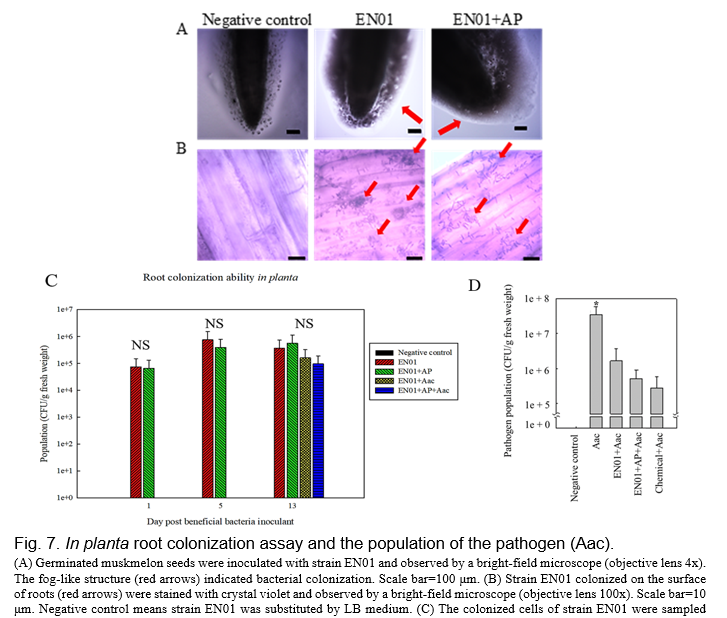
Biocontrol test in planta and putative plant defense mechanisms
We inoculated melon seedlings with EN01 broth (108 CFU/mL) prior to the infection of Aac, and found that the pretreated plants showed better biocontrol efficacy (Fig. 8). We also inoculated melon with the mixture of EN01 (108 CFU/mL) and AP before Aac infection, and observed higher biocontrol efficacy (~76%) than that without pretreatment (i.e Aac only) (Fig. 8). On the other hand, the addition of AP did not affect the biocontrol efficacy of individual treatments significantly (data not shown). As mentioned above, the growth of Aac was also inhibited by the volatile organic compounds of EN01 (Fig. 4). Thus, a pot experiment was conducted to determine whether EN01's emission of VOCs could reduce BFB severity in the presence of melon seedlings. Not surprisingly, as shown in Fig. 8, the bacteria VOCs treatment resulted in 2.5 times (24.17÷9.72) lower disease index of Aac compared with no inoculation treatment. Depending on the biocontrol efficacy of EN01 on melon seedlings, various methods (irrigation or VOCs) can be used to reduce the BFB disease by EN01.
It has been known that peroxidase and lignin play important roles in helping plants against phytopathogens infection(Narayanasamy, 2013)
. These enzymes do not directly eliminate the pathogens but induce the plant to form structural barriers, such as lignin, to prevent infection of pathogens
(Lee et al., 2019)
. We determined the activities of peroxidase (POD) and phenylalanine ammonia-lyase (PAL) following the procedures described by
(Ge et al., 2013)
, and found that the concentration of peroxidase was elevated in the plant leaves at 1-day post inoculation (dpi) with Aac inoculation (Fig. 9A). At 3 dpi, treatments with strain EN01 still exhibited higher concentration of
peroxidase
than those solely with Aac. Lignin was one downstream product of phenylalanine ammonia-lyase (PAL)
(Boerjan et al., 2003)
. We determined the enzyme activities of PAL under respective treatments. As shown in Fig. 9B, the activity of the PAL at EN01 or EN01+AP treatment was higher than those of either healthy seedlings or Aac infected seedlings at 1 dpi, which were dramatically decreased after 2 dpi, but the mean value still higher than the Aac infected seedlings.
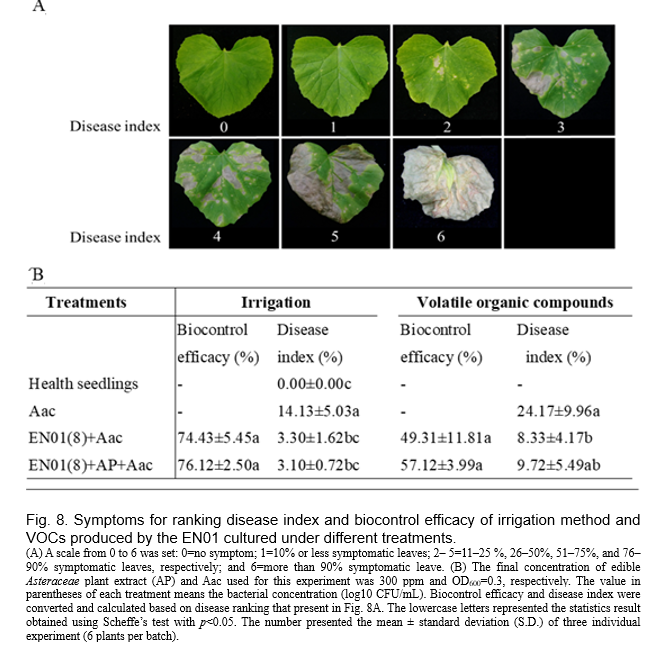
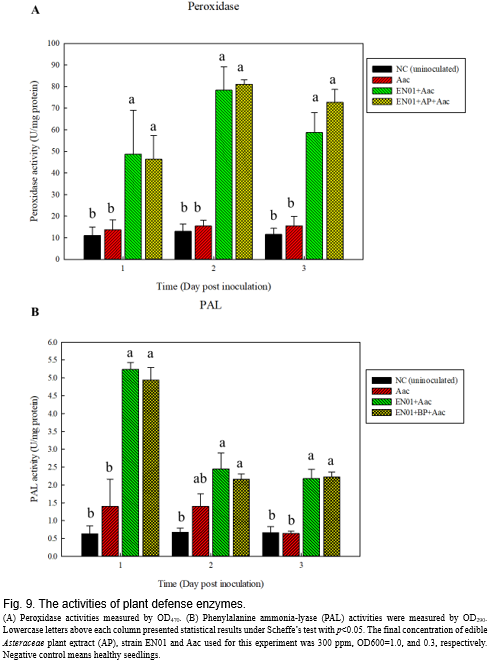
CONCLUSION
In this study, we reported that Bacillus velezensis EN01 could effectively inhibit the growth of Acidovorax citrulli with either active metabolites or volatile organic compounds (Fig. 10). When EN01 was formulated with AP, the antimicrobial activity on phytopathogens (e.g Aac) was significantly enhanced (Fig. 10). Accordingly, we deduced that the plant defense-related compounds, such as peroxidase and PAL, were synthesized synergistically to alleviate the severity of BFB disease (Fig. 10). Accordingly, formulating B. velezensis EN01 with proper amount of AP could serve as a promising biological control agent for sustainable agriculture application.
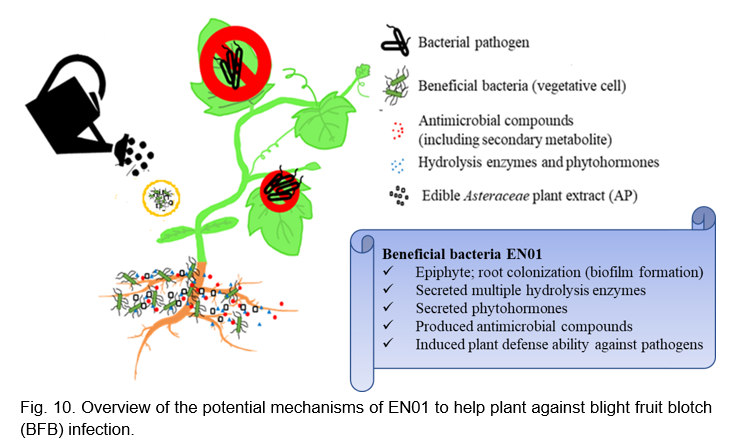
REFERENCES
Adhikari, M., Yadav, D. R., Kim, S. W., Um, Y. H., Kim, H. S., Lee, S. C., Song, J. Y., Kim, H. G. & Lee, Y. S. (2017). Biological control of bacterial fruit blotch of watermelon pathogen (Acidovorax citrulli) with rhizosphere associated bacteria. The Plant Pathogen Journal, 33, 170-183.
Ahmad, F., Ahmad, I. & Khan, M. S. (2008). Screening of free-living rhizospheric bacteria for their multiple plant growth promoting activities. Microbioogical Research, 163, 173-81.
Alori, E. T. & Babalola, O. O. (2018). Microbial Inoculants for Improving Crop Quality and Human Health in Africa. Frontiers in Microbiology, 9.
Arora, N. K. & Verma, M. (2017). Modified microplate method for rapid and efficient estimation of siderophore produced by bacteria. 3 Biotech, 7, 381-381.
Bahar, O., Kritzman, G. & Burdman, S. (2009). Bacterial fruit blotch of melon: screens for disease tolerance and role of seed transmission in pathogenicity. European Journal of Plant Pathology, 123, 71-83.
Beneduzi, A., Ambrosini, A. & Passaglia, L. M. (2012). Plant growth-promoting rhizobacteria (PGPR): their potential as antagonists and biocontrol agents. Genetics and molecular biology, 35, 1044-1051.
Boerjan, W., Ralph, J. & Baucher, M. (2003). Lignin biosynthesis. Annual Review of Plant Biology, 54, 519-546.
Bokshi, A. I., Morris, S. C. & Mcconchie, R. (2007). Environmentally‐safe control of postharvest diseases of melons (Cucumis melo) by integrating heat treatment, safe chemicals, and systemic acquired resistance. New Zealand Journal of Crop and Horticultural Science, 35, 179-186.
Burdman, S. & Walcott, R. (2012). Acidovorax citrulli: Generating basic and applied knowledge to tackle a global threat to the cucurbit industry. Molecular Plant Pathology, 13, 805-815.
Chang, C. L. T., Kuo, H. K., Chang, S. L., Chiang, Y. M., Lee, T. H., Wu, W. M., Shyur, L. F. & Yang, W. C. (2005). The distinct effects of a butanol fraction of Bidens pilosa plant extract on the development of Th1-mediated diabetes and Th2-mediated airway inflammation in mice. Journal of Biomedical Science, 12, 79-89.
Chang, C. L. T., Yang, C. Y., Muthamilselvan, T. & Yang, W. C. (2016). Field trial of medicinal plant, Bidens pilosa, against eimeriosis in broilers. Scientific Reports, 6, 24692.
Cheng, A., Hsu, Y., Huang, T. & Wang, H. (2000). Susceptibility of cucurbits to Acidovorax avenae subsp. citrulli and control of fruit blotch on melon. Plant Pathology Bulletin, 9, 151-156.
Chiang, L. C., Chang, J. S., Chen, C. C., Ng, L. T. N. & Lin, C. C. (2003). Anti-Herpes simplex virus activity of Bidens pilosa and Houttuynia cordata. The American Journal of Chinese Medicine, 31, 355-362.
Christensen, H. T., Zhang, Z., Wei, Y. & Collinge, D. B. (1997). Subcellular localization of H2O2 in plants. H2O2 accumulation in papillae and hypersensitive response during the barley—powdery mildew interaction. The Plant Journal, 11, 1187-1194.
Deba, F., Xuan, T. D., Yasuda, M. & Tawata, S. (2008). Chemical composition and antioxidant, antibacterial and antifungal activities of the essential oils from Bidens pilosa Linn. var. radiata. Food Control, 19, 346-352.
Emitaro, O. W. (2017). Characterization of Xanthomonas campestris pv.vesicatoria In African Nightshades (Solanum Scabrum Mill.) and its control using extracts of Bidens pilosa L. and Euphorbia hirta L. [Online]. Kenya: Maseno University. Available: https://repository.maseno.ac.ke/handle/123456789/58 [Accessed].
Fan, H., Zhang, Z., Li, Y., Zhang, X., Duan, Y. & Wang, Q. (2017). Biocontrol of bacterial fruit blotch by Bacillus subtilis 9407 via surfactin-mediated antibacterial activity and colonization. Frontiers in Microbiology, 8, 1973.
Fincheira, P. & Quiroz, A. (2018). Microbial volatiles as plant growth inducers. Microbiological Research, 208, 63-75.
Gao, H., Li, P., Xu, X., Zeng, Q. & Guan, W. (2018). Research on volatile organic compounds from Bacillus subtilis CF-3: Biocontrol effects on fruit fungal pathogens and dynamic changes during fermentation. Frontiers in Microbiology, 9, 456.
Ge, Y., Bi, Y. & Guest, D. I. (2013). Defence responses in leaves of resistant and susceptible melon (Cucumis melo L.) cultivars infected with Colletotrichum lagenarium. Physiological and Molecular Plant Pathology, 81, 13-21.
Grady, E. N., Macdonald, J., Ho, M. T., Weselowski, B., Mcdowell, T., Solomon, O., Renaud, J. & Yuan, Z.-C. (2019). Characterization and complete genome analysis of the surfactin-producing, plant-protecting bacterium Bacillus velezensis 9D-6. BMC Microbiology, 19, 5.
Hankin, L. & Anagnostakis, S. L. (1975). The use of solid media for detection of enzyme production by fungi. Mycologia, 67, 597-607.
Hopkins, D., Cucuzza, J. & Watterson, J. (1996). Wet seed treatments for the control of bacterial fruit blotch of watermelon. Plant Disease, 80, 529-532.
Hopkins, D. & Thompson, C. (2002). Seed transmission of Acidovorax avenae subsp. citrulli in cucurbits. HortScience, 37, 924-926.
Huang, C. N., Lin, C. P., Hsieh, F. C., Lee, S. K., Cheng, K. C. & Liu, C. T. (2016). Characterization and evaluation of Bacillus amyloliquefaciens strain WF02 regarding its biocontrol activities and genetic responses against bacterial wilt in two different resistant tomato cultivars. World Journal of Microbiology and Biotechnology, 32, 183.
I.J.Misaghi (1990). Screening bacteria for root colonizing ability by a rapid method. Soil Biology and Biochemistry, 22, 1085-1088.
Jacob, N., Niladevi, K. N., Anisha, G. S. & Prema, P. (2008). Hydrolysis of pectin: An enzymatic approach and its application in banana fiber processing. Microbiological Research, 163, 538-544.
Jayadi, M. (2013). In Vitro selection of rock phosphate solubility by microorganism from ultisols in South Sulawesi, Indonesia. American Journal of Agriculture and Forestry, 1, 68-73.
Kumar, S., Stecher, G., Li, M., Knyaz, C. & Tamura, K. (2018). MEGA X: molecular evolutionary genetics analysis across computing platforms. Molecular Biology and Evolution, 35, 1547-1549.
Lane, D. J. (1996). 16S/23S rRNA sequencing. In: Stackebrandt, E. & Goodfellow, M. (eds.) Nucleic acid techniques in bacterial systematics. Chichester, England: John Wiley and Sons.
Lee, M. H., Jeon, H. S., Kim, S. H., Chung, J. H., Roppolo, D., Lee, H. J., Cho, H. J., Tobimatsu, Y., Ralph, J. & Park, O. K. (2019). Lignin-based barrier restricts pathogens to the infection site and confers resistance in plants. The EMBO Journal, 38, e101948.
Li, S., Gan, L. Q., Li, S. K., Zheng, J. C., Xu, D. P. & Li, H. B. (2014). Effects of herbal infusions, tea and carbonated beverages on alcohol dehydrogenase and aldehyde dehydrogenase activity. Food and Function, 5, 42-49.
Li, Y., Li, Y., Wang, L. W. & Bao, J. (2018). Streptomyces dengpaensis sp. nov., an actinomycete isolated from desert soil. International Journal of Systematic and Evolutionary Microbiology, 68, 3322-3326.
Liu, Y., Wang, Z., Bilal, M., Hu, H., Wang, W., Huang, X., Peng, H. & Zhang, X. (2018). Enhanced fluorescent siderophore biosynthesis and loss of phenazine-1-carboxamide in phenotypic variant of Pseudomonas chlororaphis HT66. Frontiers in Microbiology, 9, 759.
Mehta, S. & Nautiyal, C. S. (2001). An efficient method for qualitative screening of phosphate-solubilizing bacteria. Current Microbiology, 43, 51-56.
Mohite, B. (2013). Isolation and characterization of indole acetic acid (IAA) producing bacteria from rhizospheric soil and its effect on plant growth. Journal of Soil Science and Plant Nutrition, 13, 638-649.
Narayanasamy, P. (2013). Mechanisms of action of bacterial biological control agents. Biological management of diseases of crops: Volume 1: Characteristics of biological control agents. Dordrecht: Springer Netherlands.
O'brien, T. P., Feder, N. & Mccully, M. E. (1964). Polychromatic staining of plant cell walls by toluidine blue O. Protoplasma, 59, 367-373.
Ongena, M., Jourdan, E., Adam, A., Paquot, M., Brans, A., Joris, B., Arpigny, J. L. & Thonart, P. (2007). Surfactin and fengycin lipopeptides of Bacillus subtilis as elicitors of induced systemic resistance in plants. Environmental Microbiology, 9, 1084-90.
Oni, F. E., Kieu Phuong, N. & Höfte, M. (2015). Recent advances in Pseudomonas biocontrol. Bacteria-plant interactions: Advanced research and future trends, 167-198.
Pan, R. S. & More, T. A. (1996). Screening of melon (Cucumis melo L.) germplasm for multiple disease resistance. Euphytica, 88, 125-128.
Peristiwati, Natamihardja, Y. S. & Herlini, H. (2018). Isolation and identification of cellulolytic bacteria from termites gut (Cryptotermes sp.). Journal of Physics: Conference Series, 1013, 012173.
Popović, T. & Ivanović, Ž. (2015). Occurrence of Acidovorax citrulli causing bacterial fruit blotch of watermelon in Serbia. Plant Disease, 99, 886-886.
Rojas, E. S. & Gleason, M. L. (2012). Epiphytic Survival of Erwinia tracheiphila on Muskmelon (Cucumis melo L.). Plant Disease, 96, 62-66.
Romano, I., Ventorino, V. & Pepe, O. (2020). Effectiveness of plant beneficial microbes: Overview of the methodological approaches for the assessment of root colonization and persistence. Frontiers in Plant Science, 11, 6.
Ryu, C.-M., Farag, M. A., Hu, C.-H., Reddy, M. S., Kloepper, J. W. & Paré, P. W. (2004). Bacterial volatiles induce systemic resistance in Arabidopsis. Plant physiology, 134, 1017-1026.
Sabaratnam, S. & Traquair, J. A. (2002). Formulation of a Streptomyces biocontrol agent for the suppression of Rhizoctonia damping-off in tomato transplants. Biological control, 23, 245-253.
Saima, M. K. & Roohii, Z. A. (2013). Isolation of novel chitinolytic bacteria and production optimization of extracellular chitinase. Journal of Genetic Engineering and Biotechnology, 11, 39-46.
Schaad, N. W., Postnikova, E., Sechler, A., Claflin, L. E., Vidaver, A. K., Jones, J. B., Agarkova, I., Ignatov, A., Dickstein, E. & Ramundo, B. A. (2008). Reclassification of subspecies of Acidovorax avenae as A. Avenae (Manns 1905) emend., A. cattleyae (Pavarino, 1911) comb. nov., A. citrulli Schaad et al., 1978) comb. nov., and proposal of A. oryzae sp. nov. Systematic and applied microbiology, 31, 434-446.
Schisler, D., Slininger, P., Behle, R. & Jackson, M. (2004). Formulation of Bacillus spp. for biological control of plant diseases. Phytopathology, 94, 1267-1271.
Schneider, C. A., Rasband, W. S. & Eliceiri, K. W. (2012). NIH Image to ImageJ: 25 years of image analysis. Nature Methods, 9, 671-675.
Shandukani, P. D., Tshidino, S. C., Masoko, P. & Moganedi, K. M. (2018). Antibacterial activity and in situ efficacy of Bidens pilosa Linn and Dichrostachys cinerea Wight et Arn extracts against common diarrhoea-causing waterborne bacteria. BMC Complementary and Alternative Medicine, 18, 171-171.
Shi, Y., Yang, L., Wang, X., Gao, Y., Liu, W. & Lou, K. (2012). Biocontrol of bacterial spot diseases of muskmelon using Paenibacillus polymyxa G-14. African Journal of Biotechnology, 11.
Shrestha, R. K., Rosenberg, T., Makarovsky, D., Eckshtain Levi, N., Zelinger, E., Kopelowitz, J., Sikorski, J. & Burdman, S. (2013). Phenotypic variation in the plant pathogenic bacterium Acidovorax citrulli. PLOS ONE, 8, e73189.
Sundararajan, P., Dey, A., Smith, A., Doss, A. G., Rajappan, M. & Natarajan, S. (2006). Studies of anticancer and antipyretic activity of Bidens pilosa whole plant. African health sciences, 6, 27-30.
Tahir, H. a. S., Gu, Q., Wu, H., Raza, W., Hanif, A., Wu, L., Colman, M. V. & Gao, X. (2017). Plant growth promotion by volatile organic compounds produced by Bacillus subtilis SYST2. Frontiers in Microbiology, 8, 171.
Tembo, Y., Mkindi, A. G., Mkenda, P. A., Mpumi, N., Mwanauta, R., Stevenson, P. C., Ndakidemi, P. A. & Belmain, S. R. (2018). Pesticidal plant extracts improve yield and reduce insect pests on legume crops without harming beneficial arthropods. Frontiers in Plant Science, 9, 1425.
Yaeram, C., Thummabenjapone, P. & Pachinburavan, A. (2006). Suitable medium for increasing biomass of antagonistic Streptomyces spp. against bacterial fruit blotch disease of watermelon. Khon Kaen Agric, 34, 12-19.
Yamamoto, S. & Harayama, S. (1995). PCR amplification and direct sequencing of gyrB genes with universal primers and their application to the detection and taxonomic analysis of Pseudomonas putida strains. Applied and Environmental Microbiology, 65, 1483-1490.
Yang, W. C. (2014). Botanical, pharmacological, phytochemical, and toxicological aspects of the antidiabetic plant Bidens pilosa L. Evidence-based Complementary and Alternative Medicine, 2014, 698617.
Zhang, Y., Kyle, M., Anagnostou, K. & Zitter, T. A. (1997). Screening melon (Cucumis melo) for resistance to gummy stem blight in the greenhouse and field. HortScience, 32, 117.
Biocontrol of Bacterial Fruit Blotch Disease by the Combination of Bacillus velezensis Strain EN01 and Edible Asteraceae Plant Extract
DOI: https://doi.org/10.56669/LALQ5306
ABSTRACT
Bacillus species are well known for their beneficial effects on plant growth promotion and biocontrol of plant diseases. Since they are able to produce endospores, they are allowed to resist adverse environments, and make them easily be formulated as a stable dry powder with a long shelf life for manufacturing into commercial products. Bacillus velezensis strain EN01 was isolated from green onion, which showed a number of beneficial characteristics for biocontrol applications. It especially presented excellent antibacterial activity on Acidovorax citrulli (Aac), the causal agent of bacterial fruit blotch (BFB) of cucurbit plants. The volatile organic compounds (VOCs) of EN01 could effectively reduce the infection of BFB in melon via decreasing the biofilm formation and twitching ability of Aac. While EN01 was formulated with the proper amount of Asteraceae plant extract (AP) showed synergistic biocontrol activity for BFB on muskmelon seedlings. Both the disease severity and population of Aac significantly declined compared with those of the control. According to the results of enzymatic and histochemical staining assays, EN01 formulated with AP was capable to attach root surface and decrease the disease index of BFB on melon seedlings through inducing the accumulation of plant defense enzymes, such as peroxidase and phenylalanine ammonia-lyase (PAL). Overall, B. velezensis EN01 formulated with AP could serve as a promising biological control agent for sustainable agriculture application.
Keywords: Bacillus velezensis, biological control, biofilm formation, twitching ability, plant probiotics, Asteraceae, bacterial fruit blotch (BFB), plant defense, hydrogen peroxide, lignin.
INTRODUCTION
Melon belongs to cucurbit crops and is a popular fruit grown commercially throughout the world. On the other hand, they are very susceptible to several abiotic stress (e.g. drastic temperature or injury) or biotic stress caused by pathogens. A number of diseases have been associated with melon, including bacterial fruit blotch (BFB) caused by Acidovorax citrulli (Aac, formerly called Acidovorax avenae subsp. citrulli), bacterial wilt caused by Erwinia tracheiphila, downy mildew caused by Pseudoperonospora cubensis, gummy stem blight caused by Didymella bryoniae, and powdery mildew caused by Podosphaera xanthii or Sphearotheca fuliginea (Pan and More, 1996, Bokshi et al., 2007, Zhang et al., 1997, Rojas and Gleason, 2012, Burdman and Walcott, 2012).
Among these diseases, BFB is a serious threat to melons worldwide(Bahar et al., 2009, Schaad et al., 2008). The pathogen A. citrulli is mainly transmitted by seeds and infects the cucurbit plant at all stages of growth, resulting in seedling lesions, blight or fruit rot (Hopkins and Thompson, 2002, Popović and Ivanović, 2015). There is only one recommended bactericide for the treatment of BFB in Taiwan (40% of oxine and copper with cupric hydroxide) by the Bureau of Animal and Plant Health Inspection and Quarantine (http://portal.tactri.gov.tw/) (Institute of Drugs and Toxicology, Council of Agriculture, Executive Yuan, 2020), and some studies also found that pre-treatment of seeds with kasugamycin-copper oxychloride, streptomycin with tetracycline, or 1% HCl could effectively reduce seed-borne Aac(Cheng et al., 2000, Hopkins et al., 1996). However, even after treating seeds with chemicals, the pathogen Aac cannot be completely eliminated from seeds, which means pre-treated seeds remain susceptible to infection to some extent. Considering the difficulties associated with controlling BFB and its highly destructive potential, it is essential to adopt alternative strategies to prevent and cure BFB.
The microbial inoculants of plant growth-promoting rhizobacteria (PGPR) have been widely used in agriculture as biological control agents or biofertilizers (Alori and Babalola, 2018). This strategy is being expanded and has the potential to replace the chemical methods for preventing pests or pathogens. In addition, these biocontrol agents can also enhance crop growth, increase crop yields, and fertilize soils (Beneduzi et al., 2012). A wide variety of antagonistic microorganisms, such as Bacillus species, Streptomyces species, and Pseudomonas species have been reported to possess promising potential against plant pathogens (Fan et al., 2017, Yaeram et al., 2006).
Bacillus spp. are gram-positive, spore-forming, and rod-shaped bacteria, which can produce many kinds of active secondary metabolites (Ahmad et al., 2008). A broad spectrum of structurally unrelated antimicrobial compounds, such as lipopeptides iturin, surfactin, fengycins, and bacteriocins are synthesized by these bacteria, which are capable of against pathogens (Ongena et al., 2007). On the other hand, Bacillus spp. are easily formulated and commercialized as biocontrol agents because of their fast growth, and ability to grow and sporulate under undesirable conditions.
An edible Asteraceae plant (AP) is traditionally used as herbal medicine and beverage called cool tea for its ability to clear away heat, stop thirst, and relieve fever (Li et al., 2014). In medical science, it has demonstrated several benefits, including anti-diabetic, anti-virus, anti-inflammatory, anti-cancer, and anti-microbe properties
(Chiang et al., 2003, Chang et al., 2005, Shandukani et al., 2018, Sundararajan et al., 2006, Yang, 2014). In addition, it inhibits the growth of plant pathogens, such as Sclerotium rolfsii, which causes southern blight; Fusarium solani, which causes crown and root rot; Fusarium oxysporum, which causes Fusarium wilt; and Xanthomonas campestris pv. vesicatoria, which causes bacterial leaf spot on peppers and tomatoes (Deba et al., 2008, Emitaro, 2017) It has also been used in agriculture as an environmentally friendly disease and pest control agent, decreasing protozoan parasite populations, which are responsible for 12.5-fold eimeriosis in broilers, killing arthropod pests specifically, and increasing the yield of pigeon peas and cowpeas by 65% to 230% compared to control(Sundararajan et al., 2006, Chang et al., 2016, Tembo et al., 2018).
In recent years, B. velezensis has been studied for its possible use as biopesticides(Grady et al., 2019). We previously isolated a B. velezensis strain EN01 from green onion, and found that it possessed the potential to solubilize phosphate and against plant pathogens. In this study, we would like to evaluate its plant growth-promoting and antimicrobial activities on bacteria fruit blotch diseases (BFB), and elucidate the associated mechanisms.
MATERIALS AND METHODS
Preparation of Bacillus spp. strains
Bacterial strains were cultured in Luria-Bertani broth (LB). The pH of the medium was adjusted to 7.0 with NaOH and the bacteria were precultured at 28°C at 200 rpm for 24 hours. In the following step, 1.0% inoculum was added to Erlenmeyer flask that containing 50ml LB broth. After overnight incubation, the individual bacterial broth was diluted and adjust with spectrophotometer, OD600=1.0 and 0.3 for Bacillus spp. strains and pathogenic bacteria, respectively.
Preparation of edible Asteraceae plant extract (AP)
An edible Asteraceae plant extract was prepared in Dr. Wen Chin Yang's lab. A sterilized ddH2O solution was used to dissolve the crude extract, which was then shaken at 150 rpm for 10 minutes at room temperature. Insoluble compounds were removed by filtering through 22 µm filters and centrifuging at 10,000 g for 5 minutes at room temperature. Lastly, the supernatant (AP) was sterilized by passing through a 0.22 µm PES membrane filter and transferred to a new centrifuge tube. The AP stock was diluted in sterile ddH2O for further usage.
Identification of a newly isolated bacterial strain EN01
The bacteria strain EN01 was isolated from Allium fistulosum at Yonghe, New Taipei City, Taiwan (R.O.C). For traditional method, the bacterial strains were grown on LA medium for 3 days and observed through a dissecting microscope. In addition, the genomic DNA of bacterial strain was extracted by Genomic DNA mini kit (Geneaid Biotech Ltd., Taiwan) according to the instruction manual. The 16S rDNA (1375 bp) and gyrB (1326 bp) were amplified by primer 27F, 1492R, gyrB-1730R, respectively
(Huang et al., 2016, Lane, 1996, Yamamoto and Harayama, 1995). PCR products were prepared for Sanger sequencing. Individual 16S rDNA gene of the bacterial strains was compared against National Center for Biotechnology Information (NCBI) database reported sequences with the Basic Local Alignment Search Tool (BLAST) hosted at the website of NCBI (https://blast.ncbi.nlm.nih.gov/Blast.cgi). A phylogenetic tree was then drawn using the neighbor-joining method. Phylogenetic and molecular evolutionary analyses were conducted at 1000 bootstrap value using Molecular Evolutionary Genetics Analysis (MEGA) version X software (Kumar et al., 2018).
Characterization of the plant growth-promoting, antimicrobial and other beneficial traits of Bacillus spp. strains (in-vitro)
The enzyme assays presented below were conducted according to the published methods. Paper discs with a diameter of 6 mm were used. A minimum of two and three technical replicates were conducted for each experiment.
Amylase assay was conducted by using a starch agar plate (Adhikari et al., 2017). Three percentages of iodine were added to the plate and the clear zone formed on the medium was measured after incubation. The lipase assay was conducted according to the procedure developed by Hankin and Anagnostakis (1975) and the white precipitation around the inoculant was observed after incubation. Proteinase assay was conducted with Skim agar plate (Adhikari et al., 2017). A clear zone around the inoculant appeared, indicating the proteinase was produced by EN01. Cellulase assay was conducted and the yellow zone around the inoculant was formed, indicating the bacteria can produce cellulose (Peristiwati et al., 2018). Phosphate solubilization ability was examined according to the procedure developed by (Mehta and Nautiyal, 2001). Ca3(PO4)2 were changed as to AlPO4 in order to test different types of insoluble phosphate existed in natural environments. When the color around the inoculant changed from purple to white or yellow after 7 days of incubation, the strain was determined to be capable of secreting phosphatase. The phosphate solubilization ability was calculated through the following formula as described by Jayadi (2013): The phosphate solubilization ability (%) = The diameter of the solubilization halo zone ÷ The diameter of the colony x 100. Urease assay was conducted according to the procedure developed by Li et al. (2018). While the color of broth medium change from yellow to pink presents a positive reaction. Indole-3-Acetic Acid (IAA) production ability of bacteria was measured by colorimetric analysis and record the optical density at 530nm following the method described by (Mohite, 2013). Pectinase and proteinase assay was conducted according to the procedure developed by Jacob et al. (2008).
Siderophore production assay was experimented in Chrome azurol S (CAS) agar plate (Arora and Verma, 2017). Siderophore production was considered positive when a yellow-orange halo developed around the inoculant. Chitinase assay was conducted according to the procedure developed by (Saima and RoohiI, 2013). The clear zones around the colonies on a cream background indicate that the bacteria is capable of producing chitinase.
The antimicrobial activities of Bacillus spp. strains (in-vitro)
The antagonistic activities were evaluated by dual culture test using a paper disc method (6 mm diameter of paper discs). The ampicillin (100 μg per paper disc) and cycloheximide (250 μg per paper disc) were chosen as a positive control for bacterial and fungal pathogens, respectively. The LB was used as a negative control. The antimicrobial activities against bacterial pathogen A. citrulli (Aac) was measured by the diameter of the clear zone. A minimum of two and three technical replicates were conducted for each experiment.
The antimicrobial activities of volatile organic compounds (VOCs) derived from Bacillus spp. strains
A small petri dish (6 cm in diameter) containing LA medium was placed into a larger dish (9 cm in diameter) that also containing LA medium. Two µL of EN01 inoculum was amended with 300 ppm AP (final concentration) or not, and then spotted on the LA in the 9 cm petri dish. On the other hand, 2 µL of Aac was spotted on the LA in the 6 cm petri dish. In order to avoid the emission of VOCs, the lid of the container was wrapped with double layers of parafilm M and the container was incubated at 28°C. After that, the colony width and the twitching motility were observed under an bright-field microscope and measured by ImageJ (Schneider et al., 2012). The population of Aac was determined by serial dilution. Later, a modification protocol was used to test the biofilm formation of bacterial pathogens to verify the effect of VOCs. A minimum of two and three technical replicates were conducted for each experiment.
Root colonization abilities of B. velezensis strain EN01 and mixture
In the in vitro assay, muskmelon seeds were sterilized with 5% NaOCl for 15 minutes before being rinsed three times with sterilized ddH2O. Seeds were sterilized and placed on 1/2 MS medium with 0.6% agar, and then incubated in darkness at 28°C for 2-3 days until germinating. The 2 µL bacterial cultured broth (OD600=0.1) was amended with 300 ppm AP (final concentration in broth) was dropped below the root tip approximately 0.5 cm (I.J.Misaghi, 1990). After 1 day of incubation, colonies were observed by a bright field microscope and the Gram staining method was performed to verify the colonization ability on the root surface.
In the in-vivo assay, roots were sampled from the individual plants at 1, 6, and 13 days after the inoculation of EN01, EN01+AP, respectively. The cultured broth (OD600=1.0) and 300 ppm AP (final concentration in broth) were used. In brief, the roots were cleaned with tap water in order to remove any attached matrix. The roots were transferred into a 50 mL tube containing 30 mL sterilized ddH2O and then shaken for 5 minutes at 120 rpm. The roots were gently wiped with sterilized paper towels to remove water and were then weighted. Lastly, the roots were ground up with a mortar and pestle and the mixture was prepared for serial dilutions and bacterial counts. There were at least two replications of the experiment and three technical replicates.
Biocontrol efficacy of bacterial suspension in plant with pot evaluation
(i) Irrigation
The melon seedlings (day 14 after sowing) were pre-treated with the suspension derived from respective Bacillus strain EN01 (20 mL/plant, OD600=1.0) contained 300 ppm AP (final concentration in broth) or not by irrigation, which was 5 days prior to the foliar spray of the pathogen (Aac; OD600=0.3). On the other hand, a chemical bactericide treatment with oxine-copper + cupric hydroxide according to manual instruction (Ruei-Fang plant protection Co., Ltd., Taiwan) was applied on leaves after 3 days post pathogen treatment. Plant traits (aboveground parts) were determined after 7 days of treatment. Meanwhile, disease index (also known as disease severity) and biocontrol efficacy were determined. Disease index was determined according to the percentage of symptomatic leaves. A scale from 0 to 6 was set: 0=no symptom; 1=10% or less symptomatic leaves; 2–5=11–25%, 26–50%, 51–75%, and 76–90% symptomatic leaves, respectively; and 6= more than 90% symptomatic leave (Fig. 8)
(Bahar et al., 2009) . Furthermore, we calculated the disease index (called disease severity also) and biocontrol efficacy based on previous study (Shi et al., 2012) . Each treatment was undergoing at least three biological replicates and this experiment replicated at least three times.(ii) Volatile organic compounds
After 7 days of planting, the muskmelon seedling was transferred to 3 inch pots. A pot and sterilized container were combined with petri dishes with a diameter of 6 cm containing 100µl of EN01. To avoid the emission of VOCs, at least double layer parafilm M was used to seal the junction. Upon exposure to VOCs for five days, the aboveground of plant was inoculated with AAC, and the results were harvested after 7 days of inoculation. The calculation of disease index and biocontrol efficacy was similar to the irrigation assay.
Quantification of defense-related compounds and enzymes
The 3,3’-diaminobenzidine tetrahydrochloride and toluidine blue O were used to visualize H2O2 and phenolic compounds accumulation in leaves, respectively (O'Brien et al., 1964, Christensen et al., 1997). The activities of peroxidase (POD) and phenylalanine ammonia-lyase (PAL) were determined following the procedures described by Ge et al. (2013). All experiments were repeated twice with three biological replicates.
Statistical analysis
All data were analyzed by ANOVA and significant differences at p<0.05 among treatments were determined by Scheffe’s test using SAS® 9.4 software (SAS Institute Inc., USA).
RESULTS AND DISCUSSION
Identification and characterization of EN01
The morphologies of EN01 were observed by stereomicroscope and optical microscope. EN01 formed a flat colony, and its cell length was in the range of 3.0-7.0 µm (Fig. 1). This strain belonged to Gram-positive bacteria through the Gram staining method and was able to form endospores (Fig. 1). Further, genomic DNA of EN01 strain was extracted and a molecular method with marker gene was performed to identify this isolate. Two housekeeping gene 16S rRNA and gyrB were chosen.
Based on the partial sequences of 16S rRNA shown in Fig. 2A, EN01 belonged to B. amyloliquefaciens subsp. amyloliquefaciens, B. siamensis, and B. velezensis clusters. In order to further distinguish EN01 from B. amyloliquefaciens subsp amyloliquefaciens, the partial sequence of the gyrB gene was used to further distinguish the species among the Bacillus genus. As shown in Fig. 2B, the group with the type strain of B. velezensis (i.e FZB42T) was obviously separated from the type strain of B. amyloliquefaciens (i.e DSM7T), accordingly, we deduced the species name of strain EN01 should be designated as B. velezensis rather than B. amyloliquefaciens. In addition, although B. subtilus Y1336 and B. amyloliquefaciens WF02 were included in the B. velezensis group, their species name should be revised in according to the new phylogenetic identification.
In general, phosphate solubilization, siderophore production, hydrolytic enzyme activity, etc., have been considered as the in vitro plant growth-promoting (PGP) traits. We conducted a series of assays to determine the PGP traits of EN01. As shown in Table 1, EN01 showed higher degrees of activities in most PGP traits, such as amylase, proteinase, lipase, and phosphatase production. Besides, this bacterium has also shown the ability of siderophore production.
The diameter of the paper discs was 6 mm and the final concentration of B. velezensis strain EN01 for this experiment was OD600=1.0. +: Mean the positive reaction. -: Mean the colony did not grow outside the paper disc or negative reaction respectively. Unit: millimeter (mm). *: Mean the bacterial strain can produce IAA but the concentration below 5 ppm. 1: Polygalacturonase. 2: Pectate transeliminase. Superscript uppercase letters mean the statistic of the colony width; lowercase means the statistic of the clear zone width; All statistics result according to Scheffe’s test with p<0.05. Numbers represented the mean ± standard deviation (S.D.).
Antagonistic activities against plant pathogenic bacteria
To verify whether strain EN01 was able to antagonize A. citrulli (Aac), the antimicrobial activities were evaluated by a dual culture test using a paper disc method (6 mm diameter of paper discs) and measured by the diameter of the clear zone. As shown in Fig. 3, the vegetative cells of EN01 showed the ability to against pathogen Aac and the effectiveness was similar to chemical drug treatment.
In addition to the active compounds secreted by the vegetative cells to antagonize the bacteria pathogen, previous literature also revealed that some of the volatile organic compounds (VOCs) produced by Bacillus subtilis strain (GB03) could aid plants in resisting pathogen attacks (Ryu et al., 2004), such as 2,4-di-tert-butylphenol, 1-octanol, benzothiazole, albuterol, and 1,3-propanediole
(Gao et al., 2018, Fincheira and Quiroz, 2018). Therefore, we set up the dual culture plate assay for evaluating the antibacterial activities of the VOCs derived from EN01 strains against Aac. As shown in Fig. 4, we found that the colony size as well as the population of Aac were not affected by the VOCs of EN01. However, there are two features that are considered to be related to the pathogenicity of gram-negative pathogens, such as biofilm and twitching ability
(Tahir et al., 2017, Shrestha et al., 2013). We noticed that the biofilm formation ability of Aac was inhibited by the VOCs derived from EN01 (Fig. 5). Moreover, the addition of AP to EN01 could further decrease the Aac biofilm formation by 44.4% (Fig. 5). Notably, the VOCs derived from EN01 in the presence of AP showed a higher inhibitory effect on the twitching motility of Aac (88%) than those derived from EN01 without AP addition (94%) (Fig. 6).
We deduced that the growth of Aac was directly inhibited by the compounds secreted by EN01's vegetative cells and volatile organic compounds, resulting in the decrease of biofilm formation and twitching ability. Notably, we considered that the contents or the amounts of the effective compounds in the VOCs were changed after the addition of AP. Whether there were novel compounds subsequently synthesized in EN01 due to the addition of AP remains to be elucidated.
Root colonization ability of EN01
There are many studies indicating that the beneficial effects of PGPR on plant protection or plant growth promotion are caused by the plant-microbe interactions (Romano et al., 2020). These interactions occurred through microbes living in the surrounding soil and colonizing the surface of the roots or entering into root tissues (Romano et al., 2020). To evaluate the root colonization abilities of strain EN01, an in vitro assay was conducted by inoculating with strain EN01 and observing before and after Gram staining (Fig 7A-B). EN01 cells were accumulated on the surrounding of the root surface of the muskmelon young seedlings (Fig. 7A-B). Likewise, we enumerated EN01 cells colonized on the oriental melon roots. As shown in Fig. 7C, the population of EN01 was gradually increased after the inoculation of the 1st day (7.6x104 CFU/g) to that of the 5th day (7.6x106 CFU/g). It might be due to the nutrient input at 1 dpi and the EN01 cells needed time to adapt to the new environment. That of the 13th day (3.7x106 CFU/g) was not significantly changed compared with that of the 5th day. By adding AP to the broth, the population of the strain did not change remarkably, indicating that AP did not have an adverse effect on EN01. On the other hand, at 13th day, the population of Aac pathogen in the aboveground plant among treatments was determined by the plating method. While treating EN01 with or without AP in plant roots could effectively suppress the population of Aac at least 1 log CFU/g as compared to the control (Fig. 7D). As mentioned above, AP was suggested as a safe additive for EN01 growth, and we hypothesized that EN01 formulated with AP could attach to root surfaces and reduce the severity of BFB by suppressing the population of the pathogen.
Biocontrol test in planta and putative plant defense mechanisms
We inoculated melon seedlings with EN01 broth (108 CFU/mL) prior to the infection of Aac, and found that the pretreated plants showed better biocontrol efficacy (Fig. 8). We also inoculated melon with the mixture of EN01 (108 CFU/mL) and AP before Aac infection, and observed higher biocontrol efficacy (~76%) than that without pretreatment (i.e Aac only) (Fig. 8). On the other hand, the addition of AP did not affect the biocontrol efficacy of individual treatments significantly (data not shown). As mentioned above, the growth of Aac was also inhibited by the volatile organic compounds of EN01 (Fig. 4). Thus, a pot experiment was conducted to determine whether EN01's emission of VOCs could reduce BFB severity in the presence of melon seedlings. Not surprisingly, as shown in Fig. 8, the bacteria VOCs treatment resulted in 2.5 times (24.17÷9.72) lower disease index of Aac compared with no inoculation treatment. Depending on the biocontrol efficacy of EN01 on melon seedlings, various methods (irrigation or VOCs) can be used to reduce the BFB disease by EN01.
It has been known that peroxidase and lignin play important roles in helping plants against phytopathogens infection(Narayanasamy, 2013)
. These enzymes do not directly eliminate the pathogens but induce the plant to form structural barriers, such as lignin, to prevent infection of pathogens (Lee et al., 2019) . We determined the activities of peroxidase (POD) and phenylalanine ammonia-lyase (PAL) following the procedures described by (Ge et al., 2013) , and found that the concentration of peroxidase was elevated in the plant leaves at 1-day post inoculation (dpi) with Aac inoculation (Fig. 9A). At 3 dpi, treatments with strain EN01 still exhibited higher concentration of peroxidase than those solely with Aac. Lignin was one downstream product of phenylalanine ammonia-lyase (PAL) (Boerjan et al., 2003) . We determined the enzyme activities of PAL under respective treatments. As shown in Fig. 9B, the activity of the PAL at EN01 or EN01+AP treatment was higher than those of either healthy seedlings or Aac infected seedlings at 1 dpi, which were dramatically decreased after 2 dpi, but the mean value still higher than the Aac infected seedlings.CONCLUSION
In this study, we reported that Bacillus velezensis EN01 could effectively inhibit the growth of Acidovorax citrulli with either active metabolites or volatile organic compounds (Fig. 10). When EN01 was formulated with AP, the antimicrobial activity on phytopathogens (e.g Aac) was significantly enhanced (Fig. 10). Accordingly, we deduced that the plant defense-related compounds, such as peroxidase and PAL, were synthesized synergistically to alleviate the severity of BFB disease (Fig. 10). Accordingly, formulating B. velezensis EN01 with proper amount of AP could serve as a promising biological control agent for sustainable agriculture application.
REFERENCES
Adhikari, M., Yadav, D. R., Kim, S. W., Um, Y. H., Kim, H. S., Lee, S. C., Song, J. Y., Kim, H. G. & Lee, Y. S. (2017). Biological control of bacterial fruit blotch of watermelon pathogen (Acidovorax citrulli) with rhizosphere associated bacteria. The Plant Pathogen Journal, 33, 170-183.
Ahmad, F., Ahmad, I. & Khan, M. S. (2008). Screening of free-living rhizospheric bacteria for their multiple plant growth promoting activities. Microbioogical Research, 163, 173-81.
Alori, E. T. & Babalola, O. O. (2018). Microbial Inoculants for Improving Crop Quality and Human Health in Africa. Frontiers in Microbiology, 9.
Arora, N. K. & Verma, M. (2017). Modified microplate method for rapid and efficient estimation of siderophore produced by bacteria. 3 Biotech, 7, 381-381.
Bahar, O., Kritzman, G. & Burdman, S. (2009). Bacterial fruit blotch of melon: screens for disease tolerance and role of seed transmission in pathogenicity. European Journal of Plant Pathology, 123, 71-83.
Beneduzi, A., Ambrosini, A. & Passaglia, L. M. (2012). Plant growth-promoting rhizobacteria (PGPR): their potential as antagonists and biocontrol agents. Genetics and molecular biology, 35, 1044-1051.
Boerjan, W., Ralph, J. & Baucher, M. (2003). Lignin biosynthesis. Annual Review of Plant Biology, 54, 519-546.
Bokshi, A. I., Morris, S. C. & Mcconchie, R. (2007). Environmentally‐safe control of postharvest diseases of melons (Cucumis melo) by integrating heat treatment, safe chemicals, and systemic acquired resistance. New Zealand Journal of Crop and Horticultural Science, 35, 179-186.
Burdman, S. & Walcott, R. (2012). Acidovorax citrulli: Generating basic and applied knowledge to tackle a global threat to the cucurbit industry. Molecular Plant Pathology, 13, 805-815.
Chang, C. L. T., Kuo, H. K., Chang, S. L., Chiang, Y. M., Lee, T. H., Wu, W. M., Shyur, L. F. & Yang, W. C. (2005). The distinct effects of a butanol fraction of Bidens pilosa plant extract on the development of Th1-mediated diabetes and Th2-mediated airway inflammation in mice. Journal of Biomedical Science, 12, 79-89.
Chang, C. L. T., Yang, C. Y., Muthamilselvan, T. & Yang, W. C. (2016). Field trial of medicinal plant, Bidens pilosa, against eimeriosis in broilers. Scientific Reports, 6, 24692.
Cheng, A., Hsu, Y., Huang, T. & Wang, H. (2000). Susceptibility of cucurbits to Acidovorax avenae subsp. citrulli and control of fruit blotch on melon. Plant Pathology Bulletin, 9, 151-156.
Chiang, L. C., Chang, J. S., Chen, C. C., Ng, L. T. N. & Lin, C. C. (2003). Anti-Herpes simplex virus activity of Bidens pilosa and Houttuynia cordata. The American Journal of Chinese Medicine, 31, 355-362.
Christensen, H. T., Zhang, Z., Wei, Y. & Collinge, D. B. (1997). Subcellular localization of H2O2 in plants. H2O2 accumulation in papillae and hypersensitive response during the barley—powdery mildew interaction. The Plant Journal, 11, 1187-1194.
Deba, F., Xuan, T. D., Yasuda, M. & Tawata, S. (2008). Chemical composition and antioxidant, antibacterial and antifungal activities of the essential oils from Bidens pilosa Linn. var. radiata. Food Control, 19, 346-352.
Emitaro, O. W. (2017). Characterization of Xanthomonas campestris pv.vesicatoria In African Nightshades (Solanum Scabrum Mill.) and its control using extracts of Bidens pilosa L. and Euphorbia hirta L. [Online]. Kenya: Maseno University. Available: https://repository.maseno.ac.ke/handle/123456789/58 [Accessed].
Fan, H., Zhang, Z., Li, Y., Zhang, X., Duan, Y. & Wang, Q. (2017). Biocontrol of bacterial fruit blotch by Bacillus subtilis 9407 via surfactin-mediated antibacterial activity and colonization. Frontiers in Microbiology, 8, 1973.
Fincheira, P. & Quiroz, A. (2018). Microbial volatiles as plant growth inducers. Microbiological Research, 208, 63-75.
Gao, H., Li, P., Xu, X., Zeng, Q. & Guan, W. (2018). Research on volatile organic compounds from Bacillus subtilis CF-3: Biocontrol effects on fruit fungal pathogens and dynamic changes during fermentation. Frontiers in Microbiology, 9, 456.
Ge, Y., Bi, Y. & Guest, D. I. (2013). Defence responses in leaves of resistant and susceptible melon (Cucumis melo L.) cultivars infected with Colletotrichum lagenarium. Physiological and Molecular Plant Pathology, 81, 13-21.
Grady, E. N., Macdonald, J., Ho, M. T., Weselowski, B., Mcdowell, T., Solomon, O., Renaud, J. & Yuan, Z.-C. (2019). Characterization and complete genome analysis of the surfactin-producing, plant-protecting bacterium Bacillus velezensis 9D-6. BMC Microbiology, 19, 5.
Hankin, L. & Anagnostakis, S. L. (1975). The use of solid media for detection of enzyme production by fungi. Mycologia, 67, 597-607.
Hopkins, D., Cucuzza, J. & Watterson, J. (1996). Wet seed treatments for the control of bacterial fruit blotch of watermelon. Plant Disease, 80, 529-532.
Hopkins, D. & Thompson, C. (2002). Seed transmission of Acidovorax avenae subsp. citrulli in cucurbits. HortScience, 37, 924-926.
Huang, C. N., Lin, C. P., Hsieh, F. C., Lee, S. K., Cheng, K. C. & Liu, C. T. (2016). Characterization and evaluation of Bacillus amyloliquefaciens strain WF02 regarding its biocontrol activities and genetic responses against bacterial wilt in two different resistant tomato cultivars. World Journal of Microbiology and Biotechnology, 32, 183.
I.J.Misaghi (1990). Screening bacteria for root colonizing ability by a rapid method. Soil Biology and Biochemistry, 22, 1085-1088.
Jacob, N., Niladevi, K. N., Anisha, G. S. & Prema, P. (2008). Hydrolysis of pectin: An enzymatic approach and its application in banana fiber processing. Microbiological Research, 163, 538-544.
Jayadi, M. (2013). In Vitro selection of rock phosphate solubility by microorganism from ultisols in South Sulawesi, Indonesia. American Journal of Agriculture and Forestry, 1, 68-73.
Kumar, S., Stecher, G., Li, M., Knyaz, C. & Tamura, K. (2018). MEGA X: molecular evolutionary genetics analysis across computing platforms. Molecular Biology and Evolution, 35, 1547-1549.
Lane, D. J. (1996). 16S/23S rRNA sequencing. In: Stackebrandt, E. & Goodfellow, M. (eds.) Nucleic acid techniques in bacterial systematics. Chichester, England: John Wiley and Sons.
Lee, M. H., Jeon, H. S., Kim, S. H., Chung, J. H., Roppolo, D., Lee, H. J., Cho, H. J., Tobimatsu, Y., Ralph, J. & Park, O. K. (2019). Lignin-based barrier restricts pathogens to the infection site and confers resistance in plants. The EMBO Journal, 38, e101948.
Li, S., Gan, L. Q., Li, S. K., Zheng, J. C., Xu, D. P. & Li, H. B. (2014). Effects of herbal infusions, tea and carbonated beverages on alcohol dehydrogenase and aldehyde dehydrogenase activity. Food and Function, 5, 42-49.
Li, Y., Li, Y., Wang, L. W. & Bao, J. (2018). Streptomyces dengpaensis sp. nov., an actinomycete isolated from desert soil. International Journal of Systematic and Evolutionary Microbiology, 68, 3322-3326.
Liu, Y., Wang, Z., Bilal, M., Hu, H., Wang, W., Huang, X., Peng, H. & Zhang, X. (2018). Enhanced fluorescent siderophore biosynthesis and loss of phenazine-1-carboxamide in phenotypic variant of Pseudomonas chlororaphis HT66. Frontiers in Microbiology, 9, 759.
Mehta, S. & Nautiyal, C. S. (2001). An efficient method for qualitative screening of phosphate-solubilizing bacteria. Current Microbiology, 43, 51-56.
Mohite, B. (2013). Isolation and characterization of indole acetic acid (IAA) producing bacteria from rhizospheric soil and its effect on plant growth. Journal of Soil Science and Plant Nutrition, 13, 638-649.
Narayanasamy, P. (2013). Mechanisms of action of bacterial biological control agents. Biological management of diseases of crops: Volume 1: Characteristics of biological control agents. Dordrecht: Springer Netherlands.
O'brien, T. P., Feder, N. & Mccully, M. E. (1964). Polychromatic staining of plant cell walls by toluidine blue O. Protoplasma, 59, 367-373.
Ongena, M., Jourdan, E., Adam, A., Paquot, M., Brans, A., Joris, B., Arpigny, J. L. & Thonart, P. (2007). Surfactin and fengycin lipopeptides of Bacillus subtilis as elicitors of induced systemic resistance in plants. Environmental Microbiology, 9, 1084-90.
Oni, F. E., Kieu Phuong, N. & Höfte, M. (2015). Recent advances in Pseudomonas biocontrol. Bacteria-plant interactions: Advanced research and future trends, 167-198.
Pan, R. S. & More, T. A. (1996). Screening of melon (Cucumis melo L.) germplasm for multiple disease resistance. Euphytica, 88, 125-128.
Peristiwati, Natamihardja, Y. S. & Herlini, H. (2018). Isolation and identification of cellulolytic bacteria from termites gut (Cryptotermes sp.). Journal of Physics: Conference Series, 1013, 012173.
Popović, T. & Ivanović, Ž. (2015). Occurrence of Acidovorax citrulli causing bacterial fruit blotch of watermelon in Serbia. Plant Disease, 99, 886-886.
Rojas, E. S. & Gleason, M. L. (2012). Epiphytic Survival of Erwinia tracheiphila on Muskmelon (Cucumis melo L.). Plant Disease, 96, 62-66.
Romano, I., Ventorino, V. & Pepe, O. (2020). Effectiveness of plant beneficial microbes: Overview of the methodological approaches for the assessment of root colonization and persistence. Frontiers in Plant Science, 11, 6.
Ryu, C.-M., Farag, M. A., Hu, C.-H., Reddy, M. S., Kloepper, J. W. & Paré, P. W. (2004). Bacterial volatiles induce systemic resistance in Arabidopsis. Plant physiology, 134, 1017-1026.
Sabaratnam, S. & Traquair, J. A. (2002). Formulation of a Streptomyces biocontrol agent for the suppression of Rhizoctonia damping-off in tomato transplants. Biological control, 23, 245-253.
Saima, M. K. & Roohii, Z. A. (2013). Isolation of novel chitinolytic bacteria and production optimization of extracellular chitinase. Journal of Genetic Engineering and Biotechnology, 11, 39-46.
Schaad, N. W., Postnikova, E., Sechler, A., Claflin, L. E., Vidaver, A. K., Jones, J. B., Agarkova, I., Ignatov, A., Dickstein, E. & Ramundo, B. A. (2008). Reclassification of subspecies of Acidovorax avenae as A. Avenae (Manns 1905) emend., A. cattleyae (Pavarino, 1911) comb. nov., A. citrulli Schaad et al., 1978) comb. nov., and proposal of A. oryzae sp. nov. Systematic and applied microbiology, 31, 434-446.
Schisler, D., Slininger, P., Behle, R. & Jackson, M. (2004). Formulation of Bacillus spp. for biological control of plant diseases. Phytopathology, 94, 1267-1271.
Schneider, C. A., Rasband, W. S. & Eliceiri, K. W. (2012). NIH Image to ImageJ: 25 years of image analysis. Nature Methods, 9, 671-675.
Shandukani, P. D., Tshidino, S. C., Masoko, P. & Moganedi, K. M. (2018). Antibacterial activity and in situ efficacy of Bidens pilosa Linn and Dichrostachys cinerea Wight et Arn extracts against common diarrhoea-causing waterborne bacteria. BMC Complementary and Alternative Medicine, 18, 171-171.
Shi, Y., Yang, L., Wang, X., Gao, Y., Liu, W. & Lou, K. (2012). Biocontrol of bacterial spot diseases of muskmelon using Paenibacillus polymyxa G-14. African Journal of Biotechnology, 11.
Shrestha, R. K., Rosenberg, T., Makarovsky, D., Eckshtain Levi, N., Zelinger, E., Kopelowitz, J., Sikorski, J. & Burdman, S. (2013). Phenotypic variation in the plant pathogenic bacterium Acidovorax citrulli. PLOS ONE, 8, e73189.
Sundararajan, P., Dey, A., Smith, A., Doss, A. G., Rajappan, M. & Natarajan, S. (2006). Studies of anticancer and antipyretic activity of Bidens pilosa whole plant. African health sciences, 6, 27-30.
Tahir, H. a. S., Gu, Q., Wu, H., Raza, W., Hanif, A., Wu, L., Colman, M. V. & Gao, X. (2017). Plant growth promotion by volatile organic compounds produced by Bacillus subtilis SYST2. Frontiers in Microbiology, 8, 171.
Tembo, Y., Mkindi, A. G., Mkenda, P. A., Mpumi, N., Mwanauta, R., Stevenson, P. C., Ndakidemi, P. A. & Belmain, S. R. (2018). Pesticidal plant extracts improve yield and reduce insect pests on legume crops without harming beneficial arthropods. Frontiers in Plant Science, 9, 1425.
Yaeram, C., Thummabenjapone, P. & Pachinburavan, A. (2006). Suitable medium for increasing biomass of antagonistic Streptomyces spp. against bacterial fruit blotch disease of watermelon. Khon Kaen Agric, 34, 12-19.
Yamamoto, S. & Harayama, S. (1995). PCR amplification and direct sequencing of gyrB genes with universal primers and their application to the detection and taxonomic analysis of Pseudomonas putida strains. Applied and Environmental Microbiology, 65, 1483-1490.
Yang, W. C. (2014). Botanical, pharmacological, phytochemical, and toxicological aspects of the antidiabetic plant Bidens pilosa L. Evidence-based Complementary and Alternative Medicine, 2014, 698617.
Zhang, Y., Kyle, M., Anagnostou, K. & Zitter, T. A. (1997). Screening melon (Cucumis melo) for resistance to gummy stem blight in the greenhouse and field. HortScience, 32, 117.