DOI: https://doi.org/10.56669/QXDL7420
ABSTRACT
Plant pathogens have been known to be controlled by Trichoderma species for decades. It is possible to use them either to enhance the health of crop plants or to increase the capacity for some plants to degrade toxic compounds, both in soil and water. Trichoderma species are well known for boosting plant growth and development, raised reproductive capability, capacity to alter the rhizosphere, ability to flourish under unfavorable conditions, proficiency in the use of nutrients, strong antagonistic against phytopathogenic fungi and increases defense mechanisms of the plants. Trichoderma strains can kill phytopathogens such as R solani. Utilizing the nutrients in the soil is a method of restricting the pathogens' access to them by means of aggression for nutrients. Trichoderma spp. are known to hinder the growth of other microbes by production of antibiotics and secondary metabolites. There is direct as well as indirect evidence indicating that secondary metabolites play a role in antagonistic activity of the Trichoderma spp. against various plant pathogens.
Keywords: biocontrol, Trichoderma, mechanisms, plant disease management
INTRODUCTION
Conventionally, Genus Trichoderma has been classified as Fungi Imperfecti. They give rise to only asexual spores i.e., conidia (Singh et al., 2006). With the recent advances in molecular taxonomy, many of the species have been reclassified as belonging to the genus Hypocrea of the class ascomycetes (Gams and Bisset, 1998).
The Trichoderma fungus has long been known and it was first documented in 1794 (Persoon, 1794) as a species related to Hypocrea (sexual state). However, it was tough to allocate the genus Trichoderma/Hypocrea morphologically and Trichoderma viride was the only species which was proposed. Species recognition as a specific procedure was first produced in 1969 (Rifai, 1969; Samuels, 2006). As biocontrol agents, Trichoderma spp. has been known for many years for producing antimicrobial agents that inhibit the growth of pathogens (Harman, 2006).
Trichoderma genera of fungi (Division - Ascomycota, Subdivision - Pezizomycotina, Class - Sordariomycetes, Order - Hypocreales, Family - Hypocreaceae) have been known since the 1920s for their capacity to function as biocontrol agents (BCA) against plant pathogens. These agents can be used either to improve the health of crop plants or to boost the natural degradation of toxic compounds in soil and water by some plants. Several species of Trichoderma have been found to interact with crop plants as well as soil borne pathogens (notably Trichoderma harzianum strain T22 and Trichoderma atroviride strain P1) (Woo et al., 2006).
Biocontrol and its mechanisms
Different methods in which Trichoderma acts as a biocontrol agent are: a) Trichoderma competes for nutrients with pathogens by growing faster or using the food source more efficiently than the pathogens, thereby displacing the pathogens (Tyśkiewicz et al., 2022); b) In antibiosis, Trichoderma excretes a substance that slows down or completely retards the growth of pathogens in the vicinity of the compound (Lorito and Woo, 2015); c) Parasitism means that Trichoderma directly feeds on or is a pathogenic species (Lahlali et al., 2022); d) In induced resistance, a plant produces a chemical that protects it from pathogens (Zin and Badaluddin, 2020); and e) Trichoderma can grow in an endophytic manner in other species and plant growth will be enhanced (Tseng et al., 2020) (Figure 1).
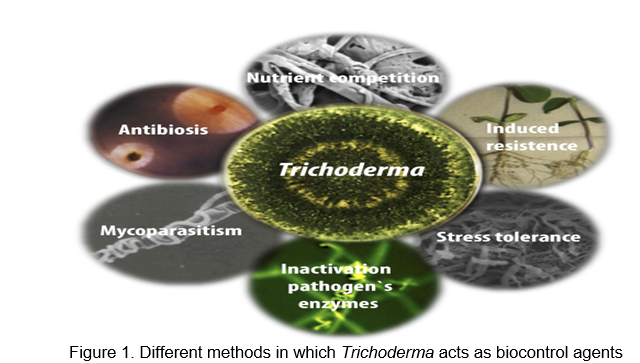
Plant pathogens are known to be controlled by Trichoderma species (Samuels, 1996) since their emergence in the 1920s. It is possible to use them either to enhance the health of crop plants or to increase the capacity for some plants to degrade toxic compounds, both in soil and water. Besides their ability to prevent plant diseases, other Trichoderma genus species are well known for boosting plant growth and development, raised reproductive capability, capacity to alter the rhizosphere, ability to flourish under unfavorable conditions, proficiency in the use of nutrients, strong antagonistic against phytopathogenic fungi and increases defense mechanisms of the plants (Table 1).
In traditional theory, Trichoderma species possess three major mechanisms of action: mycoparasitism (parasitization by another fungus), antibiosis (production of antimicrobial compounds, which inhibit other fungi) and the widespread fact that they compete for food, space, or oxygen (Chet, 1987).
Mycoparasitism
Mycoparasitism is one of the main factors for Trichoderma aggressiveness as a biocontrol agent. In this phenomenon, Trichoderma is chemotropically enhanced, and the host is identified by the mycoparasite, the hyphae are penetrated and the host is lysed (Zeilinger et al., 1999).
Table 1. Biological control of plant diseases by different Trichoderma species
Trichoderma spp.
|
Plant disease
|
Target pathogen
|
Significant findings
|
Trichoderma cellulase
|
Curvularia leaf spot in maize
|
Curvularia lunata
|
It induced plant defenses and provided high levels of systemic resistance by increasing the expression of genes involved in the jasmonate/ethylene signaling pathways (Saravanakumar et al. 2016)
|
Trichoderma asperellum,
T. harzianum
|
Gray mold of tomato
|
Botrytis cinerea
|
The non-volatile organic compound EPl-1 induced plant defense and altered pathogen virulence (Kuzmanovska et al. 2018)
|
T. harzianum Institute of Organic Farming, Dharwad strain (MH027645.1)
|
Soil borne diseases of plants
|
Sclerotium rolfsii, Fusarium oxysporum, Macrophomina phaseolina, Pythium sp., Phytophthora infestans
|
Mycoparasitism through coiling and appressoria formation, secretion of hydrolytic enzymes, penetration of hyphae, and lysis of pathogens.
It also inhibits the growth of plant pathogens by depriving them of nutrient sources and adequate space (Jadav et al., 2022)
|
Trichoderma asperellum
|
Sheath blight of rice
|
Rhizoctonia solani
|
Improved yield and grain weight due to decreased sheath blight progression rate (de França et al., 2015)
|
T. harzianum
|
bulb rot of Tuberose
|
F. oxysporum f. sp. tuberose
|
Enhanced plant health and growth, leading to higher flower production and better quality (Nosir, 2016)
|
T. viride, T. koningii,
T. virens
|
Post harvest diseases of fruits and vegetables
|
Post harvest pathogens
|
Anti-microbial compound (viridin) that inhibiting the spore germination of Penicillium expansum, Botrytis allii, Fusarium caeruleum, Colletotrichum lini, Stachybotrys atra and Aspergillus niger (Dukare et al., 2019)
|
T. pseudokoningii SMF 2
|
Fusarium wilts
|
F. oxysporum
|
Anti-microbial peptide (Trichokonins VI) had antibiotic effects due to its capacity to induce extensive apoptotic programmed cell death (Li et al., 2019)
|
T. harzianum,
T. virens
|
Damping off
|
Pythium sp.
|
A metabolite (gliotoxin) that mimic the plant growth hormone gibberellic acid and involved in faster germination and seedling vigour and makes a pathogen less likely to attack (Uddin et al., 2011)
|
T. spirale T76-1,
|
leaf spot on lettuce
|
Corynespora cassiicola or Curvularia aeria
|
The high activity of β-1,3 glucanase and chitinase inhibited the growth of pathogens (Wonglom et al., 2019)
|
T. virens NBAII Tvs12
|
stem rot in groundnut
|
Sclerotium rolfsii
|
T. harzianum
strain M10
|
Soil borne diseases of tomato
|
Sclerotium rolfsii
|
Harzianic acid is a novel siderophore that aids in seed germination and improved seedling growth (Manganiello et al., 2018)
|
T. harzianum N47
|
Damping off of Pea
|
Pythium ultimum
|
Increasing the number of lateral root and root length makes a pathogen less likely to attack (Naseby et al., 2000)
|
T. atroviride HB20111
|
Fusarium crown rot and sharp eyespot of wheat
|
F. pseudograminearum and Rhizoctonia cerealis
|
Seed dressing may improve the micro-ecological environment for wheat growth by reducing the amount of plant pathogens in the rhizosphere of wheat plants (Sui et al., 2022)
|
T. harzianum IMI-392432
|
Pineapple disease of sugarcane
|
Ceratocystis paradoxa
|
The bioagent has an antagonistic effect on mycelial growth and offers the greatest potential for controlling pineapple disease (Rahman et al., 2009)
|
T. harzianumT22
|
Root-knot nematodes of soybean
|
Meloidogyne incognita
|
Significant inhibition of egg hatching and high virulence against second stage juveniles (J2) with increased plant biomass (Izuogu and Abiri, 2015)
|
T. citrinoviride
|
Root-knot nematodes of tomato
|
Meloidogyne incognita
|
T. hamatum strain Th23
|
Tobacco mosaic disease of tomato
|
Tobacco mosaic virus
|
Plant growth was accelerated, and systemic resistance and innate immunity were boosted (Abdelkhalek et al., 2022)
|
T. asperellum (T-203)
|
Angular leaf spot of cucumber
|
Pseudomonas syringae pv. lachrymans
|
Plant signaling and biosynthesis are activated in cucumber through separate metabolic pathways, eventually leading to an accumulation of phytoalexins in the plant body (Harman et al., 2004)
|
The role of identification in Trichoderma - fungal interactions was illustrated in the demonstration where nylon fibers were smeared with lectins, and the smeared lectins were coiled all over by Trichoderma harzianum, but not the fibers that had not been smeared (Inbar and Chet, 1992). This demonstration emphasized the achievable function of signal interplay in this new host-parasite interaction. The mainly chitinases, glucanases and proteases are the hydrolytic enzymes which are responsible for the lysis of the host which relate to the mycoparasitism (Chet et al., 1998).
Reithner et al. (2011) reported that Trichoderma atroviride is having mycoparasitism-associated genes. The transcriptome of Trichoderma atroviride IMI206040 was examined when it entered mycoparasitic interaction with Rhizoctonia solani, a plant pathogen. In this analysis, Trichoderma genes were sequenced which showed 7797 transcript fragments, 175 of which were host approachable. When eukaryotic orthologous groups (KOGs) were used to determine the functions of these genes, the most abundant category was "metabolism." Quantitative reverse transcription (qRT)-PCR analysis confirmed differential transcription of 13 genes (swo1, which codes for an expansion-like protein; axe1, which codes for an acetyl xylan esterase; and homologous to papA and pra1 encoding aspartyl proteases) in the existence of R. solani. An extra parallel gene expression examination of these genes, carried out at different levels of mycoparasitism in opposition to Botrytis cinerea and Phytophthora capsici, disclosed a synergistic transcription of different genes involved in cell wall degradation. During the mycoparasitism of T. atroviride, these genes may be regulated analogically due to their similar expression patterns and occurrence of regulatory binding sites in the promoter regions. Additionally, the induction of pra1 was shown to be chitin- and distance-dependent.
A Trichoderma strain parasitizes a Pythium pathogen on the surface of a pea seed (Figure 2). A fluorescent orange dye was applied to the Trichoderma strain and a green dye to the Pythium.
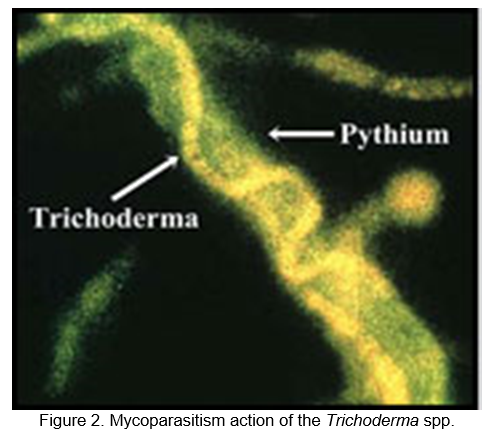
Competition
The most familiar motive for the death of many microorganisms flouring in the ambit of Trichoderma strains is the starvation and insufficient of nutrients (Figure 3). It is feasible to use this method in biological control of fungal phytopathogens. The vitality of most filamentous fungi depends on two components: carbon and iron. Besides Trichoderma, many other fungi, such as some strains of F. oxysporum, utilize competition for carbon as a mode of action (Sarrocco, et al., 2009). To mobilize iron from the surrounding environment when there is an iron crisis, most fungi secrete ferric-iron specific chelators. Additionally, T. harzianum T35 engages in rhizosphere colonization and nutrient competition with Fusarium oxysporum (Tjamos et al., 1922). Trichoderma strain filtrates were recently used to combat pineapple disease of sugarcane caused by Ceratocystis paradoxa (Rahman et al., 2009).
Biological control can be accomplished through divergent procedure, fungistasis and nutrient competition. Typically, fungistasis hinders the development of the fungus and its reproduction, but it does not kill it. Certain Trichoderma strains can kill phytopathogens such as Rhizoctonia solani (Gajera et al., 2013). Utilizing the nutrients in the soil is a method of restricting the pathogens' access to them by means of aggression for nutrients. Due to the ability of Trichoderma to mature quickly, they use nutrients more efficiently than pathogens, resulting in famine for the pathogen, thereby causing the phytopathogen to die (Gajera et al., 2013; Mukherjee et al., 2012).
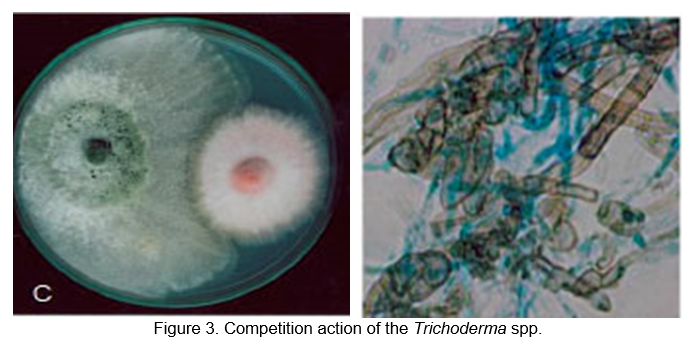
Antibiosis
The operation of antibiosis regularly appeared among numerous species consisting of microorganisms and plants. The growth of other microbes is hindered by antibiotics or the small size diffusible elements of Trichoderma (Benitez et al., 2004).
In the soil and root zone, Trichoderma species are unbound-living fungi that control a wide range of pathogens and diseases (Sharon et al., 2011). Several strains develop root colonization, and many of these strains are highly productive, resistant to abiotic stresses, and can take up and use nutrients. Trichoderma species can compete and command a large diversity of economically predominant plant pathogenic fungi, viruses, bacteria and nematodes (Figure 4). Among the huge obstacles to crops production are root-knot nematodes, including Meloidogyne javanica and Meloidogyne incognita, which are sedentary, obligatory root endoparasites of many economically significant plants. Hence, Trichoderma has been focused on its biocontrol efforts on these nematodes. Many Trichoderma species and isolates have been tested as biocontrol vehicles in opposition to the nematodes with many different crops and experimental circumstances. Remarkable answers to nematode control and plants growth were attained (Guzman-Guzman et al., 2023). Focusing to upgrade the biocontrol procedure, methods of operation of the fungus in opposition to the root-knot nematodes have been explored and are reported in this chapter. A variety of processes was investigated, including parasitism, enzymatic lysis, antibiosis, and induced resistance. Acknowledge the fungus-nematode-plant interactions and the operation of the biocontrol procedure might bestow to enhance the accomplishment of this biocontrol agent.
It was revealed by Reino et al. (2008) that the Trichoderma species are unbound-living fungi that are extremely interactive in root, soil, and foliar environments and have been successful in protecting large crops from pathogens in field trials. The structure and biology of the metabolites extracted from Trichoderma species have been reviewed. Furthermore, this comprehensive assessment, including all the literature on the subject up to the present day, in which 269 references are cited, summarizes the literature on the biological activity of the metabolites, particularly when used in biological control techniques (Jose et al., 2008).
Mukherjee, (2011) reported that entire genome sequencing of Gliocladium virens and T. atroviride, these two are the most effective antagonists of plant pathogenic fungi. Principal appreciation into the genetics of mycoparasitism and biological control are also discussed. Mukherjee et al., (2012) reported that antibiosis procedure comprises the making or delivery of compounds (antibiotics or hydrolytic enzymes) that will kill or hinder the maturity of pathogens. There has been a growing interest in Trichoderma strains for their production of volatile and non-volatile toxic metabolites, which are antagonistic to pathogens.
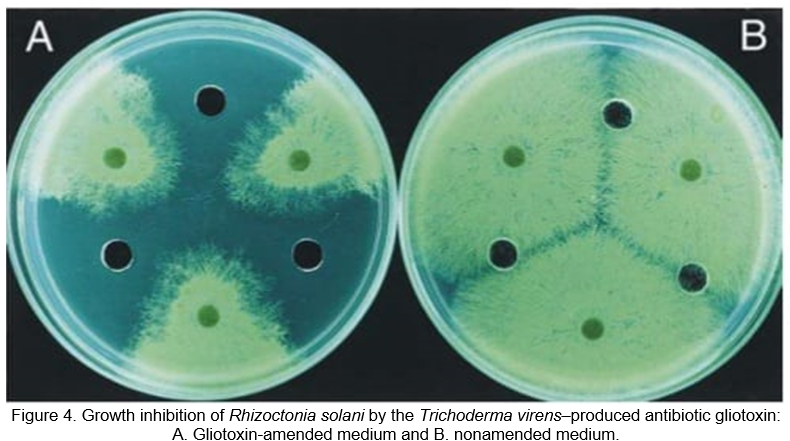
Induced systemic resistance
The great priority of Trichoderma investigation was to realize the direct consequence on other fungal species, especially mycoparasitism and antibiosis. According to Bigirimana et al. (1997) the first clear illustration of induced resistance of bean plants to fungi revealed that only a root application of T-39 enabled bean plants to withstand fungal foliar diseases caused by B. cinerea and C. lindemuthianum. Researchers found that over ten different dicots and monocots appear to be protected by the induced resistance from infections caused by fungi (B. cinerea, R. solani, Colletotrichum spp., Phytophthora spp., Alternaria spp., Magnaporthe grisea, etc.), bacteria (Xanthomonas spp., Pseudomonas syringae, etc.) and even viruses like CMV. B. cinerea and C. lindemuthianum were also effectively controlled in bean plants after soil was treated with T. harzianum strain T-39. Other dicots also exhibited similar behavior as B. cinerea (De Mayer et al., 1998).
According to Djonovic et al. (2007), induced systemic resistance in maize is regulated by proteinaceous elicitor Sm1, released by the beneficial fungus Trichoderma virens. Researchers previously demonstrated that the filamentous fungus Trichoderma virens induces resistance to systemic diseases in cotton (Gossypium hirsutum) by producing a highly effective hydrophobin-like elicitor called Sm1 (small proteins named after the scientist Stephanie Smith). A functional genomics approach was used to study the role of Sm1 during maize-fungal interactions by colonizing roots of T. virens and investigating whether systemic protection against a foliar pathogen is induced. A hydroponic system or a soil-grown maize seedling exposed to the pathogen Coletotrichum graminicola was used to immunize seedlings with T. virens Gv29-8 (wild type) and transformants that disrupted Sm1 or overexpressed it. In a similar manner to dicot plants, we demonstrated that T. virens colonization of maize roots induces systemic coverage of C. graminicola-immunized leaves. Jasmonic acid and green leaves volatile biosynthesis genes were important in this defense (Wang et al., 2020). T. virens did not undergo normal growth or development when Sm1 was removed or overexpressed, nor did the normal production of gliotoxin, hyphal coiling, or hydrophobicity, or their ability to colonize maize roots. The results of plant bioassays showed that plants grown with Sm1-elimination strains were just as protected as non-Trichoderma-treated plants. A functional decrease in disease protection was observed when Sm1 was eliminated, as well as an increase in disease protection when it was overexpressed. Based on these results, it appears that the functional Sm1 elicitor is necessary for active activation of systemic disease protection for T. virens (Figure 5).
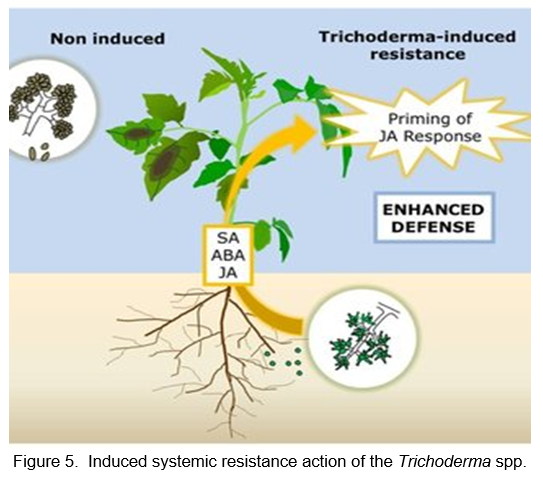
Secondary metabolism
There are four major chemical classes of fungal SMs: polyketides, terpenoids, compounds derived from shikimic acid, and non-ribosomal peptides (Pusztahelyi et al., 2015). Aside from hybrid metabolites which comprise molecules from different classes, meroterpenoids are examples of fusions between terpenes and polyketides. It was found that ascomycetes had higher numbers of secondary metabolism genes compared to basidiomycetes, archeo-ascomycetes, and chytridiomycetes, whereas hemi-ascomycetes and zygomycetes have none (Collemare et al., 2008). Polyketide synthases (PKS) code for 16 number of the genome of an ascomycete, compared to 10 number for non-ribosomal protein synthases (NRPS), two for tryptophan synthases (TS), and two for dimethylallyl tryptophan synthases (DMATS), which are crucial for SM synthesis. Enzymes encoding these types of SM genes are found in gene clusters associated with secondary metabolism and are crucial for the main synthesis of metabolites. Ascomycetes have only been found to contain PKS-NRPSs, with an average of three genes per species. Human pathogens Coccidioides spp. and Histoplasma capsulatum as well as Neurospora crassa have fewer PKSs (1–9 genes), NRPS (3–6 genes), and PKS-NRPSs (0–2 genes) than other ascomycetes. It has been reported that many fungi contain more than 40 genes encoding PKS, NRPS, hybrids, TS, and DMATS in their genomes, including M. grisea (45 genes) (Collemare et al., 2008). Siderophores, which are SMs that possess iron uptake capability, are also synthesized by the NRPS, which is also essential for the virulence of several fungi (e.g., Cochliobolus heterostrophus, C. miyabeanus, F. graminearum, and A. brassicicola) (Oide et al., 2006).
There is direct as well as indirect evidence indicating that secondary metabolites (natural products) play a role in Trichoderma's antagonistic activity against several bacteria, yeasts, and fungi as well as in its beneficial effects on crops. Though not clinically used, some of the secondary metabolites produced by Trichoderma have potential as drugs and one compound (6-pentyl-α-pyrone) is used as a food flavoring agent. Trichoderma began to be studied in the 1930s with the discovery of gliotoxin among a plethora of substances (Weindling and Emerson, 1936). In 2014, Hermosa et al. (2014) estimated that about 1,000 compounds are produced by fungi of this genus (Figure 6).
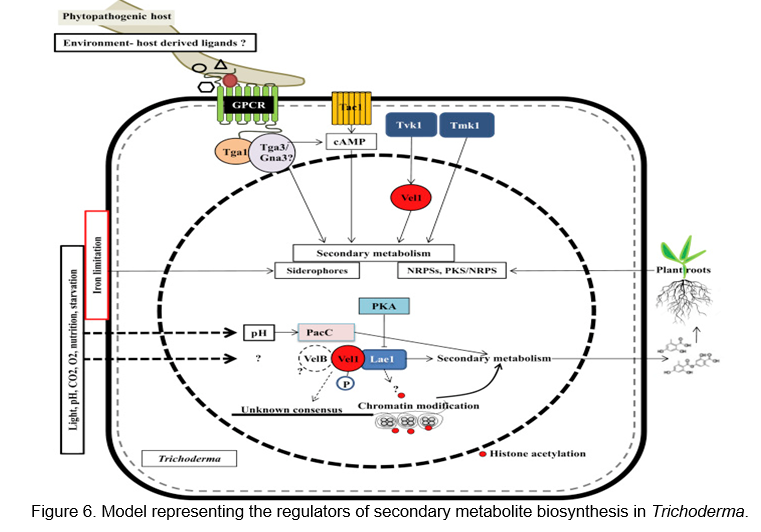
CONCLUSION
The success of Trichoderma strains as biocontrol agents against different pathogenic microorganisms is well known worldwide. Trichoderma spp. have been widely used in agricultural applications due to their well-known biological control mechanism. Trichoderma has many useful mechanisms that can possibly be utilized in introducing the best microbe to the plants. The mechanisms comprise of (I) parasitism, (II) producing the secondary metabolites, antibiosis, and enzymes, (III) inducing the defense and resistance of plant, and (IV) enhancing the plant growth. These enable the capabilities of Trichoderma to control the plant pathogens in the soil, seeds, roots, stem, leaf, fruits, postharvest, insect and weeds. Thus, Trichoderma spp. can be possibly explored both as biopesticides and biofertilizers. Trichoderma spp., when combined in a product, are able to control different crop diseases, stimulate plant growth and development, improve the composting process, and promise a clean environment towards achieving sustainable agriculture.
REFERENCES
Abdelkhalek, A., Al-A. Abdulaziz, A. Amr and B. Said. 2022. Trichoderma hamatum strain Th23 promotes tomato growth and induces systemic resistance against Tobacco mosaic virus. J. Fungi. 8: 228.
Benitez, T., A. M. Rincon, M. C. Limn and A. C. Codon. 2004. Biocontrol mechanisms of Trichoderma strains. Int. J. Microbiol. 7(4): 249–260.
Chet, I., 1987. Trichoderma- application, mode of action and potential as a biocontrol agent of soil-borne plant pathogenic fungi. In: Innovative approaches to plant disease control (Ed. Chet, I.), Wiley-Interscience, New York.
Chet, I., N. Benhamou and S. Haran. 1998. Mycoparasitism and lytic enzymes. In: Trichoderma and Gliocladium (Vol. 2). Enzymes, biological control and commercial applications (Eds. Harman, G. E. and C. P. Kubicek), Taylor and Francis, London, 153–171.
Collemare, J., M. Pianfetti, A. E. Houlle, D. Morin, L. Camborde, M. J. Gagey, C. Barbisan, I. Fudal, M. H. Lebrun and H. U. Bohnert. 2008. Magnaporthe grisea avirulence gene ACE1 belongs to aninfection-specific gene cluster involved in secondary metabolism. New Phytologist. 179(1): 196–208.
de França, S. K. S., A. F. Cardoso, D. C. Lustosa, E. M. L. S. Ramos, M. C. C. de Filippi and G. B. da Silva. 2015. Biocontrol of sheath blight by Trichoderma asperellum in tropical lowland rice. Agron. Sustain. Dev. 35: 317–324.
Djonovic, S., W. A. Vargas, M. V. Kolomiets, M. Horndeski, A. Wiest and C. M. Kenerley. 2007. A proteinaceous elicitor Sm1 from the beneficial fungus Trichoderma virens is required for induced systemic resistance in maize. Plant Physiol. 145(3): 875–889.
Dukare, A. S., S. Paul, V. E. Nambi, R. K. Gupta, R. Singh, K. Sharma and R. K. Vishwakarma. 2019. Exploitation of microbial antagonists for the control of postharvest diseases of fruits: A review. Crit. Rev. Food Sci. Nutr. 59(9): 1498–1513.
Gajera, H., R. Domadiya, S. Patel, M. Kapopara and B. Golakiya. 2013. Molecular mechanism of Trichoderma as bio-control agents against phytopathogen system – a review. Curr. Res. Microbiol. Biotechnol. 1(4): 133–142.
Gams, W. and J. Bisset. 1998. Morphology and identification of Trichoderma. In: Trichoderma and Gliocladium ( Vol. 1). Basic biology, taxonomy and genetics (Eds. Harman, G.E. and C. P. Kubicek), Taylor and Francis, London, 3–31.
Guzman-Guzman, P., A. Kumar, S. Santos-Villalobos, F. I. Parra-Cota, D. C. Ma. Orozco-Mosqueda, A. E. Fadiji, S. Hyder, O. O. Babalola and G. Santoyo. 2023. Trichoderma Species: Our best fungal allies in the biocontrol of plant diseases-A review. Plants. 12(3): 432. https://doi.org/10.3390/plants12030432.
Harman, G. E., 2006. Overview of mechanisms and uses of Trichoderma spp. Phytopathol. 96(2): 190–194.
Harman, G., C. R. Howell, A. Viterbo, I. Chet, and M. Lorito. 2004. Trichoderma species - opportunistic, avirulent plant symbionts. Nature Reviews Microbiol. 2: 43–56.
Hermosa, R., R. E. Cardoza, M. B. Rubio, S. Gutiérrez and E. Monte. 2014. Secondary metabolism and antimicrobial metabolites of Trichoderma. Biotechnol. Biol. Trichoderma. 125–137.
Inbar, J. and I. Chet. 1992. Biomimics of fungal cell-cell recognition by use of lectin-coated nylon fibres. J. Bacteriol. 174(3): 1055–1059.
Izuogu, N. B. and T. O. Abiri. 2015. Efficacy of Trichoderma harzianum T22 as a biocontrol agent against root-knot nematode (Meloidogyne incognita) on some soybean varieties. Croatian J. Food Sci. Technol. 7: 47–51.
Jadav, P. C., G. M. Hegde, S. Jahagirdar, and V. Navi. 2022 Evaluation of microbial consortia against Sclerotium rolfsii Sacc. causing foot rot of wheat. J. Farm Sci. 35(2): 219–222.
Jose, L. R., R. F. Guerrero, R. Hernández-Galán and I. G. Collado. 2008. Secondary metabolites from species of the biocontrol agent Trichoderma. Phytochemistry Reviews. 7: 89-123.
Kuzmanovska, B., R. Rusevski, M. Jankulovska and K. B. Oreshkovikj. 2018. Antagonistic activity of Trichoderma asperellum and Trichoderma harzianum against genetically diverse Botrytis cinerea isolates. Chilean J. Agric. Res. 78(3): 391–399.
Lahlali, R., S. Ezrari, N. Radouane, J. Kenfaoui, Q. Esmaeel, H. El Hamss, Z. Belabess and E. A. Barka. 2022. Biological control of plant pathogens: A global perspective. Microorganisms. 10(3): 596.
Li, M., M. A. Guang-shu, L. I. A. N. Hua, S. U. Xiao-lin, T. I. A. N. Ying, H. U. A. N. G. Wen-kun, M. E. I. Jie and J. I. A. N. G. Xi-liang. 2019. The effects of Trichoderma on preventing cucumber Fusarium wilt and regulating cucumber physiology. J. Integr. Agric. 18(3): 607–617.
Lorito, M. and S. Woo. 2015. Trichoderma: A multi-purpose tool for integrated pest management. In: Lugtenberg, B. (eds) Principles of Plant-Microbe Interactions. Springer, Cham. https://doi.org/10.1007/978-3-319-08575-3_36.
Manganiello, G., A. Sacco, M. R. Ercolano, F. Vinale, S. Lanzuise, A. Pascale, M. Napolitano, N. Lombardi, M. Lorito and S. L. Woo. 2018. Modulation of tomato response to Rhizoctonia solani by Trichoderma harzianum and its secondary metabolite harzianic acid. Front. Microbiol. 9: 1966. doi: 10.3389/fmicb.2018.01966.
Mukherjee, M., P. K. Mukherjee, B. A. Horwitz, C. Zachow, G. Berg and S. Zeilinger. 2012. Trichoderma-plant-pathogen interactions: Advances in genetics of biological control. Indian J. Microbiol. 52(4): 522–529.
Naseby, D. C., J. A. Pascual and J. M. Lynch. 2000. Effect of biocontrol strains of Trichoderma on plant growth, Pythium ultimum populations, soil microbial communities and soil enzyme activities. J. Appl. Microbiol. 88(1): 161–169.
Nosir, W. S. 2016. Trichoderma harzianum as a growth promoter and bio-control agent against Fusarium oxysporum f. sp. tuberosi. Adv. Crop Sci. Tech. 4: 217. doi:10.4172/2329-8863.1000217.
Oide, S., S. B. Krasnoff, D. M. Gibson and B. G. Turgeon. 2006. Intracellular siderophores are essential for ascomycete sexual development in heterothallic Cochliobolus heterostrophus and homothallic Gibberella zeae. Eukaryotic Cell. 6(8): 1339–1353.
Persoon, C.H., 1794. Neuer Versuch einer systematischen Einteilunder Schwamme. Racodium Romer's Neues Magazin der Botanik. 1: 123.
Pusztahelyi, T., I. J. Holb and I. Pócsi. 2015. Secondary metabolites in fungus-plant interactions. Front. Plant Sci. 6: 573. doi: 10.3389/fpls.2015.00573.
Rahman, M.A., M. F. Begum and M. F. Alam. 2009. Screening of Trichoderma isolates as a biological control agent against Ceratocystis paradoxa causing pineapple disease of sugarcane. Mycobiol. 37(4): 277–285.
Rahman, M.A., M. F. Begum, M. F. Alam. 2009. Screening of Trichoderma isolates as a biological control agent against Ceratocystis paradoxa causing pineapple disease of sugarcane. Mycobiology. 37(4): 277–285.
Reino, J. L., R. F. Guerrero, R. Hernandez-Galan and I. G. Collado. 2008. Secondary metabolites from species of the biocontrol agent Trichoderma. Phytochemistry Reviews. 7(1): 89–123.
Reithner, B., E. Ibarra-Laclette, R. L. Mach and A. Herrera-Estrella. 2011. Identification of mycoparasitism-related genes in Trichoderma atroviride. App. Environ. Microbiol. 77(13): 4361–4370.
Rifai, M. A., 1969. A revision of the genus Trichoderma. Mycological Papers. 116: 1–56.
Samuel, G. J., 1996. Trichoderma: A review of biology and systematic of the genus. Mycol. Res. 100: 923-935.
Samuels, G. J., 2006. Trichoderma: Systematic, the Sexual State and Ecology. Phytopathology. 96(2): 195–206.
Saravanakumar, K., L. Fan, K. Fu. et al. 2016. Cellulase from Trichoderma harzianum interacts with roots and triggers induced systemic resistance to foliar disease in maize. Sci. Rep. 6: 35543. https://doi.org/10.1038/srep35543.
Sarrocco, S., L. Guidi, S. Fambrini, E. DelInnocenti and G. Vannacci. 2009. Competition for cellulose exploitation between Rhizoctonia solani and two Trichoderma isolates in the decomposition of wheat straw. J. Plant Pathol. 91(2): 331–338.
Sharon, E., I. Chet and Y. Spiegel. 2011. Trichoderma as a biological control agent. Biological Control of Plant-Parasitic Nematodes. 183–201.
Singh, U. S., N. W. Zaidi, D. Joshi, S. Varshney and T. Khan. 2006. Current status of Trichoderma as a biocontrol agent. In: Current status of biological control of plant diseases using antagonistic organisms in India (Eds. Ramanujam, B. and R. J. Rabindra), Project Directorate of Biological Control, Bangalore.
Sui, L., J. Li, J. Philp, K. Yang, Y. Wei, H. Li, J. Li, L. Li, M. Ryder, R. Toh, Y. Zhou, M. D. Denton, J. Hu and Y. Wang. 2022. Trichoderma atroviride seed dressing influenced the fungal community and pathogenic fungi in the wheat rhizosphere. Sci. Rep. 12(1): 9677.
Tjamos, E. C., G. C. Papavizas, and R. J. Cook. 1922. Biological control of plant diseases. Progress and challenges for the future. Plenum Press, New York, 222.
Tseng, Y-H., H. Rouina, K. Groten, P. Rajani, A. C. U. Furch, M. Reichelt, I. T, Baldwin, K. N. Nataraja, R. Uma Shaanker and R. Oelmüller. 2020. An endophytic Trichoderma strain promotes growth of its hosts and defends against pathogen attack. Front. Plant Sci. 11: 573670.
Tyśkiewicz, R., A. Nowak, E. Ozimek and J. Jaroszuk-Ściseł. 2022. Trichoderma: The current status of its application in agriculture for the biocontrol of fungal phytopathogens and stimulation of plant growth. Int, J, Mol, Sci. 23(4): 2329.
Uddin, M. M., N. Akhtar, M. T. Islamand and A. N. Faruq. 2011. Effect of Trichoderma harzianum and some selected soil amendment against damping off disease complex of potato and chilli. The Agriculturists. 9(1&2): 106–116.
Wang, K. D., Z. Gorman, P. C. Huang, C. M. Kenerley and M. V. Kolomiets. 2020. Trichoderma virens colonization of maize roots triggers rapid accumulation of 12-oxophytodienoate and two ⍺-ketols in leaves as priming agents of induced systemic resistance. Plant Signal. Behav. 15(9): 1792187. doi: 10.1080/15592324.2020.1792187.
Weindling and Emerson. 1936. The isolation of a toxic substance from the culture filtrate of Trichoderma. Phytopathology. 26(3): 1068–1070.
Wonglom, P., W. Daengsuwan, S-i. Ito and A. Sunpapao. 2019. Biological control of Sclerotium fruit rot of snake fruit and stem rot of lettuce by Trichoderma sp. T76-12/2 and the mechanisms involved. Physiol. Mol. Plant Pathol. 107: 1–7.
Woo, S. L., F. Scala, M. Ruocco and M. Lorito. 2006. The molecular biology of the interactions between Trichoderma spp., pathogenic fungi and plants. Phytopathology. 96: 181–185.
Zeilinger, S., C. Galhaup, K. Payer, S. L. Woo, R. L. Mach, C. Fekete, M. Lorito and C. P. Kubicek. 1999. Chitinase gene expression during mycoparasitic interaction of Trichoderma harzianum with its host. Fungal Genetics and Biology. 26(2): 131–140.
Zin, N. A. and N. A. Badaluddin. 2020. Biological functions of Trichoderma spp. for agriculture applications, Ann. Agric. Sci. 65(2): 168–178.
Mechanisms of Resistance of Trichoderma spp. against Plant Disease Management
DOI: https://doi.org/10.56669/QXDL7420
ABSTRACT
Plant pathogens have been known to be controlled by Trichoderma species for decades. It is possible to use them either to enhance the health of crop plants or to increase the capacity for some plants to degrade toxic compounds, both in soil and water. Trichoderma species are well known for boosting plant growth and development, raised reproductive capability, capacity to alter the rhizosphere, ability to flourish under unfavorable conditions, proficiency in the use of nutrients, strong antagonistic against phytopathogenic fungi and increases defense mechanisms of the plants. Trichoderma strains can kill phytopathogens such as R solani. Utilizing the nutrients in the soil is a method of restricting the pathogens' access to them by means of aggression for nutrients. Trichoderma spp. are known to hinder the growth of other microbes by production of antibiotics and secondary metabolites. There is direct as well as indirect evidence indicating that secondary metabolites play a role in antagonistic activity of the Trichoderma spp. against various plant pathogens.
Keywords: biocontrol, Trichoderma, mechanisms, plant disease management
INTRODUCTION
Conventionally, Genus Trichoderma has been classified as Fungi Imperfecti. They give rise to only asexual spores i.e., conidia (Singh et al., 2006). With the recent advances in molecular taxonomy, many of the species have been reclassified as belonging to the genus Hypocrea of the class ascomycetes (Gams and Bisset, 1998).
The Trichoderma fungus has long been known and it was first documented in 1794 (Persoon, 1794) as a species related to Hypocrea (sexual state). However, it was tough to allocate the genus Trichoderma/Hypocrea morphologically and Trichoderma viride was the only species which was proposed. Species recognition as a specific procedure was first produced in 1969 (Rifai, 1969; Samuels, 2006). As biocontrol agents, Trichoderma spp. has been known for many years for producing antimicrobial agents that inhibit the growth of pathogens (Harman, 2006).
Trichoderma genera of fungi (Division - Ascomycota, Subdivision - Pezizomycotina, Class - Sordariomycetes, Order - Hypocreales, Family - Hypocreaceae) have been known since the 1920s for their capacity to function as biocontrol agents (BCA) against plant pathogens. These agents can be used either to improve the health of crop plants or to boost the natural degradation of toxic compounds in soil and water by some plants. Several species of Trichoderma have been found to interact with crop plants as well as soil borne pathogens (notably Trichoderma harzianum strain T22 and Trichoderma atroviride strain P1) (Woo et al., 2006).
Biocontrol and its mechanisms
Different methods in which Trichoderma acts as a biocontrol agent are: a) Trichoderma competes for nutrients with pathogens by growing faster or using the food source more efficiently than the pathogens, thereby displacing the pathogens (Tyśkiewicz et al., 2022); b) In antibiosis, Trichoderma excretes a substance that slows down or completely retards the growth of pathogens in the vicinity of the compound (Lorito and Woo, 2015); c) Parasitism means that Trichoderma directly feeds on or is a pathogenic species (Lahlali et al., 2022); d) In induced resistance, a plant produces a chemical that protects it from pathogens (Zin and Badaluddin, 2020); and e) Trichoderma can grow in an endophytic manner in other species and plant growth will be enhanced (Tseng et al., 2020) (Figure 1).
Plant pathogens are known to be controlled by Trichoderma species (Samuels, 1996) since their emergence in the 1920s. It is possible to use them either to enhance the health of crop plants or to increase the capacity for some plants to degrade toxic compounds, both in soil and water. Besides their ability to prevent plant diseases, other Trichoderma genus species are well known for boosting plant growth and development, raised reproductive capability, capacity to alter the rhizosphere, ability to flourish under unfavorable conditions, proficiency in the use of nutrients, strong antagonistic against phytopathogenic fungi and increases defense mechanisms of the plants (Table 1).
In traditional theory, Trichoderma species possess three major mechanisms of action: mycoparasitism (parasitization by another fungus), antibiosis (production of antimicrobial compounds, which inhibit other fungi) and the widespread fact that they compete for food, space, or oxygen (Chet, 1987).
Mycoparasitism
Mycoparasitism is one of the main factors for Trichoderma aggressiveness as a biocontrol agent. In this phenomenon, Trichoderma is chemotropically enhanced, and the host is identified by the mycoparasite, the hyphae are penetrated and the host is lysed (Zeilinger et al., 1999).
Table 1. Biological control of plant diseases by different Trichoderma species
Trichoderma spp.
Plant disease
Target pathogen
Significant findings
Trichoderma cellulase
Curvularia leaf spot in maize
Curvularia lunata
It induced plant defenses and provided high levels of systemic resistance by increasing the expression of genes involved in the jasmonate/ethylene signaling pathways (Saravanakumar et al. 2016)
Trichoderma asperellum,
T. harzianum
Gray mold of tomato
Botrytis cinerea
The non-volatile organic compound EPl-1 induced plant defense and altered pathogen virulence (Kuzmanovska et al. 2018)
T. harzianum Institute of Organic Farming, Dharwad strain (MH027645.1)
Soil borne diseases of plants
Sclerotium rolfsii, Fusarium oxysporum, Macrophomina phaseolina, Pythium sp., Phytophthora infestans
Mycoparasitism through coiling and appressoria formation, secretion of hydrolytic enzymes, penetration of hyphae, and lysis of pathogens.
It also inhibits the growth of plant pathogens by depriving them of nutrient sources and adequate space (Jadav et al., 2022)
Trichoderma asperellum
Sheath blight of rice
Rhizoctonia solani
Improved yield and grain weight due to decreased sheath blight progression rate (de França et al., 2015)
T. harzianum
bulb rot of Tuberose
F. oxysporum f. sp. tuberose
Enhanced plant health and growth, leading to higher flower production and better quality (Nosir, 2016)
T. viride, T. koningii,
T. virens
Post harvest diseases of fruits and vegetables
Post harvest pathogens
Anti-microbial compound (viridin) that inhibiting the spore germination of Penicillium expansum, Botrytis allii, Fusarium caeruleum, Colletotrichum lini, Stachybotrys atra and Aspergillus niger (Dukare et al., 2019)
T. pseudokoningii SMF 2
Fusarium wilts
F. oxysporum
Anti-microbial peptide (Trichokonins VI) had antibiotic effects due to its capacity to induce extensive apoptotic programmed cell death (Li et al., 2019)
T. harzianum,
T. virens
Damping off
Pythium sp.
A metabolite (gliotoxin) that mimic the plant growth hormone gibberellic acid and involved in faster germination and seedling vigour and makes a pathogen less likely to attack (Uddin et al., 2011)
T. spirale T76-1,
leaf spot on lettuce
Corynespora cassiicola or Curvularia aeria
The high activity of β-1,3 glucanase and chitinase inhibited the growth of pathogens (Wonglom et al., 2019)
T. virens NBAII Tvs12
stem rot in groundnut
Sclerotium rolfsii
T. harzianum
strain M10
Soil borne diseases of tomato
Sclerotium rolfsii
Harzianic acid is a novel siderophore that aids in seed germination and improved seedling growth (Manganiello et al., 2018)
T. harzianum N47
Damping off of Pea
Pythium ultimum
Increasing the number of lateral root and root length makes a pathogen less likely to attack (Naseby et al., 2000)
T. atroviride HB20111
Fusarium crown rot and sharp eyespot of wheat
F. pseudograminearum and Rhizoctonia cerealis
Seed dressing may improve the micro-ecological environment for wheat growth by reducing the amount of plant pathogens in the rhizosphere of wheat plants (Sui et al., 2022)
T. harzianum IMI-392432
Pineapple disease of sugarcane
Ceratocystis paradoxa
The bioagent has an antagonistic effect on mycelial growth and offers the greatest potential for controlling pineapple disease (Rahman et al., 2009)
T. harzianumT22
Root-knot nematodes of soybean
Meloidogyne incognita
Significant inhibition of egg hatching and high virulence against second stage juveniles (J2) with increased plant biomass (Izuogu and Abiri, 2015)
T. citrinoviride
Root-knot nematodes of tomato
Meloidogyne incognita
T. hamatum strain Th23
Tobacco mosaic disease of tomato
Tobacco mosaic virus
Plant growth was accelerated, and systemic resistance and innate immunity were boosted (Abdelkhalek et al., 2022)
T. asperellum (T-203)
Angular leaf spot of cucumber
Pseudomonas syringae pv. lachrymans
Plant signaling and biosynthesis are activated in cucumber through separate metabolic pathways, eventually leading to an accumulation of phytoalexins in the plant body (Harman et al., 2004)
The role of identification in Trichoderma - fungal interactions was illustrated in the demonstration where nylon fibers were smeared with lectins, and the smeared lectins were coiled all over by Trichoderma harzianum, but not the fibers that had not been smeared (Inbar and Chet, 1992). This demonstration emphasized the achievable function of signal interplay in this new host-parasite interaction. The mainly chitinases, glucanases and proteases are the hydrolytic enzymes which are responsible for the lysis of the host which relate to the mycoparasitism (Chet et al., 1998).
Reithner et al. (2011) reported that Trichoderma atroviride is having mycoparasitism-associated genes. The transcriptome of Trichoderma atroviride IMI206040 was examined when it entered mycoparasitic interaction with Rhizoctonia solani, a plant pathogen. In this analysis, Trichoderma genes were sequenced which showed 7797 transcript fragments, 175 of which were host approachable. When eukaryotic orthologous groups (KOGs) were used to determine the functions of these genes, the most abundant category was "metabolism." Quantitative reverse transcription (qRT)-PCR analysis confirmed differential transcription of 13 genes (swo1, which codes for an expansion-like protein; axe1, which codes for an acetyl xylan esterase; and homologous to papA and pra1 encoding aspartyl proteases) in the existence of R. solani. An extra parallel gene expression examination of these genes, carried out at different levels of mycoparasitism in opposition to Botrytis cinerea and Phytophthora capsici, disclosed a synergistic transcription of different genes involved in cell wall degradation. During the mycoparasitism of T. atroviride, these genes may be regulated analogically due to their similar expression patterns and occurrence of regulatory binding sites in the promoter regions. Additionally, the induction of pra1 was shown to be chitin- and distance-dependent.
A Trichoderma strain parasitizes a Pythium pathogen on the surface of a pea seed (Figure 2). A fluorescent orange dye was applied to the Trichoderma strain and a green dye to the Pythium.
Competition
The most familiar motive for the death of many microorganisms flouring in the ambit of Trichoderma strains is the starvation and insufficient of nutrients (Figure 3). It is feasible to use this method in biological control of fungal phytopathogens. The vitality of most filamentous fungi depends on two components: carbon and iron. Besides Trichoderma, many other fungi, such as some strains of F. oxysporum, utilize competition for carbon as a mode of action (Sarrocco, et al., 2009). To mobilize iron from the surrounding environment when there is an iron crisis, most fungi secrete ferric-iron specific chelators. Additionally, T. harzianum T35 engages in rhizosphere colonization and nutrient competition with Fusarium oxysporum (Tjamos et al., 1922). Trichoderma strain filtrates were recently used to combat pineapple disease of sugarcane caused by Ceratocystis paradoxa (Rahman et al., 2009).
Biological control can be accomplished through divergent procedure, fungistasis and nutrient competition. Typically, fungistasis hinders the development of the fungus and its reproduction, but it does not kill it. Certain Trichoderma strains can kill phytopathogens such as Rhizoctonia solani (Gajera et al., 2013). Utilizing the nutrients in the soil is a method of restricting the pathogens' access to them by means of aggression for nutrients. Due to the ability of Trichoderma to mature quickly, they use nutrients more efficiently than pathogens, resulting in famine for the pathogen, thereby causing the phytopathogen to die (Gajera et al., 2013; Mukherjee et al., 2012).
Antibiosis
The operation of antibiosis regularly appeared among numerous species consisting of microorganisms and plants. The growth of other microbes is hindered by antibiotics or the small size diffusible elements of Trichoderma (Benitez et al., 2004).
In the soil and root zone, Trichoderma species are unbound-living fungi that control a wide range of pathogens and diseases (Sharon et al., 2011). Several strains develop root colonization, and many of these strains are highly productive, resistant to abiotic stresses, and can take up and use nutrients. Trichoderma species can compete and command a large diversity of economically predominant plant pathogenic fungi, viruses, bacteria and nematodes (Figure 4). Among the huge obstacles to crops production are root-knot nematodes, including Meloidogyne javanica and Meloidogyne incognita, which are sedentary, obligatory root endoparasites of many economically significant plants. Hence, Trichoderma has been focused on its biocontrol efforts on these nematodes. Many Trichoderma species and isolates have been tested as biocontrol vehicles in opposition to the nematodes with many different crops and experimental circumstances. Remarkable answers to nematode control and plants growth were attained (Guzman-Guzman et al., 2023). Focusing to upgrade the biocontrol procedure, methods of operation of the fungus in opposition to the root-knot nematodes have been explored and are reported in this chapter. A variety of processes was investigated, including parasitism, enzymatic lysis, antibiosis, and induced resistance. Acknowledge the fungus-nematode-plant interactions and the operation of the biocontrol procedure might bestow to enhance the accomplishment of this biocontrol agent.
It was revealed by Reino et al. (2008) that the Trichoderma species are unbound-living fungi that are extremely interactive in root, soil, and foliar environments and have been successful in protecting large crops from pathogens in field trials. The structure and biology of the metabolites extracted from Trichoderma species have been reviewed. Furthermore, this comprehensive assessment, including all the literature on the subject up to the present day, in which 269 references are cited, summarizes the literature on the biological activity of the metabolites, particularly when used in biological control techniques (Jose et al., 2008).
Mukherjee, (2011) reported that entire genome sequencing of Gliocladium virens and T. atroviride, these two are the most effective antagonists of plant pathogenic fungi. Principal appreciation into the genetics of mycoparasitism and biological control are also discussed. Mukherjee et al., (2012) reported that antibiosis procedure comprises the making or delivery of compounds (antibiotics or hydrolytic enzymes) that will kill or hinder the maturity of pathogens. There has been a growing interest in Trichoderma strains for their production of volatile and non-volatile toxic metabolites, which are antagonistic to pathogens.
Induced systemic resistance
The great priority of Trichoderma investigation was to realize the direct consequence on other fungal species, especially mycoparasitism and antibiosis. According to Bigirimana et al. (1997) the first clear illustration of induced resistance of bean plants to fungi revealed that only a root application of T-39 enabled bean plants to withstand fungal foliar diseases caused by B. cinerea and C. lindemuthianum. Researchers found that over ten different dicots and monocots appear to be protected by the induced resistance from infections caused by fungi (B. cinerea, R. solani, Colletotrichum spp., Phytophthora spp., Alternaria spp., Magnaporthe grisea, etc.), bacteria (Xanthomonas spp., Pseudomonas syringae, etc.) and even viruses like CMV. B. cinerea and C. lindemuthianum were also effectively controlled in bean plants after soil was treated with T. harzianum strain T-39. Other dicots also exhibited similar behavior as B. cinerea (De Mayer et al., 1998).
According to Djonovic et al. (2007), induced systemic resistance in maize is regulated by proteinaceous elicitor Sm1, released by the beneficial fungus Trichoderma virens. Researchers previously demonstrated that the filamentous fungus Trichoderma virens induces resistance to systemic diseases in cotton (Gossypium hirsutum) by producing a highly effective hydrophobin-like elicitor called Sm1 (small proteins named after the scientist Stephanie Smith). A functional genomics approach was used to study the role of Sm1 during maize-fungal interactions by colonizing roots of T. virens and investigating whether systemic protection against a foliar pathogen is induced. A hydroponic system or a soil-grown maize seedling exposed to the pathogen Coletotrichum graminicola was used to immunize seedlings with T. virens Gv29-8 (wild type) and transformants that disrupted Sm1 or overexpressed it. In a similar manner to dicot plants, we demonstrated that T. virens colonization of maize roots induces systemic coverage of C. graminicola-immunized leaves. Jasmonic acid and green leaves volatile biosynthesis genes were important in this defense (Wang et al., 2020). T. virens did not undergo normal growth or development when Sm1 was removed or overexpressed, nor did the normal production of gliotoxin, hyphal coiling, or hydrophobicity, or their ability to colonize maize roots. The results of plant bioassays showed that plants grown with Sm1-elimination strains were just as protected as non-Trichoderma-treated plants. A functional decrease in disease protection was observed when Sm1 was eliminated, as well as an increase in disease protection when it was overexpressed. Based on these results, it appears that the functional Sm1 elicitor is necessary for active activation of systemic disease protection for T. virens (Figure 5).
Secondary metabolism
There are four major chemical classes of fungal SMs: polyketides, terpenoids, compounds derived from shikimic acid, and non-ribosomal peptides (Pusztahelyi et al., 2015). Aside from hybrid metabolites which comprise molecules from different classes, meroterpenoids are examples of fusions between terpenes and polyketides. It was found that ascomycetes had higher numbers of secondary metabolism genes compared to basidiomycetes, archeo-ascomycetes, and chytridiomycetes, whereas hemi-ascomycetes and zygomycetes have none (Collemare et al., 2008). Polyketide synthases (PKS) code for 16 number of the genome of an ascomycete, compared to 10 number for non-ribosomal protein synthases (NRPS), two for tryptophan synthases (TS), and two for dimethylallyl tryptophan synthases (DMATS), which are crucial for SM synthesis. Enzymes encoding these types of SM genes are found in gene clusters associated with secondary metabolism and are crucial for the main synthesis of metabolites. Ascomycetes have only been found to contain PKS-NRPSs, with an average of three genes per species. Human pathogens Coccidioides spp. and Histoplasma capsulatum as well as Neurospora crassa have fewer PKSs (1–9 genes), NRPS (3–6 genes), and PKS-NRPSs (0–2 genes) than other ascomycetes. It has been reported that many fungi contain more than 40 genes encoding PKS, NRPS, hybrids, TS, and DMATS in their genomes, including M. grisea (45 genes) (Collemare et al., 2008). Siderophores, which are SMs that possess iron uptake capability, are also synthesized by the NRPS, which is also essential for the virulence of several fungi (e.g., Cochliobolus heterostrophus, C. miyabeanus, F. graminearum, and A. brassicicola) (Oide et al., 2006).
There is direct as well as indirect evidence indicating that secondary metabolites (natural products) play a role in Trichoderma's antagonistic activity against several bacteria, yeasts, and fungi as well as in its beneficial effects on crops. Though not clinically used, some of the secondary metabolites produced by Trichoderma have potential as drugs and one compound (6-pentyl-α-pyrone) is used as a food flavoring agent. Trichoderma began to be studied in the 1930s with the discovery of gliotoxin among a plethora of substances (Weindling and Emerson, 1936). In 2014, Hermosa et al. (2014) estimated that about 1,000 compounds are produced by fungi of this genus (Figure 6).
CONCLUSION
The success of Trichoderma strains as biocontrol agents against different pathogenic microorganisms is well known worldwide. Trichoderma spp. have been widely used in agricultural applications due to their well-known biological control mechanism. Trichoderma has many useful mechanisms that can possibly be utilized in introducing the best microbe to the plants. The mechanisms comprise of (I) parasitism, (II) producing the secondary metabolites, antibiosis, and enzymes, (III) inducing the defense and resistance of plant, and (IV) enhancing the plant growth. These enable the capabilities of Trichoderma to control the plant pathogens in the soil, seeds, roots, stem, leaf, fruits, postharvest, insect and weeds. Thus, Trichoderma spp. can be possibly explored both as biopesticides and biofertilizers. Trichoderma spp., when combined in a product, are able to control different crop diseases, stimulate plant growth and development, improve the composting process, and promise a clean environment towards achieving sustainable agriculture.
REFERENCES
Abdelkhalek, A., Al-A. Abdulaziz, A. Amr and B. Said. 2022. Trichoderma hamatum strain Th23 promotes tomato growth and induces systemic resistance against Tobacco mosaic virus. J. Fungi. 8: 228.
Benitez, T., A. M. Rincon, M. C. Limn and A. C. Codon. 2004. Biocontrol mechanisms of Trichoderma strains. Int. J. Microbiol. 7(4): 249–260.
Chet, I., 1987. Trichoderma- application, mode of action and potential as a biocontrol agent of soil-borne plant pathogenic fungi. In: Innovative approaches to plant disease control (Ed. Chet, I.), Wiley-Interscience, New York.
Chet, I., N. Benhamou and S. Haran. 1998. Mycoparasitism and lytic enzymes. In: Trichoderma and Gliocladium (Vol. 2). Enzymes, biological control and commercial applications (Eds. Harman, G. E. and C. P. Kubicek), Taylor and Francis, London, 153–171.
Collemare, J., M. Pianfetti, A. E. Houlle, D. Morin, L. Camborde, M. J. Gagey, C. Barbisan, I. Fudal, M. H. Lebrun and H. U. Bohnert. 2008. Magnaporthe grisea avirulence gene ACE1 belongs to aninfection-specific gene cluster involved in secondary metabolism. New Phytologist. 179(1): 196–208.
de França, S. K. S., A. F. Cardoso, D. C. Lustosa, E. M. L. S. Ramos, M. C. C. de Filippi and G. B. da Silva. 2015. Biocontrol of sheath blight by Trichoderma asperellum in tropical lowland rice. Agron. Sustain. Dev. 35: 317–324.
Djonovic, S., W. A. Vargas, M. V. Kolomiets, M. Horndeski, A. Wiest and C. M. Kenerley. 2007. A proteinaceous elicitor Sm1 from the beneficial fungus Trichoderma virens is required for induced systemic resistance in maize. Plant Physiol. 145(3): 875–889.
Dukare, A. S., S. Paul, V. E. Nambi, R. K. Gupta, R. Singh, K. Sharma and R. K. Vishwakarma. 2019. Exploitation of microbial antagonists for the control of postharvest diseases of fruits: A review. Crit. Rev. Food Sci. Nutr. 59(9): 1498–1513.
Gajera, H., R. Domadiya, S. Patel, M. Kapopara and B. Golakiya. 2013. Molecular mechanism of Trichoderma as bio-control agents against phytopathogen system – a review. Curr. Res. Microbiol. Biotechnol. 1(4): 133–142.
Gams, W. and J. Bisset. 1998. Morphology and identification of Trichoderma. In: Trichoderma and Gliocladium ( Vol. 1). Basic biology, taxonomy and genetics (Eds. Harman, G.E. and C. P. Kubicek), Taylor and Francis, London, 3–31.
Guzman-Guzman, P., A. Kumar, S. Santos-Villalobos, F. I. Parra-Cota, D. C. Ma. Orozco-Mosqueda, A. E. Fadiji, S. Hyder, O. O. Babalola and G. Santoyo. 2023. Trichoderma Species: Our best fungal allies in the biocontrol of plant diseases-A review. Plants. 12(3): 432. https://doi.org/10.3390/plants12030432.
Harman, G. E., 2006. Overview of mechanisms and uses of Trichoderma spp. Phytopathol. 96(2): 190–194.
Harman, G., C. R. Howell, A. Viterbo, I. Chet, and M. Lorito. 2004. Trichoderma species - opportunistic, avirulent plant symbionts. Nature Reviews Microbiol. 2: 43–56.
Hermosa, R., R. E. Cardoza, M. B. Rubio, S. Gutiérrez and E. Monte. 2014. Secondary metabolism and antimicrobial metabolites of Trichoderma. Biotechnol. Biol. Trichoderma. 125–137.
Inbar, J. and I. Chet. 1992. Biomimics of fungal cell-cell recognition by use of lectin-coated nylon fibres. J. Bacteriol. 174(3): 1055–1059.
Izuogu, N. B. and T. O. Abiri. 2015. Efficacy of Trichoderma harzianum T22 as a biocontrol agent against root-knot nematode (Meloidogyne incognita) on some soybean varieties. Croatian J. Food Sci. Technol. 7: 47–51.
Jadav, P. C., G. M. Hegde, S. Jahagirdar, and V. Navi. 2022 Evaluation of microbial consortia against Sclerotium rolfsii Sacc. causing foot rot of wheat. J. Farm Sci. 35(2): 219–222.
Jose, L. R., R. F. Guerrero, R. Hernández-Galán and I. G. Collado. 2008. Secondary metabolites from species of the biocontrol agent Trichoderma. Phytochemistry Reviews. 7: 89-123.
Kuzmanovska, B., R. Rusevski, M. Jankulovska and K. B. Oreshkovikj. 2018. Antagonistic activity of Trichoderma asperellum and Trichoderma harzianum against genetically diverse Botrytis cinerea isolates. Chilean J. Agric. Res. 78(3): 391–399.
Lahlali, R., S. Ezrari, N. Radouane, J. Kenfaoui, Q. Esmaeel, H. El Hamss, Z. Belabess and E. A. Barka. 2022. Biological control of plant pathogens: A global perspective. Microorganisms. 10(3): 596.
Li, M., M. A. Guang-shu, L. I. A. N. Hua, S. U. Xiao-lin, T. I. A. N. Ying, H. U. A. N. G. Wen-kun, M. E. I. Jie and J. I. A. N. G. Xi-liang. 2019. The effects of Trichoderma on preventing cucumber Fusarium wilt and regulating cucumber physiology. J. Integr. Agric. 18(3): 607–617.
Lorito, M. and S. Woo. 2015. Trichoderma: A multi-purpose tool for integrated pest management. In: Lugtenberg, B. (eds) Principles of Plant-Microbe Interactions. Springer, Cham. https://doi.org/10.1007/978-3-319-08575-3_36.
Manganiello, G., A. Sacco, M. R. Ercolano, F. Vinale, S. Lanzuise, A. Pascale, M. Napolitano, N. Lombardi, M. Lorito and S. L. Woo. 2018. Modulation of tomato response to Rhizoctonia solani by Trichoderma harzianum and its secondary metabolite harzianic acid. Front. Microbiol. 9: 1966. doi: 10.3389/fmicb.2018.01966.
Mukherjee, M., P. K. Mukherjee, B. A. Horwitz, C. Zachow, G. Berg and S. Zeilinger. 2012. Trichoderma-plant-pathogen interactions: Advances in genetics of biological control. Indian J. Microbiol. 52(4): 522–529.
Naseby, D. C., J. A. Pascual and J. M. Lynch. 2000. Effect of biocontrol strains of Trichoderma on plant growth, Pythium ultimum populations, soil microbial communities and soil enzyme activities. J. Appl. Microbiol. 88(1): 161–169.
Nosir, W. S. 2016. Trichoderma harzianum as a growth promoter and bio-control agent against Fusarium oxysporum f. sp. tuberosi. Adv. Crop Sci. Tech. 4: 217. doi:10.4172/2329-8863.1000217.
Oide, S., S. B. Krasnoff, D. M. Gibson and B. G. Turgeon. 2006. Intracellular siderophores are essential for ascomycete sexual development in heterothallic Cochliobolus heterostrophus and homothallic Gibberella zeae. Eukaryotic Cell. 6(8): 1339–1353.
Persoon, C.H., 1794. Neuer Versuch einer systematischen Einteilunder Schwamme. Racodium Romer's Neues Magazin der Botanik. 1: 123.
Pusztahelyi, T., I. J. Holb and I. Pócsi. 2015. Secondary metabolites in fungus-plant interactions. Front. Plant Sci. 6: 573. doi: 10.3389/fpls.2015.00573.
Rahman, M.A., M. F. Begum and M. F. Alam. 2009. Screening of Trichoderma isolates as a biological control agent against Ceratocystis paradoxa causing pineapple disease of sugarcane. Mycobiol. 37(4): 277–285.
Rahman, M.A., M. F. Begum, M. F. Alam. 2009. Screening of Trichoderma isolates as a biological control agent against Ceratocystis paradoxa causing pineapple disease of sugarcane. Mycobiology. 37(4): 277–285.
Reino, J. L., R. F. Guerrero, R. Hernandez-Galan and I. G. Collado. 2008. Secondary metabolites from species of the biocontrol agent Trichoderma. Phytochemistry Reviews. 7(1): 89–123.
Reithner, B., E. Ibarra-Laclette, R. L. Mach and A. Herrera-Estrella. 2011. Identification of mycoparasitism-related genes in Trichoderma atroviride. App. Environ. Microbiol. 77(13): 4361–4370.
Rifai, M. A., 1969. A revision of the genus Trichoderma. Mycological Papers. 116: 1–56.
Samuel, G. J., 1996. Trichoderma: A review of biology and systematic of the genus. Mycol. Res. 100: 923-935.
Samuels, G. J., 2006. Trichoderma: Systematic, the Sexual State and Ecology. Phytopathology. 96(2): 195–206.
Saravanakumar, K., L. Fan, K. Fu. et al. 2016. Cellulase from Trichoderma harzianum interacts with roots and triggers induced systemic resistance to foliar disease in maize. Sci. Rep. 6: 35543. https://doi.org/10.1038/srep35543.
Sarrocco, S., L. Guidi, S. Fambrini, E. DelInnocenti and G. Vannacci. 2009. Competition for cellulose exploitation between Rhizoctonia solani and two Trichoderma isolates in the decomposition of wheat straw. J. Plant Pathol. 91(2): 331–338.
Sharon, E., I. Chet and Y. Spiegel. 2011. Trichoderma as a biological control agent. Biological Control of Plant-Parasitic Nematodes. 183–201.
Singh, U. S., N. W. Zaidi, D. Joshi, S. Varshney and T. Khan. 2006. Current status of Trichoderma as a biocontrol agent. In: Current status of biological control of plant diseases using antagonistic organisms in India (Eds. Ramanujam, B. and R. J. Rabindra), Project Directorate of Biological Control, Bangalore.
Sui, L., J. Li, J. Philp, K. Yang, Y. Wei, H. Li, J. Li, L. Li, M. Ryder, R. Toh, Y. Zhou, M. D. Denton, J. Hu and Y. Wang. 2022. Trichoderma atroviride seed dressing influenced the fungal community and pathogenic fungi in the wheat rhizosphere. Sci. Rep. 12(1): 9677.
Tjamos, E. C., G. C. Papavizas, and R. J. Cook. 1922. Biological control of plant diseases. Progress and challenges for the future. Plenum Press, New York, 222.
Tseng, Y-H., H. Rouina, K. Groten, P. Rajani, A. C. U. Furch, M. Reichelt, I. T, Baldwin, K. N. Nataraja, R. Uma Shaanker and R. Oelmüller. 2020. An endophytic Trichoderma strain promotes growth of its hosts and defends against pathogen attack. Front. Plant Sci. 11: 573670.
Tyśkiewicz, R., A. Nowak, E. Ozimek and J. Jaroszuk-Ściseł. 2022. Trichoderma: The current status of its application in agriculture for the biocontrol of fungal phytopathogens and stimulation of plant growth. Int, J, Mol, Sci. 23(4): 2329.
Uddin, M. M., N. Akhtar, M. T. Islamand and A. N. Faruq. 2011. Effect of Trichoderma harzianum and some selected soil amendment against damping off disease complex of potato and chilli. The Agriculturists. 9(1&2): 106–116.
Wang, K. D., Z. Gorman, P. C. Huang, C. M. Kenerley and M. V. Kolomiets. 2020. Trichoderma virens colonization of maize roots triggers rapid accumulation of 12-oxophytodienoate and two ⍺-ketols in leaves as priming agents of induced systemic resistance. Plant Signal. Behav. 15(9): 1792187. doi: 10.1080/15592324.2020.1792187.
Weindling and Emerson. 1936. The isolation of a toxic substance from the culture filtrate of Trichoderma. Phytopathology. 26(3): 1068–1070.
Wonglom, P., W. Daengsuwan, S-i. Ito and A. Sunpapao. 2019. Biological control of Sclerotium fruit rot of snake fruit and stem rot of lettuce by Trichoderma sp. T76-12/2 and the mechanisms involved. Physiol. Mol. Plant Pathol. 107: 1–7.
Woo, S. L., F. Scala, M. Ruocco and M. Lorito. 2006. The molecular biology of the interactions between Trichoderma spp., pathogenic fungi and plants. Phytopathology. 96: 181–185.
Zeilinger, S., C. Galhaup, K. Payer, S. L. Woo, R. L. Mach, C. Fekete, M. Lorito and C. P. Kubicek. 1999. Chitinase gene expression during mycoparasitic interaction of Trichoderma harzianum with its host. Fungal Genetics and Biology. 26(2): 131–140.
Zin, N. A. and N. A. Badaluddin. 2020. Biological functions of Trichoderma spp. for agriculture applications, Ann. Agric. Sci. 65(2): 168–178.