DOI: https://doi.org/10.56669/SOLS6960
ABSTRACT
BioGroe™ is a solid-based microbial plant growth promoter which contains plant growth promoting bacteria (PGPB) which was produced and developed in the National Institute of Molecular Biology and Biotechnology – University of the Philippines at Los Baños (BIOTECH-UPLB). It promotes root growth through plant growth hormone production and providing nutrients in soluble form. It can be seed inoculated, mixing the product directly to the seed during planting, or it can me mixed with water for root dipping or applied during watering. BioGroe™ aims to reduce the increasing chemical fertilizer usage of local farmers without trimming down their yield and income. It provides an environmentally friendly system which can facilitate our effort against climate change. The results of the demonstration trials conducted showed the effectiveness of substituting 50% of chemical fertilizer with the BioGroe™. Results of the cost and benefit studies revealed positive net change in income when the chemical fertilizers used in selected vegetables i.e. pechay and mustard were reduced to only half of the recommended rate. On a per hectare basis and based on demo trials conducted increases in incomes were attained through the application of BioGroe™ on pechay and mustard amounting to Php41,615.00 (US$735) and Php69,700.00 (US$1,230). The use of BioGroe™ has great potential to increase crop yields with lower cost of production resulting to higher income of farmers. Reduction of importation of chemical fertilizers will also be realized upon commercialization of BioGroe™ production.
Keywords: microbial inoculant, PGPB, plant hormones, mustard, yield
INTRODUCTION
An increasing demand for low-input agriculture has resulted in greater interest in soil microorganisms that increases soil fertility or improve plant nutrition and health. The nutrient rich rhizosphere of roots is naturally colonized by many beneficial or pathogenic bacteria and fungi, which may have a considerable impact on plant growth, development and productivity. Different physiological processes are mainly dominated by these different microorganisms which are important for long-term sustainability.
Root-associated bacteria termed plant growth promoting bacteria (PGPB) provide plant with nitrogen obtained from the atmosphere through nitrogen fixation, influence root growth by producing plant hormones and protect roots from disease through production of antibiotics (Lynch, 1983). Studies have shown that plant hormones produced by soil microorganisms are involved in plant growth promotion and development. This is attributed to the production of plant growth regulators (PGRs) such as indole-acetic acid (IAA), cytokinins and gibberellins (Glick, 1995). PGPB were suggested for use on a number of crops to enhance plant growth, development and yield.
The soil is composed of bacteria, fungi, actinomycetes, protozoa, and algae. It has been known for some time that the soil hosts a large number of bacteria (often around 108 to 109 cells per gram of soil) and that the number of culturable bacterial cells in soil is generally only about 1% of the total number of cells present (Schoenborn et al., 2004). These bacteria can act in two ways to improve the fitness of its hosts, either directly or indirectly (Gómez-Godínez et al., 2023). Among the direct mechanisms are those that promote plant growth. Within these, we find the production of hormones, such as auxins, cytokinins, and gibberellins, as well as nitrogen fixation and phosphorus solubilization. The indirect mechanisms are related to inhibiting the function of one or more plant-pathogenic organisms (Beneduzi et al., 2012).
Microbial inoculants combined or separate, can be inoculated into seed, leave, seedling roots, or soil (Lopes et al., 2021). They colonize the rhizosphere or the interior of the plant, stimulating growth and plant tolerance against abiotic stresses. On the other hand, beneficial microbes improve plant growth by enhancing the availability of nutrients, the regulation of phytohormones, and by increasing plant tolerance against biotic and abiotic stresses. The effects of stress on plants are mitigated by microbial inoculants, through the production of auxin, cytokinin, gibberellin, ACC deaminase, abscisic acid, jasmonates, brassinosteroids, and strigolactones (Oosten et al., 2017; Arora et al., 2020; Khan et al., 2020).
Inoculation of plants with PGPB resulted in significant changes of various growth characteristics: promotion of mineral and water uptake, dry matter accumulation, root surface area, root diameter and density, and length of root hairs. Changes in the soil Total N, available P, K, Ca, Mg, Fe, Mn and Zn contents were also reported (Orhan et al., 2006). Preliminary studies conducted under PCASTRD-funded project has able to enhance root development and shoot growth of papaya using Azotobacter vinelandii Mac 259 with combined nitrogen fertilizer (Paterno et al., 1996). The improved root growth apparently led to more efficient nutrient absorption. PGPB isolates were then screened for growth promotion of selected crops under controlled conditions, by evaluating their ability to produce PGRs. The selected isolates were packed into a user- and environment-friendly bioinoculant called BioGroe™.
BioGroe™ technology has a great potential to increase crop yields with lower cost of production. A reduction in cost will result in increased net benefit per area planted to the crop and provide higher income to farmers. Commercialization of this technology is expected to alleviate poverty among small farmers in the country.
The beneficial effects of BioGroe™ has been observed since the early stages of the product. The application of the recommended rate of the inoculant increased the total marketable yield of ampalaya or bittergourd by 63% and the total number of fruits by 50% relative to the control. The potential and actual yields were 42% and 63%, respectively, higher than the control. These results suggested that the application of BioGroe™ can significantly increase the yield of bittergourd. Sugarcane yields also increased with the inoculation of the inoculant with 6.6% to 7.6% over the control treatments (Paterno, 2005). Also, inoculation of PGPB in tomato, beans, barley, wheat, corn, etc., has resulted in improved yields.
For the technology to be used, trainings and seminars throughout the region were organized to increase the awareness of the local farmers. With coordination with the Department of Agriculture and local farmer groups, the benefits and use of the inoculant was taught. Partnership with private investors was also established in order to commercialize and mass produce the product and provide a mode of marketing and distribution in their respective areas.
The variety used was Black Behi for pechay and Monteverde for mustard. Seeds were sown in sowing beds first before transplanting in their respective plots. At sowing, the plants that was used for the BioGroe™ treated plots were watered by the inoculant suspension (2L water: 1pack BioGroe™). Two weeks after sowing, plants were transplanted in their respective plots and for the treated plants, another inoculation was done by root dipping. Samples were gathered for another two weeks. The data gathered were the marketable and dry matter yield. This set-up was done at Rosario, Batangas and Alfonso, Cavite. Another set-up was done at Sto. Tomas, Batangas. The seeds were not transplanted but broadcasted throughout the field. The method of inoculation used was seed coating.
METHODOLOGY
Efficacy Trials for Pechay and Mustard
Site Description
The field trial was conducted at the UPLB experimental station located at Brgy. Tranca, Bay, Laguna. The soil used belongs to the Lipa series. The physiological position of the experiment site is plain. The land from the surrounding area is flat and the site has a 0.2% slope.
Trial Conditions
The experiment was conducted using a Randomized Complete Block Design (RCBD) with four replications. The treatments used were the following:
- Control - (no chemical fertilizer)
- Full Recommended Rate of Chemical Fertilizer (RRC): 150- 60-60 (NPK)
- Half RRC: 60-30-30 (NPK)
- Full RRC + BioGroe™
- Half RRC + BioGroe™
- Half RRC + 2x BioGroe™
Pechay var. Black Behi was used during the experiment and was sown in sowing beds. The seedlings were transplanted 10 days after sowing in each respective block with 1m x 10m plot size. They were placed 10cm between hills and 10cm between rows.
Method and time of application of the product
BioGroe™ Plant Growth Promoter was irrigated in the seedling beds at sowing by mixing a pack (100g) into 2L of water. A second application was conducted during transplanting by dipping the roots of the seedlings in 2L suspension of the inoculant. The application of N fertilizer as urea was done at planting and a week after transplanting.
BioGroe™ efficacy trials on pechay and mustard were conducted in Brgy. Poblacion I, Rosario, Batangas, with the cooperation of Mr. Rodrigo Balbaira. The effect of BioGroe™ inoculation with increasing rate of chemical fertilizer on the performance of the test crops was evaluated. The fertilizer treatments were: no chemical fertilizer, ¼ RRC, ½ RRC, ¾ RRC and Full RRC (RRC) and their corresponding BioGroe™ inoculated counterpart. The experimental design was 2 Factorial in Randomized Complete Block Design (RCBD), replicated three times, and each block consists of a 1m x 5m bed. The data was analyzed using a Two-factor ANOVA model.
RESULTS AND DISCUSSION
Efficacy Trials in Pechay and Mustard
Efficacy trial on pechay was conducted at the experimental station in Brgy. Tranca, Bay, Laguna, Philippines. Results showed that the highest yield was obtained with the RRC + BioGroe™ treatment with 4.39 kg/m² which is significantly higher than the Control and about 16% higher than with RRC alone (3.66 kg/m²) (Table 1). Treatments with ½ RRC, ½ RRC + BioGroe™ and ½ RRC + 2x BioGroe™ did not show significant differences in yield. The lowest yields were obtained in the Control, ¼ RRC and ¼ RRC + BioGroe.
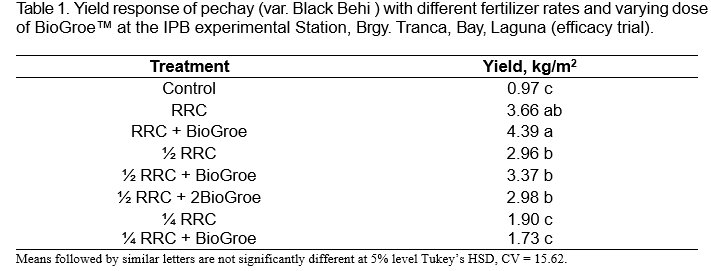
In mustard, results showed that the highest yield was obtained with the RRC + BioGroe™ treatment with 2.12 kg/m², which is about 66% higher than the Control (Table 2). The lowest yields were obtained in the Control, ¼ RRC and ¾ RRC.
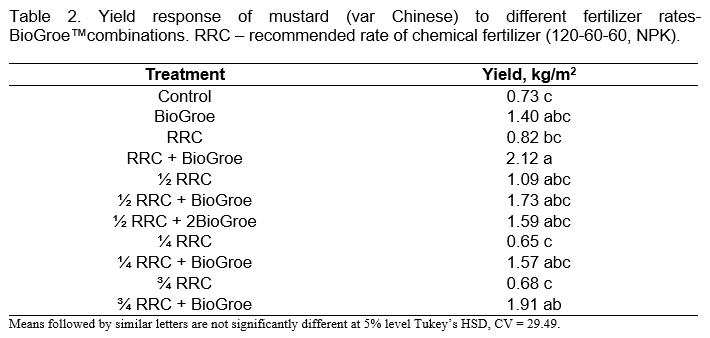
Generally, microbial inoculants promote crop yield through direct improvement of plant nutrient availability or through alleviating plant stresses such as drought, disease and salt stress (Bakhshandeh et al., 2020; Woo and Pepe, 2018). A diverse array of microbial inoculants from genera Azospirillum, Azotobacter, Pseudomonas, Bacillus, Enterobacter, Burkholderia, Serratia and Rhizobium are capable of facilitating the growth of vegetable and legume crops (Bhattacharyya and Jha, 2012; Hayat et al., 2010). Farmers are now more receptive to the use of inoculants mainly because high-quality products and multi-purpose elite strains are available at the market, improving yields at low cost in comparison to chemical fertilizers (Santos et al., 2019). In the context of a more sustainable agriculture, microbial inoculants also help to mitigate environmental impacts caused by agrochemicals. Challenges rely on the production of microbial inoculants for a broader range of crops, and the expansion of the inoculated area worldwide.
However, the active use of PGPB sometimes encounters challenges in agricultural application, because of the inconsistent efficiency and vitality of PGPB (Vegan et al., 2016; Ji, et al., 2019). First, PGPB is not broadly efficient to many plant species, and therefore field application can generate inconsistent outcomes in productivity. In particular, the surrounding environment and microbial community can affect the activity of PGPB. Secondly, colonization of PGPB on and in plants is not always stably maintained, because changeable environments and plant species can affect the success of PGPB establishment (Dessaux et al., 2016).
Despite the large number of reports showing the advantages of the use of PGPB in crops, the application of these microorganisms on the fields is still little explored in comparison to the total amount of agricultural land of the world. Bacterial formulations with PGPB have not always the desired effectiveness (Dobbelaere et al., 2001). The capability of microorganisms to promote the growth of plants in crop fields dependent of several factors that limit their effectiveness, for example, soil types, climatic conditions, variety of the crop, bacterial genotype, effectiveness of the bacterial isolate, the proper inoculation technology and others (Bashan, 1998; Bashan et al., 2014).
Practical use and commercialization of PGPB as biofertilizers in agriculture started worldwide in the 1950s (Timmusk et al., 2017). According to Glick (2012), for the more extensive commercialization of PGPB strains, a number of issues need to be addressed. These include (i) determination of those traits that are most important for efficacious functioning and subsequent selection of PGPB strains with appropriate biological activities; (ii) consistency among regulatory agencies in different countries regarding what strains can be released to the environment, and under what conditions genetically engineered strains are suitable for environmental use; (iii) a better understanding of the advantages and disadvantages of using rhizospheric versus endophytic bacteria; (iv) selection of PGPB strains that function optimally under specific environmental conditions (e.g., those that work well in warm and sandy soils versus organisms better adapted to cool and wet environments); (v) development of more effective means of applying PGPB to plants in various settings (e.g., in the field versus in the greenhouse); (vi) a better understanding of the potential interactions between PGPB and mycorrhizae and other soil fungi.
CONCLUSION
For the efficacy trials, significant increase in yield were observed at the Rosario, Batangas trial. A significant increase in yield in pechay was obtained from three fertilizer treatments: 1/4 RRC, 1/2 RRC and 3/4 RRC with increases ranging from 36 to 94%. The highest yield increase due to BioGroe™ inoculation was obtained from 1/2 RRC treatment with yield of 2.47 t ha-1. Actual yield values showed that with BioGroe™ inoculation, the highest yield of 2.47 kg m2 was obtained from 1/2 RRC treatment followed by RRC plots and the lowest harvest of 1.49 kg m2 from the unfertilized plots. Without inoculation, the highest yield was obtained from RRC plots while the lowest was obtained from 1/2 RRC plots. For mustard, all the fertilizer rate- BioGroe™ combinations showed significant increase in yield, with yield increases ranging from 60 to 180%, with the highest increase obtained from 3/4 RRC + BG . The low yield increase in ½ RRC treatment could be attributed to the relatively high yield obtained from the uninoculated ½ RRC plots. Actual yield values however showed that the highest yield among inoculated treatments was obtained from inoculated plots with full RRC applied followed by plots with 3/4 RRC with yields of 2.12 and 1.91 kg m2, respectively. For corn, the efficacy trial results showed that there were no significant differences found between the treatments: RRC, ½ RRC + BioGroe™, ½ RRC + 2x BioGroe™ and RRC + BioGroe™. But significant differences were present in terms of dry matter partitioning and dry matter yield.
ACKNOWLEDGEMENT
We are grateful for the funding support of the Department of Science and Technology-Technology Innovation for Commercialization (DOST-TECHNICOM) and the generosity of our farmer collaborators.
REFERENCE
Arora, N. K., Fatima, T., Mishra, I., and Verma, S. 2020. “Microbe-based inoculants: role in next green revolution,” in Environmental Concerns and Sustainable Development, eds V. Shukla and N. Kumar (Singapore: Springer), 191–245. doi: 10.1007/978-981-13-6358-0_9
Bakhshandeh, E., Gholamhosseini, M., Yaghoubian, Y., Pirdashti, H. 2020. Plant growth promoting microorganisms can improve germination, seedling growth and potassium uptake of soybean under drought and salt stress. Plant Growth Regul. 90: 123–36.
Bashan, Y. 1998. Inoculants of plant growth-promoting bacteria for use in agriculture. Biotechnol Adv 16: 729–770.
Bashan, Y., de-Bashan, L.E., Prabhu, S.R., and Hernandez, J.-P. 2014. Advances in plant growth-promoting bacterial inoculant technology: formulations and practical perspectives (1998-2013). Plant Soil 378: 1–33.
Beneduzi, A., Ambrosini, A., and Passaglia, L.M.P. Plant growth-promoting rhizobacteria (PGPR): Their potential as antagonists and biocontrol agents. 2012. Genet. Mol. Biol. 35 (Suppl. 4): 1044–1051.
Bhattacharyya, P.N., Jha, D.K. 2012. Plant growth-promoting rhizobacteria (PGPR): emergence in agriculture. World J Microbiol Biotechnol. 28: 1327–50.
Dessaux, Y., Grandclément, C. & Faure, D. 2016. Engineering the rhizosphere. Trends in Plant Science 21, 266–278.
Dobbelaere, S., Croonenborghs, A., Thys, A., Ptacek, D., Vanderleyden, J., Dutto, P., et al. 2001. Responses of Agronomically important crops to inoculation with Azospirillum. Aust J Plant Physiol 28: 871–879.
Glick, B.R. 1995. The enhancement of plant growth by free living bacteria. Can. J, microbial. 43:109-117.
Glick, B.R. 2012. Plant growth-promoting bacteria: Mechanisms and applications", Scientifica. doi.org/10.6064/2012/963401
Gómez-Godínez, L.J.; Aguirre-Noyola, J.L.; Martínez-Romero, E.; Arteaga-Garibay, R.I.; Ireta-Moreno, J.; Ruvalcaba-Gómez, J.M. 2023. A look at plant-growth-promoting bacteria. Plants. 12: 1668. https://doi.org/10.3390/plants12081668
Hayat, R., Ali, S., Amara, U., Khalid, R., Ahmed, I. 2010. Soil beneficial bacteria and their role in plant growth promotion: a review. Ann Microbiol. 60: 579–98.
Ji, S.H., Kim, J.S., Lee, C.H., Seo, H.S., Chun, S.H., Oh, J., Choi, E.H., Park, G. 2019. Enhancement of vitality and activity of a plant growth-promoting bacteria (PGPB) by atmospheric pressure non-thermal plasma. Sci Rep 9, 1044. https://doi.org/10.1038/s41598-018-38026-z
Khan, N., Bano, A., Ali, S., and Babar, M.d. A. 2020. Crosstalk amongst phytohormones from planta and PGPR under biotic and abiotic stresses. Plant Growth Regul. 90, 189–203. doi: 10.1007/s10725-020-00571-x
Lopes, M.J.S., Dias-Filho, M.B. and Gurgel, E.S.C. 2021. Successful plant growth-promoting microbes: Inoculation methods and abiotic factors. Front. Sustain. Food Syst. 5:606454. doi: 10.3389/fsufs.2021.606454
Oosten, M. J. V., Pepe, O., Pascale, S., Silletti, S., and Maggio, A. 2017. The role of biostimulants and bioeffectors as alleviators of abiotic stress in crop plants. Chem. Biol. Technol. Agric. 4:5. doi: 10.1186/s40538-017-0089-5
Orhan, E., Esitken, A., Ercisli, S., Turan, M., Sahin, F., 2006. Effects of plant growth promoting Rhizobacteria (PGPR) on yield, growth and nutrient contents in organically growing raspberry. Scientia Horticulturae, Vol. 111, Issue 1, December 2006, pp. 38-43.
Paterno, E.S, M.L.Q. Sison, F.G. Torres, E.S. Garcia, S.M. Pantua and M.E. Orlina. 1996. Development of microbial inoculants for stressed environments. Terminal Report. BIOTECH-UPLB-PCASTRD Project.
Santos, M.S., Nogueira, M.A. & Hungria, M. Microbial inoculants: reviewing the past, discussing the present and previewing an outstanding future for the use of beneficial bacteria in agriculture. AMB Expr 9, 205 (2019). https://doi.org/10.1186/s13568-019-0932-0
Schoenborn, L., Yates, P. S., Grinton, B. E., Hugenholtz, P. and Janssen, P. H. 2004. Liquid serial dilution is inferior to solid media for isolation of cultures representative of the phylum-level diversity of soil bacteria. Applied and Environmental Microbiology. 70 (7): 4363–4366.
Timmusk, S., Behers, L., Muthoni, J., Muraya, A. & Aronsson, A.-C. 2017. Perspectives and challenges of microbial application for crop improvement. Frontiers in Plant Science. 8: 49.
Vejan, P., Abdullah, R., Khadiran, T., Ismail, S. & Nasrulhaq Boyce, A. 2016. Role of plant growth promoting rhizobacteria in agricultural sustainability - a review. Molecules 21, 573.
Woo, S.L., Pepe, O. 2018. Microbial consortia: promising probiotics as plant biostimulants for sustainable agriculture. Front Plant Sci. 9: 1801.
Efficacy Trials of BioGroe® Microbial Inoculant for Improved Production of Vegetables
DOI: https://doi.org/10.56669/SOLS6960
ABSTRACT
BioGroe™ is a solid-based microbial plant growth promoter which contains plant growth promoting bacteria (PGPB) which was produced and developed in the National Institute of Molecular Biology and Biotechnology – University of the Philippines at Los Baños (BIOTECH-UPLB). It promotes root growth through plant growth hormone production and providing nutrients in soluble form. It can be seed inoculated, mixing the product directly to the seed during planting, or it can me mixed with water for root dipping or applied during watering. BioGroe™ aims to reduce the increasing chemical fertilizer usage of local farmers without trimming down their yield and income. It provides an environmentally friendly system which can facilitate our effort against climate change. The results of the demonstration trials conducted showed the effectiveness of substituting 50% of chemical fertilizer with the BioGroe™. Results of the cost and benefit studies revealed positive net change in income when the chemical fertilizers used in selected vegetables i.e. pechay and mustard were reduced to only half of the recommended rate. On a per hectare basis and based on demo trials conducted increases in incomes were attained through the application of BioGroe™ on pechay and mustard amounting to Php41,615.00 (US$735) and Php69,700.00 (US$1,230). The use of BioGroe™ has great potential to increase crop yields with lower cost of production resulting to higher income of farmers. Reduction of importation of chemical fertilizers will also be realized upon commercialization of BioGroe™ production.
Keywords: microbial inoculant, PGPB, plant hormones, mustard, yield
INTRODUCTION
An increasing demand for low-input agriculture has resulted in greater interest in soil microorganisms that increases soil fertility or improve plant nutrition and health. The nutrient rich rhizosphere of roots is naturally colonized by many beneficial or pathogenic bacteria and fungi, which may have a considerable impact on plant growth, development and productivity. Different physiological processes are mainly dominated by these different microorganisms which are important for long-term sustainability.
Root-associated bacteria termed plant growth promoting bacteria (PGPB) provide plant with nitrogen obtained from the atmosphere through nitrogen fixation, influence root growth by producing plant hormones and protect roots from disease through production of antibiotics (Lynch, 1983). Studies have shown that plant hormones produced by soil microorganisms are involved in plant growth promotion and development. This is attributed to the production of plant growth regulators (PGRs) such as indole-acetic acid (IAA), cytokinins and gibberellins (Glick, 1995). PGPB were suggested for use on a number of crops to enhance plant growth, development and yield.
The soil is composed of bacteria, fungi, actinomycetes, protozoa, and algae. It has been known for some time that the soil hosts a large number of bacteria (often around 108 to 109 cells per gram of soil) and that the number of culturable bacterial cells in soil is generally only about 1% of the total number of cells present (Schoenborn et al., 2004). These bacteria can act in two ways to improve the fitness of its hosts, either directly or indirectly (Gómez-Godínez et al., 2023). Among the direct mechanisms are those that promote plant growth. Within these, we find the production of hormones, such as auxins, cytokinins, and gibberellins, as well as nitrogen fixation and phosphorus solubilization. The indirect mechanisms are related to inhibiting the function of one or more plant-pathogenic organisms (Beneduzi et al., 2012).
Microbial inoculants combined or separate, can be inoculated into seed, leave, seedling roots, or soil (Lopes et al., 2021). They colonize the rhizosphere or the interior of the plant, stimulating growth and plant tolerance against abiotic stresses. On the other hand, beneficial microbes improve plant growth by enhancing the availability of nutrients, the regulation of phytohormones, and by increasing plant tolerance against biotic and abiotic stresses. The effects of stress on plants are mitigated by microbial inoculants, through the production of auxin, cytokinin, gibberellin, ACC deaminase, abscisic acid, jasmonates, brassinosteroids, and strigolactones (Oosten et al., 2017; Arora et al., 2020; Khan et al., 2020).
Inoculation of plants with PGPB resulted in significant changes of various growth characteristics: promotion of mineral and water uptake, dry matter accumulation, root surface area, root diameter and density, and length of root hairs. Changes in the soil Total N, available P, K, Ca, Mg, Fe, Mn and Zn contents were also reported (Orhan et al., 2006). Preliminary studies conducted under PCASTRD-funded project has able to enhance root development and shoot growth of papaya using Azotobacter vinelandii Mac 259 with combined nitrogen fertilizer (Paterno et al., 1996). The improved root growth apparently led to more efficient nutrient absorption. PGPB isolates were then screened for growth promotion of selected crops under controlled conditions, by evaluating their ability to produce PGRs. The selected isolates were packed into a user- and environment-friendly bioinoculant called BioGroe™.
BioGroe™ technology has a great potential to increase crop yields with lower cost of production. A reduction in cost will result in increased net benefit per area planted to the crop and provide higher income to farmers. Commercialization of this technology is expected to alleviate poverty among small farmers in the country.
The beneficial effects of BioGroe™ has been observed since the early stages of the product. The application of the recommended rate of the inoculant increased the total marketable yield of ampalaya or bittergourd by 63% and the total number of fruits by 50% relative to the control. The potential and actual yields were 42% and 63%, respectively, higher than the control. These results suggested that the application of BioGroe™ can significantly increase the yield of bittergourd. Sugarcane yields also increased with the inoculation of the inoculant with 6.6% to 7.6% over the control treatments (Paterno, 2005). Also, inoculation of PGPB in tomato, beans, barley, wheat, corn, etc., has resulted in improved yields.
For the technology to be used, trainings and seminars throughout the region were organized to increase the awareness of the local farmers. With coordination with the Department of Agriculture and local farmer groups, the benefits and use of the inoculant was taught. Partnership with private investors was also established in order to commercialize and mass produce the product and provide a mode of marketing and distribution in their respective areas.
The variety used was Black Behi for pechay and Monteverde for mustard. Seeds were sown in sowing beds first before transplanting in their respective plots. At sowing, the plants that was used for the BioGroe™ treated plots were watered by the inoculant suspension (2L water: 1pack BioGroe™). Two weeks after sowing, plants were transplanted in their respective plots and for the treated plants, another inoculation was done by root dipping. Samples were gathered for another two weeks. The data gathered were the marketable and dry matter yield. This set-up was done at Rosario, Batangas and Alfonso, Cavite. Another set-up was done at Sto. Tomas, Batangas. The seeds were not transplanted but broadcasted throughout the field. The method of inoculation used was seed coating.
METHODOLOGY
Efficacy Trials for Pechay and Mustard
Site Description
The field trial was conducted at the UPLB experimental station located at Brgy. Tranca, Bay, Laguna. The soil used belongs to the Lipa series. The physiological position of the experiment site is plain. The land from the surrounding area is flat and the site has a 0.2% slope.
Trial Conditions
The experiment was conducted using a Randomized Complete Block Design (RCBD) with four replications. The treatments used were the following:
Pechay var. Black Behi was used during the experiment and was sown in sowing beds. The seedlings were transplanted 10 days after sowing in each respective block with 1m x 10m plot size. They were placed 10cm between hills and 10cm between rows.
Method and time of application of the product
BioGroe™ Plant Growth Promoter was irrigated in the seedling beds at sowing by mixing a pack (100g) into 2L of water. A second application was conducted during transplanting by dipping the roots of the seedlings in 2L suspension of the inoculant. The application of N fertilizer as urea was done at planting and a week after transplanting.
BioGroe™ efficacy trials on pechay and mustard were conducted in Brgy. Poblacion I, Rosario, Batangas, with the cooperation of Mr. Rodrigo Balbaira. The effect of BioGroe™ inoculation with increasing rate of chemical fertilizer on the performance of the test crops was evaluated. The fertilizer treatments were: no chemical fertilizer, ¼ RRC, ½ RRC, ¾ RRC and Full RRC (RRC) and their corresponding BioGroe™ inoculated counterpart. The experimental design was 2 Factorial in Randomized Complete Block Design (RCBD), replicated three times, and each block consists of a 1m x 5m bed. The data was analyzed using a Two-factor ANOVA model.
RESULTS AND DISCUSSION
Efficacy Trials in Pechay and Mustard
Efficacy trial on pechay was conducted at the experimental station in Brgy. Tranca, Bay, Laguna, Philippines. Results showed that the highest yield was obtained with the RRC + BioGroe™ treatment with 4.39 kg/m² which is significantly higher than the Control and about 16% higher than with RRC alone (3.66 kg/m²) (Table 1). Treatments with ½ RRC, ½ RRC + BioGroe™ and ½ RRC + 2x BioGroe™ did not show significant differences in yield. The lowest yields were obtained in the Control, ¼ RRC and ¼ RRC + BioGroe.
In mustard, results showed that the highest yield was obtained with the RRC + BioGroe™ treatment with 2.12 kg/m², which is about 66% higher than the Control (Table 2). The lowest yields were obtained in the Control, ¼ RRC and ¾ RRC.
Generally, microbial inoculants promote crop yield through direct improvement of plant nutrient availability or through alleviating plant stresses such as drought, disease and salt stress (Bakhshandeh et al., 2020; Woo and Pepe, 2018). A diverse array of microbial inoculants from genera Azospirillum, Azotobacter, Pseudomonas, Bacillus, Enterobacter, Burkholderia, Serratia and Rhizobium are capable of facilitating the growth of vegetable and legume crops (Bhattacharyya and Jha, 2012; Hayat et al., 2010). Farmers are now more receptive to the use of inoculants mainly because high-quality products and multi-purpose elite strains are available at the market, improving yields at low cost in comparison to chemical fertilizers (Santos et al., 2019). In the context of a more sustainable agriculture, microbial inoculants also help to mitigate environmental impacts caused by agrochemicals. Challenges rely on the production of microbial inoculants for a broader range of crops, and the expansion of the inoculated area worldwide.
However, the active use of PGPB sometimes encounters challenges in agricultural application, because of the inconsistent efficiency and vitality of PGPB (Vegan et al., 2016; Ji, et al., 2019). First, PGPB is not broadly efficient to many plant species, and therefore field application can generate inconsistent outcomes in productivity. In particular, the surrounding environment and microbial community can affect the activity of PGPB. Secondly, colonization of PGPB on and in plants is not always stably maintained, because changeable environments and plant species can affect the success of PGPB establishment (Dessaux et al., 2016).
Despite the large number of reports showing the advantages of the use of PGPB in crops, the application of these microorganisms on the fields is still little explored in comparison to the total amount of agricultural land of the world. Bacterial formulations with PGPB have not always the desired effectiveness (Dobbelaere et al., 2001). The capability of microorganisms to promote the growth of plants in crop fields dependent of several factors that limit their effectiveness, for example, soil types, climatic conditions, variety of the crop, bacterial genotype, effectiveness of the bacterial isolate, the proper inoculation technology and others (Bashan, 1998; Bashan et al., 2014).
Practical use and commercialization of PGPB as biofertilizers in agriculture started worldwide in the 1950s (Timmusk et al., 2017). According to Glick (2012), for the more extensive commercialization of PGPB strains, a number of issues need to be addressed. These include (i) determination of those traits that are most important for efficacious functioning and subsequent selection of PGPB strains with appropriate biological activities; (ii) consistency among regulatory agencies in different countries regarding what strains can be released to the environment, and under what conditions genetically engineered strains are suitable for environmental use; (iii) a better understanding of the advantages and disadvantages of using rhizospheric versus endophytic bacteria; (iv) selection of PGPB strains that function optimally under specific environmental conditions (e.g., those that work well in warm and sandy soils versus organisms better adapted to cool and wet environments); (v) development of more effective means of applying PGPB to plants in various settings (e.g., in the field versus in the greenhouse); (vi) a better understanding of the potential interactions between PGPB and mycorrhizae and other soil fungi.
CONCLUSION
For the efficacy trials, significant increase in yield were observed at the Rosario, Batangas trial. A significant increase in yield in pechay was obtained from three fertilizer treatments: 1/4 RRC, 1/2 RRC and 3/4 RRC with increases ranging from 36 to 94%. The highest yield increase due to BioGroe™ inoculation was obtained from 1/2 RRC treatment with yield of 2.47 t ha-1. Actual yield values showed that with BioGroe™ inoculation, the highest yield of 2.47 kg m2 was obtained from 1/2 RRC treatment followed by RRC plots and the lowest harvest of 1.49 kg m2 from the unfertilized plots. Without inoculation, the highest yield was obtained from RRC plots while the lowest was obtained from 1/2 RRC plots. For mustard, all the fertilizer rate- BioGroe™ combinations showed significant increase in yield, with yield increases ranging from 60 to 180%, with the highest increase obtained from 3/4 RRC + BG . The low yield increase in ½ RRC treatment could be attributed to the relatively high yield obtained from the uninoculated ½ RRC plots. Actual yield values however showed that the highest yield among inoculated treatments was obtained from inoculated plots with full RRC applied followed by plots with 3/4 RRC with yields of 2.12 and 1.91 kg m2, respectively. For corn, the efficacy trial results showed that there were no significant differences found between the treatments: RRC, ½ RRC + BioGroe™, ½ RRC + 2x BioGroe™ and RRC + BioGroe™. But significant differences were present in terms of dry matter partitioning and dry matter yield.
ACKNOWLEDGEMENT
We are grateful for the funding support of the Department of Science and Technology-Technology Innovation for Commercialization (DOST-TECHNICOM) and the generosity of our farmer collaborators.
REFERENCE
Arora, N. K., Fatima, T., Mishra, I., and Verma, S. 2020. “Microbe-based inoculants: role in next green revolution,” in Environmental Concerns and Sustainable Development, eds V. Shukla and N. Kumar (Singapore: Springer), 191–245. doi: 10.1007/978-981-13-6358-0_9
Bakhshandeh, E., Gholamhosseini, M., Yaghoubian, Y., Pirdashti, H. 2020. Plant growth promoting microorganisms can improve germination, seedling growth and potassium uptake of soybean under drought and salt stress. Plant Growth Regul. 90: 123–36.
Bashan, Y. 1998. Inoculants of plant growth-promoting bacteria for use in agriculture. Biotechnol Adv 16: 729–770.
Bashan, Y., de-Bashan, L.E., Prabhu, S.R., and Hernandez, J.-P. 2014. Advances in plant growth-promoting bacterial inoculant technology: formulations and practical perspectives (1998-2013). Plant Soil 378: 1–33.
Beneduzi, A., Ambrosini, A., and Passaglia, L.M.P. Plant growth-promoting rhizobacteria (PGPR): Their potential as antagonists and biocontrol agents. 2012. Genet. Mol. Biol. 35 (Suppl. 4): 1044–1051.
Bhattacharyya, P.N., Jha, D.K. 2012. Plant growth-promoting rhizobacteria (PGPR): emergence in agriculture. World J Microbiol Biotechnol. 28: 1327–50.
Dessaux, Y., Grandclément, C. & Faure, D. 2016. Engineering the rhizosphere. Trends in Plant Science 21, 266–278.
Dobbelaere, S., Croonenborghs, A., Thys, A., Ptacek, D., Vanderleyden, J., Dutto, P., et al. 2001. Responses of Agronomically important crops to inoculation with Azospirillum. Aust J Plant Physiol 28: 871–879.
Glick, B.R. 1995. The enhancement of plant growth by free living bacteria. Can. J, microbial. 43:109-117.
Glick, B.R. 2012. Plant growth-promoting bacteria: Mechanisms and applications", Scientifica. doi.org/10.6064/2012/963401
Gómez-Godínez, L.J.; Aguirre-Noyola, J.L.; Martínez-Romero, E.; Arteaga-Garibay, R.I.; Ireta-Moreno, J.; Ruvalcaba-Gómez, J.M. 2023. A look at plant-growth-promoting bacteria. Plants. 12: 1668. https://doi.org/10.3390/plants12081668
Hayat, R., Ali, S., Amara, U., Khalid, R., Ahmed, I. 2010. Soil beneficial bacteria and their role in plant growth promotion: a review. Ann Microbiol. 60: 579–98.
Ji, S.H., Kim, J.S., Lee, C.H., Seo, H.S., Chun, S.H., Oh, J., Choi, E.H., Park, G. 2019. Enhancement of vitality and activity of a plant growth-promoting bacteria (PGPB) by atmospheric pressure non-thermal plasma. Sci Rep 9, 1044. https://doi.org/10.1038/s41598-018-38026-z
Khan, N., Bano, A., Ali, S., and Babar, M.d. A. 2020. Crosstalk amongst phytohormones from planta and PGPR under biotic and abiotic stresses. Plant Growth Regul. 90, 189–203. doi: 10.1007/s10725-020-00571-x
Lopes, M.J.S., Dias-Filho, M.B. and Gurgel, E.S.C. 2021. Successful plant growth-promoting microbes: Inoculation methods and abiotic factors. Front. Sustain. Food Syst. 5:606454. doi: 10.3389/fsufs.2021.606454
Oosten, M. J. V., Pepe, O., Pascale, S., Silletti, S., and Maggio, A. 2017. The role of biostimulants and bioeffectors as alleviators of abiotic stress in crop plants. Chem. Biol. Technol. Agric. 4:5. doi: 10.1186/s40538-017-0089-5
Orhan, E., Esitken, A., Ercisli, S., Turan, M., Sahin, F., 2006. Effects of plant growth promoting Rhizobacteria (PGPR) on yield, growth and nutrient contents in organically growing raspberry. Scientia Horticulturae, Vol. 111, Issue 1, December 2006, pp. 38-43.
Paterno, E.S, M.L.Q. Sison, F.G. Torres, E.S. Garcia, S.M. Pantua and M.E. Orlina. 1996. Development of microbial inoculants for stressed environments. Terminal Report. BIOTECH-UPLB-PCASTRD Project.
Santos, M.S., Nogueira, M.A. & Hungria, M. Microbial inoculants: reviewing the past, discussing the present and previewing an outstanding future for the use of beneficial bacteria in agriculture. AMB Expr 9, 205 (2019). https://doi.org/10.1186/s13568-019-0932-0
Schoenborn, L., Yates, P. S., Grinton, B. E., Hugenholtz, P. and Janssen, P. H. 2004. Liquid serial dilution is inferior to solid media for isolation of cultures representative of the phylum-level diversity of soil bacteria. Applied and Environmental Microbiology. 70 (7): 4363–4366.
Timmusk, S., Behers, L., Muthoni, J., Muraya, A. & Aronsson, A.-C. 2017. Perspectives and challenges of microbial application for crop improvement. Frontiers in Plant Science. 8: 49.
Vejan, P., Abdullah, R., Khadiran, T., Ismail, S. & Nasrulhaq Boyce, A. 2016. Role of plant growth promoting rhizobacteria in agricultural sustainability - a review. Molecules 21, 573.
Woo, S.L., Pepe, O. 2018. Microbial consortia: promising probiotics as plant biostimulants for sustainable agriculture. Front Plant Sci. 9: 1801.