ABSTRACT
Agriculture relies on the interaction between plants and the diverse microbes surrounding them. Pathogenic microbes incite deleterious effects on plant growth, while beneficial microbes enhance plant health. Beneficial microbes have always been a part of research and formulating them into potential biopesticide is a challenge. Among them, Pseudomonas spp., which is a widely studied antagonist, is available in various formulations which delimits the pathogens and enhances the growth and yield attributes of plants. The review appraises types of formulations, application of Pseudomonas-based formulations in mitigating the plant pathogens in different crops, the general mechanisms of action associated with the bacteria, and limitations of the formulations. There exists a scope to extrapolate the laboratory proven results of effective strains of the Pseudomonas spp. Its potential can be explored by developing highly efficient formulations that can maintain the shelf life and quality of the microbial product.
Keywords: Biocontrol, liquid formulation, PGPR, plant diseases, Pseudomonas fluorescens
INTRODUCTION
Biological control of plant diseases focusses on confining pathogen populations, through another beneficial microorganism by its germination, colonization, or spread (Pal and Gardener, 2006; Tariq et al., 2020). Although chemical pesticides result in a quick confinement of diseases and pathogens, their deleterious and hazardous impacts on nature and human health, can’t be ignored. At this point, a shift to a more sound and safer method of sustainable agriculture to feed an ever-increasing population is inevitable (Bejarano and Puopolo, 2020).
Among the multitude of beneficial microbes studied to repress the phytopathogens, Pseudomonas spp. always served as promising antagonists in tackling down the pathogens and enhancing the plant growth, accounting to its PGPR (Plant growth promoting rhizobacteria) activity (Kumar et al., 2017). It is well known for its rhizosphere competence, phytohormones, antimicrobial metabolites, lytic enzymes, HCN (Hydrogen cyanide), siderophores production and its ability to induce systemic resistance (Haas and Defago, 2005; Ganeshan and Kumar, 2007; Kedia et al., 2023). Despite all these, the bioagent if applied directly to the field, it may not be able to corroborate the same results obtained in disease suppression as that under lab conditions. This sheds light on the fact that the antagonists are unable to multiply or even survive in the newly introduced niche where the kingdom of microflora and microfauna are already established. Hence, the introduced cells should be protected and amended to multiply and establish itself successfully in the environment (Bashan et al., 2014). Consequently, these bioagents are formulated in different forms for the development of eco-friendly biopesticides to maintain their shelf life, withstand the environmental stresses, and guard the plants from pathogens. This review chapter mainly concentrates on various bioformulations of Pseudomonas spp. which have been regarded as potential antagonist and PGPR fora very long time (Sharma et al., 2003; Maleki et al., 2010; Tiyagi et al., 2011).
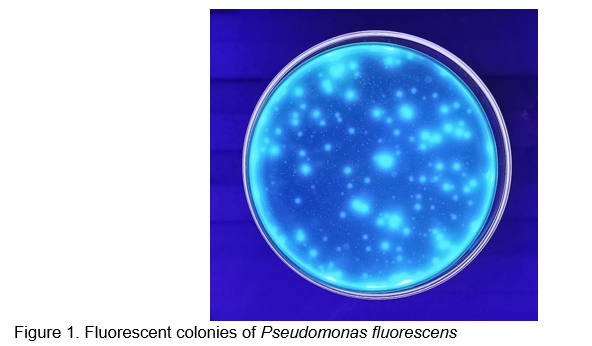
FORMULATIONS AND THEIR TYPES
The primary step in formulating a biopesticide is selecting a potent antagonist. Its efficacy has to be determined and growth requirements/culture conditions have to be verified. Once a bioagent is selected, the next step is to assign a suitable “vehicle” to carry this antagonist, provide a substrate for its growth, and ensure protection (Chaudhary et al., 2020). Additionally, they are amended with various cell protectants, preservatives, emulsifiers, adjuvants, UV protectants, foaming and defoaming agents, etc. (in case of liquid formulations), that aids in preserving the cells for a long duration, to improve its efficiency, and to maintain the quality of the product even during unfavorable conditions (Bejarano and Puopolo, 2020). The role of these amendments has been studied to validate their role in maintaining the quality of the formulated products (Biradar and Santhosh, 2018; Shravani et al., 2019, Bhattacharyya et al., 2020). This process was regarded as “secret art” as quoted by Bashan et al., (2014), where a bioagent verified as a potent antagonist is mass multiplied and grown under controlled conditions and designed into a commercial formulation to be applied to the field. Based on the carriers used, the formulation types vary and here we have reviewed the three major formulation methods, namely powder/granular formulations, liquid formulations, and capsule formulations.
Powder/granular formulations: This utilizes a variety of solid organic and inorganic substrates viz., peat, lignite, charcoal, sugarcane bagasse, saw dust, talcum powder, vermiculite, kaolin, etc. as carrier materials and are commonly used in formulations. A recent study elucidated that, P. fluorescens Ur21 solubilized the phosphorus and released it effectively (1,684.39 mgkg-1; 90% dissolved) at different levels of vermicompost (58.8%), phosphate rock (35.3%) and sulfur (5.8%), for formulating biofertilizer using response surface methodology (Barin et al., 2022). Another study reported the peat-based P. fluorescens (freeze dried), maintained the viability of cells for longer duration (Barman et al., 2022). The storage period of P. fluorescens was compared by formulating it on different carriers and found that, talc was the best carrier when it comes to long term storage (6 months), although, spent mushroom substrate and fly ash could be better alternatives, when it comes to short-term storage (Gade et al., 2014). Recently, a study elucidated that, there was no significant difference between using peat/ talc as carrier material, as the population of bioagent, growth promotion offered by them and rhizosphere competence remained comparable in both the formulations. Even though talc-based formulations are widely used, they suffer from many shortcomings, especially, reduced shelf life, high possibility for contaminations, and reduced field performance (Hegde, 2008). The formulations need to be validated based on the strain of bioagent used (Novinscak and Filion, 2020). Granular formulations are also available on the market, which were easier to handle and store, less dusty, and efficient even under stress conditions (Chaudhary et al., 2020).
Liquid based bioformulations: They are user friendly formulations, where the potential bioagent is formulated with a water/ oil based medium and is found to be more efficient in maintaining the shelf life for more than a year, in contrast to solid carrier-based formulations, which lost the viability after six months. The moisture content required for the organism to survive is maintained in such formulations, and therefore, it can withstand field-related stresses such as desiccation, it has a possibility to be sterilized in bulk, and has stress tolerance too (Singleton et al., 2002; Bashan et al., 2014). The growth rate of P. fluorescens was found to be higher when formulated with Sargassum sp. extracts supplemented with silage and molasses as a liquid biofertilizer (Basmal et al., 2019). Liquid formulation with isotonic phosphate buffer and diluted nutrient broth containing 4% glycerol was proved to be efficient in maintaining the phosphate solubilization ability of P. putida and Pantoea agglomerans (Goljanian-Tabrizi et al., 2016). Coconut water was used as a low-cost medium for development of liquid formulation of P. fluorescens, that maintained population and was found effective in plant growth promotion (Anith et al., 2016; Gopal et al., 2019). Increased survival of P. fluorescens was observed in liquid formulation amended with glycerol (5 mM), as compared to that of solid carrier materials (Karunya and Reetha, 2014). Manikandan and Raghuchander (2014a), detected the antibiotic compounds by TLC and HPLC techniques, produced by P. fluorescens in liquid formulation, which supported its biocontrol efficiency. Both under refrigerated and ambient conditions, the population of P. fluorescens was maintained as high in liquid-based formulation as compared to solid carrier based, during the period of storage (Mohle et al., 2019). Pseudomonas sp. MSSRFD41, which was demonstrated as an effective bioagent against Pyricularia grisea in finger millet, was formulated into a liquid based product in KB broth with amendments, and it was found to maintain the viability until 150 days of storage (Sekar et al., 2018). Oil based formulation of the bioagent was found promising in maintaining the shelf life and in curtailing many pathogens (Peeran et al., 2014; Dobhal, 2020).
Capsule based formulations: Microencapsulation is a method of covering the microbes in a coating and thus protecting the living cells from external stresses, along with a gradual release into the environment (Kim et al., 2012). Usually, hydrogels extracted from the seaweed are used as natural encapsulation materials, but synthetic polymers such as polyacrylamide, polyurethane, polystyrene are also utilized (Dommergues et al., 1979; Cassidy et al. 1996; Schoebitz et al., 2013).The storage capability of this formulation is so high that it was stored over years as documented by Trivedi and Pandey (2008) (3 years of shelf life) and Bashan and Gonzalez (1999) (14 years of shelf life). Several physical, chemical and physiochemical processes are adopted as encapsulation technologies (Schoebitz et al., 2013). When cost and efficacy factors were considered, the resources like broth, water, and sterile medium, which are required in enormous quantities, make it remarkably costly. However, when inserted into the soil, capsules have the ability to function as small-scale bioreactors, which could eliminate the requirement for significant bacterial expansion during fermentation. This might make production less expensive. Additionally, when several capsules are positioned surrounding the rhizosphere, each capsule has the ability to raise cell concentrations on its own. This could improve root colonization and the general efficacy of microbial inoculants in the field (Schoebitz et al., 2013). van Elsas et al. (1992) observed a better survival and wheat root colonization by alginate encapsulated P. fluorescens amended with skim milk and bentonite clay.
APPLICATION OF DIFFERENT FORMULATIONS OF PSEUDOMONAS SPP. IN COMBATING VARIOUS PHYTOPATHOGENS
Pseudomonas spp. have served as effective candidates in curtailing the ill effects of disease causing phytopathogens. It is one of the most researched proteobacteria, owing to its traits such as plant growth promotion and disease suppression (Selvakumar et al., 2015). Keeping the aim of sustainable agriculture ahead, these Pseudomonas spp. which has the potential of producing volatile compounds, lytic enzymes, siderophores, secondary metabolites, hormones, and inducing systemic resistance (Panpatte et al., 2016; Kumar et al., 2017; Mehmood et al., 2023), is formulated in various forms. Here under, we discuss the various formulations of Pseudomonas sp. used in disease suppression in crops.
Cereals and millets
The seed, root, and soil applications of peat-based formulations of P. fluorescens PfALR2 were proved to curb Rhizoctonia solani causing sheath blight of rice, along with enhancing the yield (Rabindran and Vidhyasekaran, 1996). Sheath blight and leaf folder incidence was reduced significantly (62.1% and 47.7-56.1%, respectively), when a combination of two P. fluorescens (PF1 & FP7) strains were applied in rice fields as talc-based formulas (Commare et al., 2002). The reduction in sheath blight using solid carrier-based Pseudomonas was also reported by Mathivanan et al., (2005). The blast incidence in rice was highly reduced when liquid formulation of P. fluorescens (BRM 32111) and B. pyrrocinia (BRM32113),was applied in the field, and served as a breakthrough in biopesticide application (de Sousa Oliveria et al., 2020). The carrier-based powder formulations (talc/peat/kaolin etc.) of P. fluorescens were effective in curbing Xanthomonas oryzae pv. oryzae, (Jambhulkar and Sharma, 2014), dirty panicle of rice (Prathuangwong et al., 2013), rice root-knot nematode (Seenivasan et al., 2012), and sheath blight (Vidhyasekaran and Muthamilan, 1999).
Blast disease of finger millet was suppressed in the presence of Pseudomonas sp. MSSRFD41, in in vitro, green house and field conditions, and was formulated further into a liquid biopesticide for further storage. The bioagent has not only reduced the disease incidence by three-fold, but also enhanced the seedling vigor, germination, and general growth of the crop (Sekar et al., 2018). Another study illustrated that a coconut water-based bioagent formulation was potent enough to reduce the disease severity of leaf blast in finger millet besides improving its growth attributes (Shinde et al., 2017). Liquid formulation of P. fluorescens arrested the sheath blight pathogen in rice significantly (64.5%) when applied as seed and foliar treatment and enhanced the yield (Yadav et al., 2020).
Pulses
Talc and sodium alginate-based formulations of P. fluorescens and Trichoderma harzianum were tested against Fusarium udum in pigeon pea in greenhouse trials. The results revealed that, better seedling emergence and reduced disease incidence was associated with talc-based P. fluorescens treated seedlings than other treatments (Niranjana et al., 2009). Chitin amended talc- based formulation of P. fluorescens was effectively used to inhibit Macrophomina phaseolina in mung bean, under in vitro (44.50%), greenhouse (85.62%) and field conditions (82.13%), apart from improving plant growth (Saravanakumar et al., 2007). Suppression of collar rot of chickpea was evaluated with talc formulation of the bioagent and found it promising in both in vitro and greenhouse (Kumar et al., 2021).
A liquid-based formulation of P. fluorescens was developed and its efficacy was tested against various soil borne pathogens viz., F. oxysporum f. sp. ciceri, S. rolfsii and R. bataticola of chickpea. The results demonstrated that, the formulation not only maintained the shelf life of the antagonist, but also inhibited all the three pathogens in vitro throughout the storage period. The glasshouse studies further revealed that application of the formulation at 10 ml/kg of seeds followed by drenching reduced the incidence of wilt, collar rot and dry root rot (50.01%, 59.48% and 59.09%, respectively), as compared to control (Baby, 2023). The inhibition exhibited by the developed liquid formulation on F. oxysporum f. sp. ciceri and R. bataticola, in vitro, is represented in Figure 2.
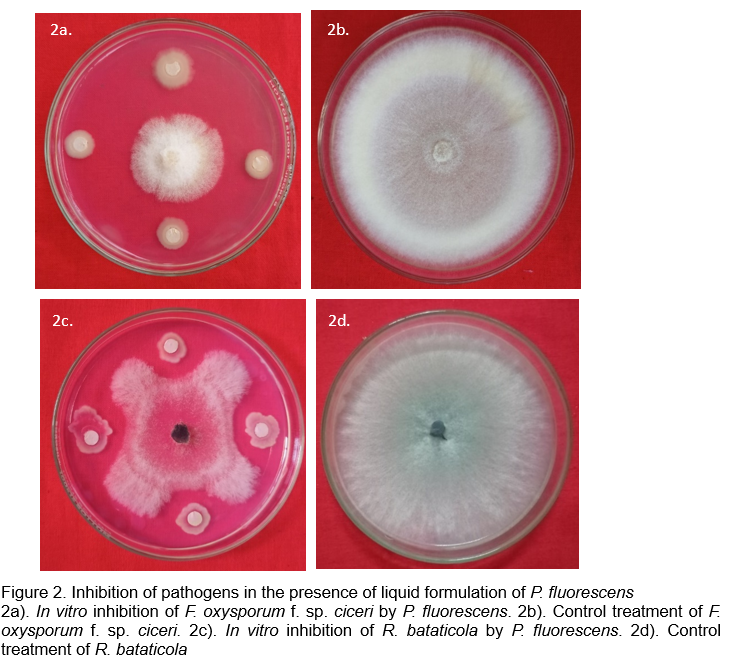
Oilseeds, fiber crops and other cash crops
The effect of P. fluorescens PS1 with sawdust-soil as a carrier was demonstrated in checking the stem blight of Indian mustard, caused by Sclerotinia sclerotiorum. The formulated P. fluorescens was potent enough to impede the hyphal growth of the pathogen by perforation and lysis, even after a storage period of one year, apart from improving the growth traits (Aeron et al., 2011). A similar effect of disease suppression was observed when cotton seeds were treated with bentonite-based P. fluorescens, against R. solani inciting damping off, which further ascertained the role of formulating the biocontrol agents for better efficacy (Ardakani et al., 2010). A notable reduction in groundnut leaf spot and rust diseases was observed by the seed and foliage application of P. fluorescens with talc-based formulation and was found to be more efficient than the fungicidal treatment (Meena et al., 2002).
Red rot of sugarcane incitant, Colletotrichum falcatum was effectively managed in the field when liquid formulation of P. fluorescens was applied at 4 lit/ha and the disease incidence was recorded in a range between 2.34 - 6.45% (1st trial), 2.47 - 5.17% (2nd trial) and 4.23 - 6.51% (3rd trial), as compared to the control treatment (13.72- 28.56%, 9.62-26.44% and 8.83- 20.75%, respectively). In addition, the formulation also reduced early shoot borer and leaf hopper population in the field (Senthil et al., 2011).
Horticultural crops
Pseudomonas spp. have wider application when it comes to horticulture crops. A dry formulation of P. fluorescens EPS62e was developed through lyophilization with lactose as cryoprotectant, and was additionally osmo adapted. This procedure increased the survivability of the antagonistic cells (100%) for a year and enhanced the biocontrol activity against Erwinia amylovora inciting fire blight of pear at low relative humidity (Cabrefiga et al., 2014). Talc-based formulation of P. fluorescens was even found effective in management of tomato spotted wilt virus (TSWV), along with causing increment in the yield (Kandan et al., 2005). The damping off of cucumber caused by Phytophthora capsiciwas effectively suppressed in the presence of seed treatment and pre-plant amendment with irradiated talc and peat formulations of P. fluorescens Pf 9A-14 (85% healthy seedlings) and promoted the growth as well. This was even better than the disease control offered by chemical treatment with metalaxyl (69% healthy seedlings) or with mere cell suspension of the same bacteria (78% healthy seedlings) and even the control (3-6% healthy seedlings) (Khabbaz et al., 2015).The same study elucidated that combined or individual application of irradiated peat formulation of bacteria viz., Pseudomonas fluorescens (Pf 9A-14), Bacillus subtilis (Bs 8B-1) and Pseudomonas sp. (Psp.8D-45) as seed treatment resulted in higher survival of seedlings (25-51%) as compared to that of control (5%). These talc formulations performed well against Pythium damping-off, besides improving the growth parameters of cucumber. Even though the population decreased over time, it was maintained as high as 108 cfu/g at 180th day of storage in irradiated talc and peat formulations (Khabbaz and Abbasi, 2014). The plant growth promotion and disease reduction were also observed in tomato seedlings against Fusarium oxysporum f. sp. radicis-lycopersici, when treated with talc or peat formulations of six bacterial strains, comprising mainly of Pseudomonas spp. (Zhang et al. 2015). Wettable powder formulation of P. syringe and P. fluorescens were also illustrated as efficient for managing post-harvest diseases of fruits (Keswani et al., 2016).The improvement in growth parameters (shoot and root characters) were also recorded in shallots treated with powdered formulation of P. fluorescens and Bacillus coagulans, where the carrier materials used were groundnut shell and water hyacinth (as powder carriers, respectively), and liquid carriers (herbal mixture solution and archae bacterial solution (as liquid carriers respectively) (Yaashikaa et al., 2020). The CaCO3 based formulation of P. fluorescens was found effective in reducing the radial growth of two important soil borne pathogens viz., Fusarium solani and R. solani affecting egg plants, throughout their six months of storage. In addition, the formulation also restrained the egg hatching (5%) and caused the death of J2 stage of Meloidogyne incognita (44%) in egg plants as well (Al-Waily et al., 2018). The field experiment conducted with vermicompost and carboxymethyl cellulose formulation (CVPf) of P. fluorescens in brinjal against bacterial wilt proposed a suppression of the disease up to 83.33%, as it maintained higher cell population of the antagonist, apart from enhancing the yield attributes (Chakravarty and Kalita, 2011; Chakravarty, 2023). Soft rot causing by Pectobacterium carotovorum was effectively managed in Kale by a novel formulation of P. fluorescens SP007S, comprising of sugar sludge, paper sludge and glycerol 1%. The disease incidence was reduced up to 65.2% by the treatment with this formulation and was better than that of cell suspension alone (Nilmat et al., 2023). This underscores the importance of formulating the bioagent, where it sustains the population of antagonist, without losing its efficacy. The efficiency in biocontrol of solid carrier-based formulation of this antagonist bacteria is documented by other researchers also (Moënne‐Loccoz et al., 1999; Soesanto et al., 2019; Rani et al., 2022).
Enhanced suppression of soil borne pathogens like Fusarium oxysporum f. sp. lycopersici and Sclerotium rolfsii, was observed when talc-based P. fluorescens coupled with FYM (farmyard manure) was inoculated in tomato plants. This also induced defense enzymes, adding to its efficiency (Johnson et al., 2022). Liquid formulation of the bacteria restrained Fusarium oxysporum f. sp. Lycopersici (FOL), in tomato plants and the chitinase activity was found to be better in liquid formulation than talc-based formulation (Manikandan and Raguchander, 2015). The reports suggest this formulation even induced defense related enzymes (phenylalanine ammonia lyase (PAL), peroxidase (PO), polyphenol oxidase (PPO), catalase, β-1,3 glucanase and super oxide dismutase (SOD) in tomato plants, and therefore, proved the efficiency of bioformulation (Manikandan and Raguchander, 2014b). Effect of liquid Pseudomonas against this pathogen was further studied widely and have concluded that the shelf life was found up to 210 days. This was efficient in suppressing the wilt pathogen all throughout the storage period and even in glasshouse and field conditions (Paranthaman et al., 2022). Apart from this, liquid formulation of P. fluorescens FP7 remarkably reduced rhizome rot of turmeric (10.18% and13.29% in two field trials) and furthermore, produced an array of defense molecules, promoted growth and improved yield (Prabhukarthikeyan et al., 2018). Glycerol-based formulation of P. fluorescens was proved to be efficient in arresting the grey mold incidence in strawberries, and was found to be even better than chemical treatments in reducing the severity and promoting plant growth (Haggag and El Soud, 2012). Yet another water-in-oil formulation of this bioagent was found to be effective in managing Colletotrichum musae, as it reduced the post-harvest anthracnose incidence and improved the bunch parameters and yield of banana (Peeran et al., 2014). Liquid based P. fluorescens which maintained maximum viability over a year, was also found promising in augmenting the growth of tomato plants apart from suppressing the egg hatching and causing mortality of juveniles of root knot nematode (Meena et al., 2012). Potato cyst nematode was managed successfully by seed treatment with liquid P. fluorescens and Purpureocillium lilacinum, which reduced egg density, multiplication rate, root penetration and number of eggs per cyst, during the field trials (Nagachandrabose, 2020). Similar work to determine on efficacy of liquid-based P. fluorescens in curbing pathogens of horticulture crops also validated its antagonistic potential (Sendhilvel et al., 2007; Peighami-Ashnaei et al., 2009; Manikandan et al., 2010; Pushpa et al., 2014; Selvaraj et al., 2014; Seenivasan, 2017).
Microencapsulated Pseudomonas chlororaphis with alginate-whey protein exhibited reduction in Rhizoctonia solani infection in potato plants (70% reduction), thus ascertaining the importance of encapsulation of bacteria for better efficiency (Fathi et al., 2021). Nano encapsulation of two strains of P. fluorescens (VUPF5 and T17-4) was demonstrated in greenhouse to repress Fusarium solani in potato. The disease reduction offered by capsulated bacterial strains (81% and 75% respectively) was found to be better than that compared to non-capsulated bacteria (76% and 63%, respectively). It also had better shelf life and improved the growth of plants than non-coated bacteria (Pour et al., 2019).
COMMERCIAL FORMULATIONS OF PSEUDOMONAS SPP. a
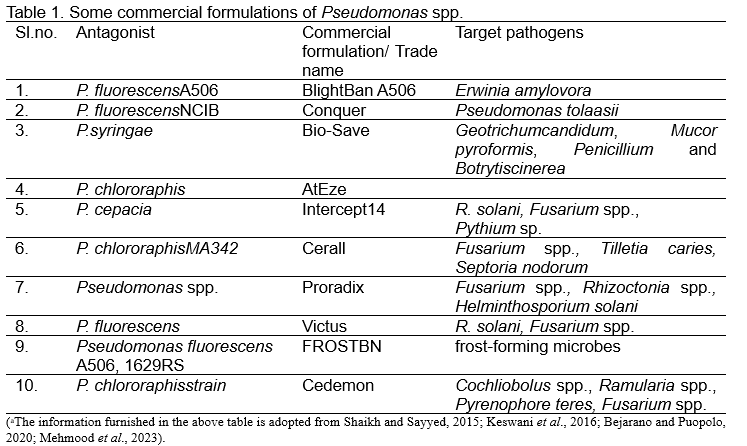
GENERAL MECHANISMS OF ACTION OF PSEUDOMONAS SPP.
The biocontrol agents gain an upper hand over the pathogens as they exert several mechanisms to ensure its own survival. They are regarded as effective competitors, and some important mechanisms are discussed here under.
Competition for nutrients: This is considered as one of the basic mechanisms of action, where the bioagents compete with pathogenic organisms for food and space. Pseudomonas spp. colonize, compete and acquire the nutrients required for their rapid growth, and thereby makes the pathogens thrive in the same environment (Walsh et al., 2001; Panpatte et al., 2016). The iron sequestering compound, siderophore, produced by Pseudomonas spp., chelate the Fe3+ ions in the soil and thereby limit the pathogens from acquiring them. This provides an extra advantage for Pseudomonas to survive in the soil rather than pathogens which require iron for its growth (Loper and Buyer, 1991). The role of siderophores is widely demonstrated in disease suppression (Solans et al., 2016; Arya et al., 2018, Jadav, 2021; Baby, 2023).
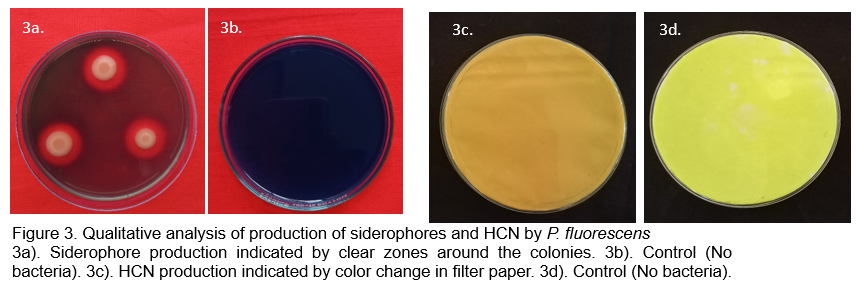
Production of HCN and antibiotics: The secondary metabolite HCN, produced by Pseudomonas spp. serves as a volatile inhibitory toxic compound that delimits the growth of pathogen (Voisard et al., 1989; Jayaprakashvel et al., 2011; Jadav, 2021; Baby, 2023). Antibiotics play key roles in pathogen inhibition and have a broad-spectrum activity. Pseudomonas is known to synthesize an array of antibiotics viz., 2,4-diacetylphloroglucinol (2,4-DAPG), phenazine, pyoluteorin, and pyrrolnitrin, pyochelin, etc. that aid in inhibition of pathogen growth (Voisard et al., 1989; Reddy et al., 2009; Compant et al. 2010; Castaldi et al., 2021; Trejo- Raya et al., 2021).
Production of lytic enzymes and induction of ISR: The hydrolytic enzymes viz., cellulases, chitinases, glucanses, proteases, peroxidases, phenylalanine ammonia lyase etc., synthesized by the bacteria, results in cell wall degradation and disruption of metabolic functions, finally leading to disease suppression (Rai, 2011; Suresh et al., 2022). It is also known to possess ACC-deaminase activity that hinder the ethylene levels and thus, disease reduction (Gamalero et al., 2017). These beneficial microbes have yet another capability to induce the systematic resistance in plants, following their application. This paves the way to the enhancement of plant defense against deleterious pathogens (van Loon and Bakker, 2006; Singh et al., 2015; Kannojia et al., 2019).
LIMITATIONS OF THE FORMULATIONS
Even though there are ample reports on the antagonistic ability of highly efficient strains of biocontrol agents, the development of their effective formulation is still not yet fully achieved. The biopesticides are always questioned for their field efficiency, even though they exhibit potential inhibition in vitro (Shaikh and Sayyed, 2015; Keswani et al., 2016). One of the reasons behind this is the reduction in population of active cells, and therefore the performance capability decreases. It even has to adapt to the new environment which is totally heterogeneous in nature and has to interact with already established microflora. This can affect the field performance significantly (Glick et al., 1999). Shelf life is another constraint in developing formulations. The strain of antagonist used, carriers, production methodologies adopted, packaging and transport influences shelf life (Keswani et al., 2016). During storage, there is a possibility of contaminations with undesired microbes, that will deplete the quality and efficiency of the product (Arora et al. 2010). There are the general factors pulling backward the biopesticide industry from growth.
CONCLUSION
Biological control of plant diseases has always been a part of research, when the world seeks out for better alternatives for hazardous chemicals. Pseudomonas spp. served as an efficient antagonist and plant growth promoter, improving the plant health. The review has focused on the bioformulations of this bacterium, its efficacy in tackling the pathogens of major crops, and the mechanisms of action. The efficacy of biopesticides will usually last for about 15 to 20 days while interaction with the targeted pathogens under field conditions. There exists a wide scope to be explored in formulating an efficient biopesticide, which exhibits its maximum potential in field that can benefit the farmers in terms of reducing the cost of cultivation with due emphasis on safeguarding the environment and for sustainable yields.
REFERENCES
Aeron, A., R. C. Dubey, D. K. Maheshwari, P. K. Pandey, V. K. Bajpai andS. C. Kang. 2011. Multifarious activity of bioformulatedPseudomonas fluorescens PS1 and biocontrol of Sclerotinia sclerotiorum in Indian rapeseed (Brassica campestris L.). Eur. J. Plant Pathol. 131: 81-93.
Al-Waily, D.S., L. A. Al-Saad and S. S. Al-Dery. 2018. Formulation of Pseudomonas fluorescens as a biopesticide against soil borne root pathogens. Iraqi J. Agric.l Sci. 49(2):235-242.
Anith K. S., A. S. Vyshakhi,A. Viswananthan, S. Varkey, and S. Aswini. 2016. Population dynamics and efficiency of coconut water based liquid formulation of Pseudomonas fluorescens AMB-8. J. Trop. Agri. 54(2): 184-189.
Arora, N. K., E. Khare andD. K. Maheshwari. 2010. Plant growthpromoting rhizobacteria: constraints inbioformulation, commercialization, and futurestrategies. In: Plant growth andhealth promoting bacteria (Ed. Maheshwari, D. K.), Springer, Berlin, 97–116.
Arya, N., A. Rana, A. Rajwar, M. Sahgal and A. K. Sharma. 2018. Biocontrol efficacy of siderophore producing indigenous Pseudomonas strains against Fusarium wilt in tomato. Natl. Acad. Sci. Lett.41: 133–136.
Baby, A. S. 2023. Standardisation and evaluation of liquid formulations of Pseudomonas fluorescens (Flugge) Migula against soil borne fungal diseases of chickpea. M.Sc. (Agri.) Thesis, University of Agricultural Sciences, Dharwad, Karnataka, India.
Barin, M., F. Asadzadeh, M. Hosseini, E. C. Hammer, R. R.Vetukuri, R. Vahedi. 2022. Optimization of biofertilizer formulation for phosphorus solubilizing by Pseudomonas fluorescens Ur21 via response surface methodology. Processes. 10: 650.
Barman, N.C., M.S Sarker, M. Ahmed, Z. Ahmedand S. Ramachandran. 2023. Optimizing shelf-life of Pseudomonas fluorescens after freeze drying. In:Pseudomonas aeruginosa - new perspectives and applications [working title] (Eds.Dharwesh O. M. and I. Matter). IntechOpen. http://dx.doi.org/10.5772/intechopen.108034.
Bashan, Y. and L. E. Gonzalez. 1999. Long-term survival of the plant-growthpromotingbacteria Azospirillum brasilense and Pseudomonasfluorescens in dry alginate inoculant. Appl.Microbiol.Biotechnol. 51: 262–266.
Bashan, Y., L.E.de-Bashan, S.R. Prabhu, J. Hernandez. 2014. Advances in plant growth-promoting bacterial inoculant technology: formulations and practical perspectives (1998–2013). Plant Soil. 378: 1–33.
Basmal, J., M. E.Aribowo, Nurhayatiand R.Kusumawati. 2019. Growth rate of Pseudomonas fluorescens in liquid fertilizer from brown seaweed (Sargassum sp.) extracts. IOP Conf. Ser.: Earth Environ. Sci. 383: 012027.
Bejarano,A. andG. Puopolo. 2020. Bioformulation of microbial biocontrol agents for a sustainable agriculture. In: How research can stimulate the development of commercial biological control against plant diseasesprogress in biological control (Vol 21) (Eds. A. De Cal, P. Melgarejo and N. Magan), Springer, Cham.
Berninger, T., Ó.González López, A. Bejarano, C. Preininger and A. Sessitsch, 2018. Maintenance and assessment of cell viability in formulation of non‐sporulating bacterial inoculants. Microb.Biotechnol. 11(2): 277-301.
Bhattacharyya, C., R. Roy, P. Tribedi, A. Ghosh and A. Ghosh. 2020. Biofertilizersas substitute to commercial agrochemicals. In: Agrochemicals detection, treatment and remediation (Eds.Majeti N.,P. Vara),Butterworth-Heinemann, 263–290.
Biradar, P. B. J. and G. P. Santhosh. 2018. Cell protectants, adjuvants, surfactant and preservative and their role in increasing the shelf life of liquid inoculant formulations of Pseudomonas fluorescens. Int. J. Pure Appl. Biosci. 6(4): 116-122.
Cabrefiga, J., J. Francés, E. Montesinosand A. Bonaterra. 2014. Improvement of a dry formulation of Pseudomonas fluorescens EPS62e for fire blight disease biocontrol by combination of culture osmoadaptation with a freeze‐drying lyoprotectant. J.Appl.Microbiol. 117(4): 1122-1131.
Cassidy, M. B., H. Lee and J. T.Trevors. 1996. Environmental applications of immobilized microbial cells: a review. J. Ind. Microbiol. 16: 79–101.
Castaldi, S., M. Masi,F. Sautua, A. Cimmino, R. Isticato, M. Carmona, A. Tuzi and A. Evidente. 2021.Pseudomonas fluorescens showing antifungal activity against Macrophomina phaseolina, a severe pathogenic fungus of soybean, produces phenazine as the main active metabolite. Biomolecules. 11(11): 1-13.
Chakravarty, G. 2023. Management of bacterial wilt disease of brinjal by P. fluorescens based bioformulation: experimental investigation. In: Emerging Issues in Agricultural Sciences (Vol. 2) (Ed. Al-Naggar A. M.), B P International, 130-150.
Chakravarty, G. and M. C. Kalita. 2011. Comparative evaluation of organic formulations of Pseudomonas fluorescens based biopesticides and their application in the management of bacterial wilt of brinjal (Solanum melongena L.). Afr. J.Biotechnol. 10(37): 7174-7182.
Chaudhary, T., M. Dixit, R. Gera, A. K. Shukla,A. Prakash, G.Guptaand P. Shukla. 2020. Techniques for improving formulations of bioinoculants. 3 Biotech. 10: 199.
Commare, R.R., R. Nandakumar, A. Kandan, S. Suresh, M. Bharathi, T. Raguchanderand R. Samiyappan. 2002. Pseudomonas fluorescens based bio-formulation for the management of sheath blight disease and leaffolder insect in rice. Crop Prot. 21(8): 671-677.
Compant, S., C. Clément and A. Sessitsch. 2010. Plant growth-promotingbacteria in the rhizo and endosphere of plants: their role,colonization, mechanisms involved and prospects for utilization. Soil Biol.Biochem. 42:669–678.
de Sousa Oliveira, M.I., A. A. Chaibub, T. P. Sousa, M.V.C.B.Cortes, A. C. A. de Souza, E. C. da Conceiçãoand M.C. C. de Filippi. 2020. Formulations of Pseudomonas fluorescens and Burkholderia pyrrocinia control rice blast of upland rice cultivated under no-tillage system. Biol.Control, 144: 104153.
Dobhal, A. 2020. Development of liquid formulation of Pseudomonas fluorescens (Flugge) Migula and their efficacy against spot blotch of wheat. M.Sc. (Agri.) Thesis, University of Agricultural Sciences, Dharwad, Karnataka, India.
Dommergues, Y. R., H. G. Diem and C.Divies. 1979. PolyacrylamideentrappedRhizobium as an inoculant for legumes. Appl. Environ.Microbiol. 37:779–781.
Fathi, F., R. Saberi Riseh, P. Khodaygan,S. Hosseini,Y. A. Skorik. 2021. Microencapsulation of a Pseudomonas Strain (VUPF506) in Alginate–Whey Protein–Carbon Nanotubes and Next-Generation Sequencing identification of this strain. Polymers.13(23): 4269. https://doi.org/10.3390/polym13234269
Gade, R.M., B. H. Chaithanyaand K. C. Khurade. 2014. A comparative study of different carriers for shelf life of Pseudomonas fluorescens. The Bioscan. 9(1): 287-290.
Gamalero, E.,C. Marzachi, L. Galetto, F. Veratti, N. Massa, E.Bona, G. Novello, B. Glick, S. Ali, S. Cantamessa, D’Agostino, G. and Berta, G. 2017. An 1-Aminocyclopropane-1-carboxylate (ACC) deaminase-expressing endophyteincreases plant resistance to FlavescenceDoree phytoplasma infection. Plant Biosyst. 151(2): 331−340.
Ganeshan, G. and M. A. Kumar. 2007.Pseudomonas fluorescens, a potentialbacterial antagonist to control plant diseases. J. Plant Interact. 1(3): 123-134.
Glick, B. R., C. L. Patten, G. Holguin andD. M. Penrose. 1999. Biochemical and genetic mechanisms used by plantgrowth promoting bacteria. Imperial College Press, London
Gopal, M., A. Gupta, K. S. Hameed, R. Chandramohanan and G. V. Thomas. 2019. A simple, quick and contamination-free method for mass-multiplication of plant-beneficial microbes by small and marginal farmers using coconut water and rice gruel medium. Indian J. Agric. Sci. 89(2): 339-343.
Haas, D. and G. Defago. 2005. Biologicalcontrol of soil-borne pathogens byfluorescent Pseudomonads. Nat. Rev.Microbiol. 3: 307-319.
Haggag, W. M. and M. A. El Soud. 2012. Production and optimization of Pseudomonas fluorescens biomass and metabolites for biocontrol of strawberry grey mould. Am. J. Plant Sci. 3(7): 836-845.
Hegde, S. V. 2008. Liquid bio-fertilizers in Indian agriculture. Bio-fertilizer newsletter, 12: 17-22.
Jadav, P. C. 2021. Evaluation of microbial consortia for the management of foot rot of wheat caused by Sclerotium rolfsii Sacc. M. Sc. (Agri.) Thesis, University of Agricultural Sciences, Dharwad, Karnataka, India.
Jambhulkar, P.P. and Sharma, P. 2014. Development of bioformulation and delivery system of Pseudomonas fluorescens against bacterial leaf blight of rice (Xanthomonas oryzaepv. oryzae). J.Environ.Biol. 35(5): 843-849.
Jayaprakashvel, M., R. Muthezhilan, R. Srinivasan, A. Hussain, S. Gobalakrishnan, J. Bhagat, C. Karthigeyan and R. Muthulakshmi. 2010. Hydrogen cyanide mediated biocontrol potential of Pseudomonas sp. AMET1055 isolated from the rhizosphere of coastal sand dune vegetation. J Advanced Biotech. 9(10): 39-42.
Johnson, I., B. Sreenayana, V.P. Suruthi, R.Manikandan, R.Ramjegathesh and M. Karthikeyan. 2022. Rhizosphere population dynamics and biocontrol potential of Pseudomonas fluorescens Pf1 against Wilt and collar rot pathogens in tomato. Pharma Innovat. 11(5): 1042-1051.
Kandan, A., M. Ramiah, V. J. Vasanthi, R. Radjacommare, R. Nandakumar, A. Ramanathanand Samiyappan, R. 2005. Use of Pseudomonas fluorescens-based formulations for management of tomato spotted wilt virus (TSWV) and enhanced yield in tomato. Biocontrol Sci.Technol. 15(6): 553-569.
Kannojia, P.,K. K. Choudhary, A. K. Srivastava andA. K. Singh. (2019). PGPR bioelicitors: induced systemic resistance (ISR) and proteomicperspective on biocontrol. In: PGPR amelioration in sustainableagriculture (Eds. Singh, A. K., A. Kumar andP. K. Singh), Elsevier, 67−84.
Karunya, S.K. andD. Reetha. 2014. Survival of saline tolerant PGPR in different carriers and liquid formulations.Int. J. Adv. Res. Biol.Sci. 1(2): 179-183.
Kedia, V. K., M. Sharma and K. Shantanu. 2023. Pseudomonas sp.: A promising biocontrol agent against selected phytopathogens. J. Mountain Res. 18(1): 245-255.
Keswani, C., K. Bisen, V. Singh, B. K. Sarma and H. B. Singh. 2016. Formulation technology of biocontrol agents: present status and future prospects. In: Bioformulations: for sustainable agriculture (Eds. Arora N. K., S. M. Raff, R. Balestrini),Springer, Varanasi,35–52.
Khabbaz, S.E. and P.A. Abbasi. 2014. Isolation, characterization, and formulation of antagonistic bacteria for the management of seedlings damping-off and root rot disease of cucumber. Can.J. Microbiol. 60(1): 25-33.
Khabbaz, S.E., L. Zhang, L. A. Cáceres, M. Sumarah, A. Wang and P. A. Abbasi. 2015. Characterisation of antagonistic Bacillus and Pseudomonas strains for biocontrol potential and suppression of damping‐off and root rot diseases. Ann. Appl. Biol. 166(3): 456-471.
Kim, I. Y., P. L. Pusey, Y. Zhao, S. S. Korban, H. Choi and K. K. Kim. 2012. Controlled release of Pantoeaagglomerans E325 for biocontrol of fire blight disease of apple. J. Control Release 161: 9–15.
Kumar, A., H. Verma, V. K. Singh, P. P. Singh, S. K. Singh, W. A. Ansari, A. Yadav, P. K. Singhand K. D. Pandey. 2017. Role of Pseudomonas sp. in sustainable agriculture and disease management. In: Agriculturally important microbes for sustainable agriculture (Vol 2) Applications in crop production and protection(Eds. Meena, V., P. Mishra, J. Bisht, A.Pattanayak), 195-215.
Kumar, R., P. Bhargava and D. Sinha. 2021.Pseudomonas based formulation for biocontrol of Sclerotium rolfsii causing collar rot of chickpea. Biological forum, 13(4): 1100-1103.
Loper, J. E. andJ. S. Buyer. 1991. Siderophores in microbialinteractions on plant surfaces. Mol Plant-Microbe Interact. 4:5–13.
Maleki, M., S. Mostafaee, L.Mokhtarnejad and M. Farzaneh. 2010. Characterization ofPseudomonas fluorescens Strain CV6 isolated from cucumber rhizosphere in varamin as a potential biocontrol agent. Aust.J Crop Sci. 4(9): 676-683.
Manikandan, R. and Raguchander, T. 2015. Pseudomonas fluorescens (Pf1) mediated chitinolytic activity in tomato plants against Fusarium oxysporum f. sp. lycopersici. Afr. J. Microbiol. Res. 9: 1331-1337.
Manikandan, R. andT. Raguchander, T. (2014a). Detection of antibiotic compounds from liquid formulation of Pseudomonas fluorescens (Pf1) by TLC and HPLC analysis. Journal of Pure and Applied Microbiology. 8. 4025-4030.
Manikandan, R. and T. Raguchander.(2014b). Fusarium oxysporum f. sp. lycopersici retardation through induction of defensive response in tomato plants using a liquid formulation of Pseudomonas fluorescens (Pf1). Eur J Plant Pathol. 140: 469–480.
Manikandan, R., D. Saravanakumar, L. Rajendran, T. Raguchanderand R. Samiyappan. 2010. Standardization of liquid formulation of Pseudomonas fluorescens Pf1 for its efficacy against Fusarium wilt of tomato. Biological control. 54(2): 83-89.
Mathivanan, N., V. R. Prabavathy and V. R. Vijayanandraj. 2005. Application of talc formulations of Pseudomonas fluorescensMigula and Trichoderma viride Pers. ex SF Gray decrease the sheath blight disease and enhance the plant growth and yield in rice. J Phytopathol. 153(11‐12): 697-701.
Meena, K.S., E. I. Jonathanand P. G. Kavitha. 2012. Viability of Pseudomonas fluorescens in liquid formulation and its effect on plant growth promotion and inhibition of root knot nematode, Meloidogyne incognita. Madras Agric. J. 99(10-12): 850-853.
Meena, B., R. Radhajeyalakshmi, T. Marimuthu, P. Vidhyasekaran and R. Velazhahan. 2002. Biological control of groundnut late leaf spot and rust by seed and foliar applications of a powder formulation of Pseudomonas fluorescens, Biocontrol Sci Technol. 12(2): 195-204.
Mehmood, N., M. Saeed, S. Zafarullah, S. Hyder, Z. F. Rizvi, A. S. Gondal, N. Jamil, R. Iqbal, B. Ali, S. Ercisli and M. Kupe. 2023. Multifaceted impacts of plant-beneficial Pseudomonas spp. in managing various plant diseases and crop yield improvement. ACS omega, 8(25): 22296-22315.
Moënne‐Loccoz, Y., M. Naughton, P. Higgins, J. Powell, B. O'connorand F. O'gara. 1999. Effect of inoculum preparation and formulation on survival and biocontrol efficacy of Pseudomonas fluorescens F113. J. Appl. Microbiol. 86(1): 108-116.
Mohle,K. K., R. K. S. Tiwari, M. Tondeyand K, Kumar. 2019. Shelf life study of Pseudomonas fluorescens in talc based carrier and liquid formulations. Int. J. Chem. Studies. 7(6): 922-925.
Nagachandrabose, S. 2020. Management of potato cyst nematodes using liquid bioformulations of Pseudomonas fluorescens, Purpureocilliumlilacinum and Trichoderma viride. Potato Research, 63(4), pp.479-496.
Nilmat, A., W. Thepbandit, W.Chuaboon and D. Athinuwat. 2023. Pseudomonas fluorescens SP007S formulations in controlling soft rot disease and promoting growth in kale. Agronomy, 13(7): 1856.
Niranjana, S. R., S. Lalitha and P. Hariprasad. 2009. Mass multiplication and formulations of biocontrol agents for use against fusarium wilt of pigeonpea through seed treatment, Int J Pest Manag. 55(4): 317-324.
Novinscak, A. and M. Filion. 2020. Long term comparison of talc-and peat-based phytobeneficialPseudomonas fluorescens and Pseudomonas synxantha bioformulations for promoting plant growth. Front. Sustain. Food Syst. 4: 602911.
Pal, K. K. and B. M. Gardener. 2006. Biological control of plant pathogens. Plant Health Instr. 1–25.
Panpatte, D. G., Y. K. Jhala, H. N. Shela and R. V. Vyas. 2016. Pseudomonas fluorescens: A Promising Biocontrol Agent and PGPR for Sustainable Agriculture. In: Microbial inoculants in sustainable agricultural productivity (Eds. Singh, D. P., H. B. Singh, R. Prabha),Springer, New Delhi, 257–270.
Peeran, M.F., N. Krishnan, P. R. Thangamani, K. Gandhi, T. Raguchander, and P. Kuppusamy. 2014. Development and evaluation of water-in-oil formulation of Pseudomonas fluorescens (FP7) against Colletotrichum musae incitant of anthracnose disease in banana. Eur. J. Plant Pathol. 138: 167-180.
Peighami-Ashnaei, S., A. Sharifi-Tehrani, M. Ahmadzadeh and B. Keivan. 2009. Interaction of different media on production and biocontrol efficacy of Pseudomonasfluorescens P-35 and Bacillus subtilis B-3 against grey mould of apple. Journal of Plant Pathology, 91(1): 65-70.
Pour, M.M., R. Saberi-Riseh, R. Mohammadinejad and A. Hosseini. 2019. Investigating the formulation of alginate-gelatin encapsulated Pseudomonas fluorescens (VUPF5 and T17-4 strains) for controlling Fusarium solani on potato. Int.J. Biol.Macromol. 133: 603-613.
Prabhukarthikeyan, S.R., U. Keerthanaand T. Raguchander. 2018. Antibiotic-producing Pseudomonas fluorescens mediates rhizome rot disease resistance and promotes plant growth in turmeric plants. Microbiol. Res. 210: 65-73.
Prathuangwong, S., D. Athinuwat, W.Chuaboon, T.Chatnaparat and N. Buensanteai. 2013. Bioformulation Pseudomonas fluorescens SP007s against dirty panicle disease of rice. Afr. J Microbiol. Res. 7(47): 5274-5283.
Pushpa, A., P. C. Subhash, M. A. H. Reddy. 2014. Development of liquid formulation for the dual purpose of crop protection and production. J. Environ. Res. Dev. 8:378–383.
Rabindran, R. andP. Vidhyasekaran. 1996. Development of a formulation of Pseudomonas fluorescens PfALR2 for management of rice sheath blight. Crop Prot. 15(8): 715-721.
Rai, V. 2011. Screening of Pseudomonas fluorescens isolates forbiological control of Macrophomina phaseolina root-rot of safflower.Afr. J. Agric. Res.6(29): 6256−6266.
Rani, P., M. Singh, H. Prashadand M. Sharma. 2022. Evaluation of bacterial formulations as potential biocontrol agents against the southern root-knot nematode, Meloidogyne incognita. Egypt. J. Biol. Pest Control. 32(1): 29.
Reddy, B. P., M. S. Reddy and K. V. K. Kumar. 2009. Characterization of antifungal metabolites of Pseudomonas fluorescens and their effect on mycelial growth of Magnaporthe grisea and Rhizoctonia solani. Int. J. Chem. Tech. Res. 1(4): 1490-1493.
Saravanakumar, D., S. Harish, M. Loganathan, R. Vivekananthan, L. Rajendran, T. Raguchander and R. Samiyappan. 2007.Rhizobacterial bioformulation for the effective management of Macrophominaroot rot in mungbean. Arch.Phytopathol. Plant Prot. 40(5): 323–337.
Schoebitz, M.,M. López andA. Roldán.2013. Bioencapsulation of microbial inoculants for bettersoil–plant fertilization. A review. Agron. Sustain. Dev. 33(4): 751-765.
Seenivasan, N. 2017. Combined application of Pseudomonas fluorescens and Purpureocilliumlilacinum liquid formulations to manage Globoderaspp on potato. J. Crop Prot. 6(4): 529-537.
Seenivasan, N., P. M. M. David, P. Vivekanandanand R. Samiyappan. 2012. Biological control of rice root-knot nematode, Meloidogyne graminicola through mixture of Pseudomonas fluorescens strains. Biocontrol Sci. Technol. 22(6): 611-632.
Sekar, J., K. Raju, P. Duraisamy and V. P. Ramalingam. 2018. Potential of finger millet indigenous rhizobacterium Pseudomonas sp. MSSRFD41 in blast disease management—growth promotion and compatibility with the resident rhizomicrobiome. Front. Microbiol. 9:1029.
Selvakumar, G. P., G. H. Panneerselvam, A. N. Bindu and Ganeshamurthy. 2015. Pseudomonads: plant growth promotion and beyond. Plant microbes symbiosis: applied facets (Ed. Arora, N.K.), Springer India, 193–208.
Selvaraj, S., P. Ganeshamoorthi,T. Anand, T. Raghuchander, N. Seenivasan and R. Samiyappan. 2014. Evaluation of a liquid formulation of Pseudomonas fluorescens against Fusarium oxysporum f.sp. cubense and Helicotylenchusmulticinctus in banana plantation. BioControl. 59: 345-359.
Sendhilvel, V., T. Marimuthuand R. Samiappan. 2007. Talc-based formulation of Pseudomonas fluorescens-induced defense genes against powdery mildew of grapevine. Arch.Phytopathol. Plant Prot. 40(2): 81-89.
Senthil, R., S. Selvaraj, T. Anand, T. Raguchanderand R. Samiyappan. 2011. Efficacy of liquid Pseudomonas fluorescens (Pf1) against sugarcane red rot, caused by Colletotrichum falcatum, under field conditions. Int.Sugar J. 113(1356): 888.
Shaikh, S.andR. Sayyed. 2015. Role of plant growth-promoting rhizobacteria and their formulation in biocontrol of plant diseases. In book: Plant microbes symbiosis: applied facets(Ed. Arora, N.K.),Springer India, 337-351.
Sharma, A., B.N.Johri, A.K. Sharma, and B. R. Glick. 2003. Plant growth-promoting bacterium Pseudomonas sp. strain GRP3 influences iron acquisition in mung bean (Vigna radiata L. Wilzeck). Soil Biol.Biochem. 35(7): 887-894.
Shinde, M. S., A. N. Sabalpara and A. Deshmukh. 2017. Mass multiplication and shelf-life study of liquid formulation of Pseudomonas sp. & their effect on PGPR activity and blast disease of finger millet. Green Farming Int. J. 8: 214-217.
Shravani, K., S.Triveni, P. C. Latha and K. D. Chari. 2019. Evaluation of shelf life and quality of carrier and liquid based biofertilizers. Int. J.Microbiol. Res. 11(6): 1598-1601.
Singh, N. P., R. K. Singh, V. S. Meena and R. K. Meena. 2015. Can we use maize (Zea mays) rhizobacteria as plant growth promoter? Vegetos. 28(1): 86-99.
Singleton, P., H. Keyser, E. Sande. 2002. Development and evaluation of liquid inoculants. In: Inoculants and nitrogen fixation of legumes in Vietnam (Ed. Herridge, D.), ACIAR: Canberra, Australia, 109e, 52-66.
Soesanto, L., E. Mugiastuti and K. Khoeruriza. 2019. Granular formulation test of Pseudomonas fluorescens P60 for controling bacterial wilt (Ralstonia solanacearum) of tomato in planta. AGRIVITA. 41(3): 513-523.
Solans, M., J. M. Scervino, M. I.Messuti, G. Vobis and L. G. Wall. 2016. Potential biocontrol actinobacteria: rhizospheric isolates from the Argentine pampas lowlands legumes. J. Basic Microbiol. 54: 585-596.
Suresh, P.,V. Shanmugaiah, R. Rajagopal,K. Muthusamy and V.Ramamoorthy. 2022. Pseudomonas fluorescens VSMKU3054 mediatedinduced systemic resistance in tomato against Ralstonia solanacearum.Physiol. Mol. Plant Pathol.119: 101836.
Tariq, M.,A. Khan, M. Asif, F. Khan, T. Ansari, M.Shariq andM. A. Siddiqui. 2020. Biological control: a sustainable and practical approachfor plant disease management. Acta Agric. Scand. B. Soil Plant Sci. 70:6, 507-524.
Tiyagi, S.A., I. Mahmood, Z. Khan and H. Ahmad. 2011. Biological control of soil-pathogenic nematodes infecting mungbean using Pseudomonas fluorescens. Arch.Phytopathol. Plant Prot. 44(18): 1770-1778.
Trejo-Raya, A.B., V. M.Rodríguez-Romero, S. Bautista-Baños, F. R. Quiroz-Figueroa,R. Villanueva-Arce andE. Durán-Páramo. 2021. Effective in vitrocontrol of two phytopathogens ofagricultural interest using cell-freeextracts of Pseudomonas fluorescensand chitosan. Molecules. 26: 6359.
Trivedi, P. andA. Pandey. 2008. Recovery of plant growth-promotingrhizobacteria from sodium alginate beads after 3 years following storage at 4 degrees C. J. Ind. Microbiol. Biotechnol. 35(3): 205–209.
van Elsas, J. D., J. T. Trevors, D. Jain, A. C. Wolters, C. E. Heijnen and L. S. van Overbeek. 1992. Survival of, and root colonization by, alginate-encapsulated Pseudomonas fluorescens cells following introduction into soil. Biol Fertil Soils. 14: 14–22.
Van Loon, L. C. and P. A. H. M. Bakker. 2006. Root-associatedbacteria inducing systemic resistance. In:Plant-associated bacteria (Eds. Gnanamanickam, S. S.), Springer, Dordrecht, 269–316
Voisard, C., C. Keel, D. Haas and G.Defago. 1989. Cyanideproduction by Pseudomonas fluorescens helps suppressblack root rot of tobacco under gnotobiotic conditions. EMBO J. 8:351–358.
Walsh, U.F., J. P. Morrissey and F. O’Gara. 2001.Pseudomonasfor biocontrol of phytopathogens: from functionalgenomics to commercial exploitation. Curr.Opin.Biotechnol. 12: 289–295.
Yaashikaa, P.R., P. S. Kumar, S. Varjani, S. Tamilselvi and A. Saravanan. 2020. Formulation and combinatorial effect of Pseudomonas fluorescens and Bacillus coagulans as biocontrol agents. Biocatal. Agric.Biotechnol. 30: 101868.
Zhang, L., S. E. Khabbaz, A. Wang, H. Li and P. A. Abbasi. 2015. Detection and characterization of broad‐spectrum antipathogen activity of novel rhizobacterial isolates and suppression of Fusarium crown and root rot disease of tomato. J Appl.Microbiol. 118(3): 685-703.
Efficacy of Formulations of Pseudomonas spp.- A Potential Biopesticide in Combating Plant Diseases
ABSTRACT
Agriculture relies on the interaction between plants and the diverse microbes surrounding them. Pathogenic microbes incite deleterious effects on plant growth, while beneficial microbes enhance plant health. Beneficial microbes have always been a part of research and formulating them into potential biopesticide is a challenge. Among them, Pseudomonas spp., which is a widely studied antagonist, is available in various formulations which delimits the pathogens and enhances the growth and yield attributes of plants. The review appraises types of formulations, application of Pseudomonas-based formulations in mitigating the plant pathogens in different crops, the general mechanisms of action associated with the bacteria, and limitations of the formulations. There exists a scope to extrapolate the laboratory proven results of effective strains of the Pseudomonas spp. Its potential can be explored by developing highly efficient formulations that can maintain the shelf life and quality of the microbial product.
Keywords: Biocontrol, liquid formulation, PGPR, plant diseases, Pseudomonas fluorescens
INTRODUCTION
Biological control of plant diseases focusses on confining pathogen populations, through another beneficial microorganism by its germination, colonization, or spread (Pal and Gardener, 2006; Tariq et al., 2020). Although chemical pesticides result in a quick confinement of diseases and pathogens, their deleterious and hazardous impacts on nature and human health, can’t be ignored. At this point, a shift to a more sound and safer method of sustainable agriculture to feed an ever-increasing population is inevitable (Bejarano and Puopolo, 2020).
Among the multitude of beneficial microbes studied to repress the phytopathogens, Pseudomonas spp. always served as promising antagonists in tackling down the pathogens and enhancing the plant growth, accounting to its PGPR (Plant growth promoting rhizobacteria) activity (Kumar et al., 2017). It is well known for its rhizosphere competence, phytohormones, antimicrobial metabolites, lytic enzymes, HCN (Hydrogen cyanide), siderophores production and its ability to induce systemic resistance (Haas and Defago, 2005; Ganeshan and Kumar, 2007; Kedia et al., 2023). Despite all these, the bioagent if applied directly to the field, it may not be able to corroborate the same results obtained in disease suppression as that under lab conditions. This sheds light on the fact that the antagonists are unable to multiply or even survive in the newly introduced niche where the kingdom of microflora and microfauna are already established. Hence, the introduced cells should be protected and amended to multiply and establish itself successfully in the environment (Bashan et al., 2014). Consequently, these bioagents are formulated in different forms for the development of eco-friendly biopesticides to maintain their shelf life, withstand the environmental stresses, and guard the plants from pathogens. This review chapter mainly concentrates on various bioformulations of Pseudomonas spp. which have been regarded as potential antagonist and PGPR fora very long time (Sharma et al., 2003; Maleki et al., 2010; Tiyagi et al., 2011).
FORMULATIONS AND THEIR TYPES
The primary step in formulating a biopesticide is selecting a potent antagonist. Its efficacy has to be determined and growth requirements/culture conditions have to be verified. Once a bioagent is selected, the next step is to assign a suitable “vehicle” to carry this antagonist, provide a substrate for its growth, and ensure protection (Chaudhary et al., 2020). Additionally, they are amended with various cell protectants, preservatives, emulsifiers, adjuvants, UV protectants, foaming and defoaming agents, etc. (in case of liquid formulations), that aids in preserving the cells for a long duration, to improve its efficiency, and to maintain the quality of the product even during unfavorable conditions (Bejarano and Puopolo, 2020). The role of these amendments has been studied to validate their role in maintaining the quality of the formulated products (Biradar and Santhosh, 2018; Shravani et al., 2019, Bhattacharyya et al., 2020). This process was regarded as “secret art” as quoted by Bashan et al., (2014), where a bioagent verified as a potent antagonist is mass multiplied and grown under controlled conditions and designed into a commercial formulation to be applied to the field. Based on the carriers used, the formulation types vary and here we have reviewed the three major formulation methods, namely powder/granular formulations, liquid formulations, and capsule formulations.
Powder/granular formulations: This utilizes a variety of solid organic and inorganic substrates viz., peat, lignite, charcoal, sugarcane bagasse, saw dust, talcum powder, vermiculite, kaolin, etc. as carrier materials and are commonly used in formulations. A recent study elucidated that, P. fluorescens Ur21 solubilized the phosphorus and released it effectively (1,684.39 mgkg-1; 90% dissolved) at different levels of vermicompost (58.8%), phosphate rock (35.3%) and sulfur (5.8%), for formulating biofertilizer using response surface methodology (Barin et al., 2022). Another study reported the peat-based P. fluorescens (freeze dried), maintained the viability of cells for longer duration (Barman et al., 2022). The storage period of P. fluorescens was compared by formulating it on different carriers and found that, talc was the best carrier when it comes to long term storage (6 months), although, spent mushroom substrate and fly ash could be better alternatives, when it comes to short-term storage (Gade et al., 2014). Recently, a study elucidated that, there was no significant difference between using peat/ talc as carrier material, as the population of bioagent, growth promotion offered by them and rhizosphere competence remained comparable in both the formulations. Even though talc-based formulations are widely used, they suffer from many shortcomings, especially, reduced shelf life, high possibility for contaminations, and reduced field performance (Hegde, 2008). The formulations need to be validated based on the strain of bioagent used (Novinscak and Filion, 2020). Granular formulations are also available on the market, which were easier to handle and store, less dusty, and efficient even under stress conditions (Chaudhary et al., 2020).
Liquid based bioformulations: They are user friendly formulations, where the potential bioagent is formulated with a water/ oil based medium and is found to be more efficient in maintaining the shelf life for more than a year, in contrast to solid carrier-based formulations, which lost the viability after six months. The moisture content required for the organism to survive is maintained in such formulations, and therefore, it can withstand field-related stresses such as desiccation, it has a possibility to be sterilized in bulk, and has stress tolerance too (Singleton et al., 2002; Bashan et al., 2014). The growth rate of P. fluorescens was found to be higher when formulated with Sargassum sp. extracts supplemented with silage and molasses as a liquid biofertilizer (Basmal et al., 2019). Liquid formulation with isotonic phosphate buffer and diluted nutrient broth containing 4% glycerol was proved to be efficient in maintaining the phosphate solubilization ability of P. putida and Pantoea agglomerans (Goljanian-Tabrizi et al., 2016). Coconut water was used as a low-cost medium for development of liquid formulation of P. fluorescens, that maintained population and was found effective in plant growth promotion (Anith et al., 2016; Gopal et al., 2019). Increased survival of P. fluorescens was observed in liquid formulation amended with glycerol (5 mM), as compared to that of solid carrier materials (Karunya and Reetha, 2014). Manikandan and Raghuchander (2014a), detected the antibiotic compounds by TLC and HPLC techniques, produced by P. fluorescens in liquid formulation, which supported its biocontrol efficiency. Both under refrigerated and ambient conditions, the population of P. fluorescens was maintained as high in liquid-based formulation as compared to solid carrier based, during the period of storage (Mohle et al., 2019). Pseudomonas sp. MSSRFD41, which was demonstrated as an effective bioagent against Pyricularia grisea in finger millet, was formulated into a liquid based product in KB broth with amendments, and it was found to maintain the viability until 150 days of storage (Sekar et al., 2018). Oil based formulation of the bioagent was found promising in maintaining the shelf life and in curtailing many pathogens (Peeran et al., 2014; Dobhal, 2020).
Capsule based formulations: Microencapsulation is a method of covering the microbes in a coating and thus protecting the living cells from external stresses, along with a gradual release into the environment (Kim et al., 2012). Usually, hydrogels extracted from the seaweed are used as natural encapsulation materials, but synthetic polymers such as polyacrylamide, polyurethane, polystyrene are also utilized (Dommergues et al., 1979; Cassidy et al. 1996; Schoebitz et al., 2013).The storage capability of this formulation is so high that it was stored over years as documented by Trivedi and Pandey (2008) (3 years of shelf life) and Bashan and Gonzalez (1999) (14 years of shelf life). Several physical, chemical and physiochemical processes are adopted as encapsulation technologies (Schoebitz et al., 2013). When cost and efficacy factors were considered, the resources like broth, water, and sterile medium, which are required in enormous quantities, make it remarkably costly. However, when inserted into the soil, capsules have the ability to function as small-scale bioreactors, which could eliminate the requirement for significant bacterial expansion during fermentation. This might make production less expensive. Additionally, when several capsules are positioned surrounding the rhizosphere, each capsule has the ability to raise cell concentrations on its own. This could improve root colonization and the general efficacy of microbial inoculants in the field (Schoebitz et al., 2013). van Elsas et al. (1992) observed a better survival and wheat root colonization by alginate encapsulated P. fluorescens amended with skim milk and bentonite clay.
APPLICATION OF DIFFERENT FORMULATIONS OF PSEUDOMONAS SPP. IN COMBATING VARIOUS PHYTOPATHOGENS
Pseudomonas spp. have served as effective candidates in curtailing the ill effects of disease causing phytopathogens. It is one of the most researched proteobacteria, owing to its traits such as plant growth promotion and disease suppression (Selvakumar et al., 2015). Keeping the aim of sustainable agriculture ahead, these Pseudomonas spp. which has the potential of producing volatile compounds, lytic enzymes, siderophores, secondary metabolites, hormones, and inducing systemic resistance (Panpatte et al., 2016; Kumar et al., 2017; Mehmood et al., 2023), is formulated in various forms. Here under, we discuss the various formulations of Pseudomonas sp. used in disease suppression in crops.
Cereals and millets
The seed, root, and soil applications of peat-based formulations of P. fluorescens PfALR2 were proved to curb Rhizoctonia solani causing sheath blight of rice, along with enhancing the yield (Rabindran and Vidhyasekaran, 1996). Sheath blight and leaf folder incidence was reduced significantly (62.1% and 47.7-56.1%, respectively), when a combination of two P. fluorescens (PF1 & FP7) strains were applied in rice fields as talc-based formulas (Commare et al., 2002). The reduction in sheath blight using solid carrier-based Pseudomonas was also reported by Mathivanan et al., (2005). The blast incidence in rice was highly reduced when liquid formulation of P. fluorescens (BRM 32111) and B. pyrrocinia (BRM32113),was applied in the field, and served as a breakthrough in biopesticide application (de Sousa Oliveria et al., 2020). The carrier-based powder formulations (talc/peat/kaolin etc.) of P. fluorescens were effective in curbing Xanthomonas oryzae pv. oryzae, (Jambhulkar and Sharma, 2014), dirty panicle of rice (Prathuangwong et al., 2013), rice root-knot nematode (Seenivasan et al., 2012), and sheath blight (Vidhyasekaran and Muthamilan, 1999).
Blast disease of finger millet was suppressed in the presence of Pseudomonas sp. MSSRFD41, in in vitro, green house and field conditions, and was formulated further into a liquid biopesticide for further storage. The bioagent has not only reduced the disease incidence by three-fold, but also enhanced the seedling vigor, germination, and general growth of the crop (Sekar et al., 2018). Another study illustrated that a coconut water-based bioagent formulation was potent enough to reduce the disease severity of leaf blast in finger millet besides improving its growth attributes (Shinde et al., 2017). Liquid formulation of P. fluorescens arrested the sheath blight pathogen in rice significantly (64.5%) when applied as seed and foliar treatment and enhanced the yield (Yadav et al., 2020).
Pulses
Talc and sodium alginate-based formulations of P. fluorescens and Trichoderma harzianum were tested against Fusarium udum in pigeon pea in greenhouse trials. The results revealed that, better seedling emergence and reduced disease incidence was associated with talc-based P. fluorescens treated seedlings than other treatments (Niranjana et al., 2009). Chitin amended talc- based formulation of P. fluorescens was effectively used to inhibit Macrophomina phaseolina in mung bean, under in vitro (44.50%), greenhouse (85.62%) and field conditions (82.13%), apart from improving plant growth (Saravanakumar et al., 2007). Suppression of collar rot of chickpea was evaluated with talc formulation of the bioagent and found it promising in both in vitro and greenhouse (Kumar et al., 2021).
A liquid-based formulation of P. fluorescens was developed and its efficacy was tested against various soil borne pathogens viz., F. oxysporum f. sp. ciceri, S. rolfsii and R. bataticola of chickpea. The results demonstrated that, the formulation not only maintained the shelf life of the antagonist, but also inhibited all the three pathogens in vitro throughout the storage period. The glasshouse studies further revealed that application of the formulation at 10 ml/kg of seeds followed by drenching reduced the incidence of wilt, collar rot and dry root rot (50.01%, 59.48% and 59.09%, respectively), as compared to control (Baby, 2023). The inhibition exhibited by the developed liquid formulation on F. oxysporum f. sp. ciceri and R. bataticola, in vitro, is represented in Figure 2.
Oilseeds, fiber crops and other cash crops
The effect of P. fluorescens PS1 with sawdust-soil as a carrier was demonstrated in checking the stem blight of Indian mustard, caused by Sclerotinia sclerotiorum. The formulated P. fluorescens was potent enough to impede the hyphal growth of the pathogen by perforation and lysis, even after a storage period of one year, apart from improving the growth traits (Aeron et al., 2011). A similar effect of disease suppression was observed when cotton seeds were treated with bentonite-based P. fluorescens, against R. solani inciting damping off, which further ascertained the role of formulating the biocontrol agents for better efficacy (Ardakani et al., 2010). A notable reduction in groundnut leaf spot and rust diseases was observed by the seed and foliage application of P. fluorescens with talc-based formulation and was found to be more efficient than the fungicidal treatment (Meena et al., 2002).
Red rot of sugarcane incitant, Colletotrichum falcatum was effectively managed in the field when liquid formulation of P. fluorescens was applied at 4 lit/ha and the disease incidence was recorded in a range between 2.34 - 6.45% (1st trial), 2.47 - 5.17% (2nd trial) and 4.23 - 6.51% (3rd trial), as compared to the control treatment (13.72- 28.56%, 9.62-26.44% and 8.83- 20.75%, respectively). In addition, the formulation also reduced early shoot borer and leaf hopper population in the field (Senthil et al., 2011).
Horticultural crops
Pseudomonas spp. have wider application when it comes to horticulture crops. A dry formulation of P. fluorescens EPS62e was developed through lyophilization with lactose as cryoprotectant, and was additionally osmo adapted. This procedure increased the survivability of the antagonistic cells (100%) for a year and enhanced the biocontrol activity against Erwinia amylovora inciting fire blight of pear at low relative humidity (Cabrefiga et al., 2014). Talc-based formulation of P. fluorescens was even found effective in management of tomato spotted wilt virus (TSWV), along with causing increment in the yield (Kandan et al., 2005). The damping off of cucumber caused by Phytophthora capsiciwas effectively suppressed in the presence of seed treatment and pre-plant amendment with irradiated talc and peat formulations of P. fluorescens Pf 9A-14 (85% healthy seedlings) and promoted the growth as well. This was even better than the disease control offered by chemical treatment with metalaxyl (69% healthy seedlings) or with mere cell suspension of the same bacteria (78% healthy seedlings) and even the control (3-6% healthy seedlings) (Khabbaz et al., 2015).The same study elucidated that combined or individual application of irradiated peat formulation of bacteria viz., Pseudomonas fluorescens (Pf 9A-14), Bacillus subtilis (Bs 8B-1) and Pseudomonas sp. (Psp.8D-45) as seed treatment resulted in higher survival of seedlings (25-51%) as compared to that of control (5%). These talc formulations performed well against Pythium damping-off, besides improving the growth parameters of cucumber. Even though the population decreased over time, it was maintained as high as 108 cfu/g at 180th day of storage in irradiated talc and peat formulations (Khabbaz and Abbasi, 2014). The plant growth promotion and disease reduction were also observed in tomato seedlings against Fusarium oxysporum f. sp. radicis-lycopersici, when treated with talc or peat formulations of six bacterial strains, comprising mainly of Pseudomonas spp. (Zhang et al. 2015). Wettable powder formulation of P. syringe and P. fluorescens were also illustrated as efficient for managing post-harvest diseases of fruits (Keswani et al., 2016).The improvement in growth parameters (shoot and root characters) were also recorded in shallots treated with powdered formulation of P. fluorescens and Bacillus coagulans, where the carrier materials used were groundnut shell and water hyacinth (as powder carriers, respectively), and liquid carriers (herbal mixture solution and archae bacterial solution (as liquid carriers respectively) (Yaashikaa et al., 2020). The CaCO3 based formulation of P. fluorescens was found effective in reducing the radial growth of two important soil borne pathogens viz., Fusarium solani and R. solani affecting egg plants, throughout their six months of storage. In addition, the formulation also restrained the egg hatching (5%) and caused the death of J2 stage of Meloidogyne incognita (44%) in egg plants as well (Al-Waily et al., 2018). The field experiment conducted with vermicompost and carboxymethyl cellulose formulation (CVPf) of P. fluorescens in brinjal against bacterial wilt proposed a suppression of the disease up to 83.33%, as it maintained higher cell population of the antagonist, apart from enhancing the yield attributes (Chakravarty and Kalita, 2011; Chakravarty, 2023). Soft rot causing by Pectobacterium carotovorum was effectively managed in Kale by a novel formulation of P. fluorescens SP007S, comprising of sugar sludge, paper sludge and glycerol 1%. The disease incidence was reduced up to 65.2% by the treatment with this formulation and was better than that of cell suspension alone (Nilmat et al., 2023). This underscores the importance of formulating the bioagent, where it sustains the population of antagonist, without losing its efficacy. The efficiency in biocontrol of solid carrier-based formulation of this antagonist bacteria is documented by other researchers also (Moënne‐Loccoz et al., 1999; Soesanto et al., 2019; Rani et al., 2022).
Enhanced suppression of soil borne pathogens like Fusarium oxysporum f. sp. lycopersici and Sclerotium rolfsii, was observed when talc-based P. fluorescens coupled with FYM (farmyard manure) was inoculated in tomato plants. This also induced defense enzymes, adding to its efficiency (Johnson et al., 2022). Liquid formulation of the bacteria restrained Fusarium oxysporum f. sp. Lycopersici (FOL), in tomato plants and the chitinase activity was found to be better in liquid formulation than talc-based formulation (Manikandan and Raguchander, 2015). The reports suggest this formulation even induced defense related enzymes (phenylalanine ammonia lyase (PAL), peroxidase (PO), polyphenol oxidase (PPO), catalase, β-1,3 glucanase and super oxide dismutase (SOD) in tomato plants, and therefore, proved the efficiency of bioformulation (Manikandan and Raguchander, 2014b). Effect of liquid Pseudomonas against this pathogen was further studied widely and have concluded that the shelf life was found up to 210 days. This was efficient in suppressing the wilt pathogen all throughout the storage period and even in glasshouse and field conditions (Paranthaman et al., 2022). Apart from this, liquid formulation of P. fluorescens FP7 remarkably reduced rhizome rot of turmeric (10.18% and13.29% in two field trials) and furthermore, produced an array of defense molecules, promoted growth and improved yield (Prabhukarthikeyan et al., 2018). Glycerol-based formulation of P. fluorescens was proved to be efficient in arresting the grey mold incidence in strawberries, and was found to be even better than chemical treatments in reducing the severity and promoting plant growth (Haggag and El Soud, 2012). Yet another water-in-oil formulation of this bioagent was found to be effective in managing Colletotrichum musae, as it reduced the post-harvest anthracnose incidence and improved the bunch parameters and yield of banana (Peeran et al., 2014). Liquid based P. fluorescens which maintained maximum viability over a year, was also found promising in augmenting the growth of tomato plants apart from suppressing the egg hatching and causing mortality of juveniles of root knot nematode (Meena et al., 2012). Potato cyst nematode was managed successfully by seed treatment with liquid P. fluorescens and Purpureocillium lilacinum, which reduced egg density, multiplication rate, root penetration and number of eggs per cyst, during the field trials (Nagachandrabose, 2020). Similar work to determine on efficacy of liquid-based P. fluorescens in curbing pathogens of horticulture crops also validated its antagonistic potential (Sendhilvel et al., 2007; Peighami-Ashnaei et al., 2009; Manikandan et al., 2010; Pushpa et al., 2014; Selvaraj et al., 2014; Seenivasan, 2017).
Microencapsulated Pseudomonas chlororaphis with alginate-whey protein exhibited reduction in Rhizoctonia solani infection in potato plants (70% reduction), thus ascertaining the importance of encapsulation of bacteria for better efficiency (Fathi et al., 2021). Nano encapsulation of two strains of P. fluorescens (VUPF5 and T17-4) was demonstrated in greenhouse to repress Fusarium solani in potato. The disease reduction offered by capsulated bacterial strains (81% and 75% respectively) was found to be better than that compared to non-capsulated bacteria (76% and 63%, respectively). It also had better shelf life and improved the growth of plants than non-coated bacteria (Pour et al., 2019).
COMMERCIAL FORMULATIONS OF PSEUDOMONAS SPP. a
GENERAL MECHANISMS OF ACTION OF PSEUDOMONAS SPP.
The biocontrol agents gain an upper hand over the pathogens as they exert several mechanisms to ensure its own survival. They are regarded as effective competitors, and some important mechanisms are discussed here under.
Competition for nutrients: This is considered as one of the basic mechanisms of action, where the bioagents compete with pathogenic organisms for food and space. Pseudomonas spp. colonize, compete and acquire the nutrients required for their rapid growth, and thereby makes the pathogens thrive in the same environment (Walsh et al., 2001; Panpatte et al., 2016). The iron sequestering compound, siderophore, produced by Pseudomonas spp., chelate the Fe3+ ions in the soil and thereby limit the pathogens from acquiring them. This provides an extra advantage for Pseudomonas to survive in the soil rather than pathogens which require iron for its growth (Loper and Buyer, 1991). The role of siderophores is widely demonstrated in disease suppression (Solans et al., 2016; Arya et al., 2018, Jadav, 2021; Baby, 2023).
Production of HCN and antibiotics: The secondary metabolite HCN, produced by Pseudomonas spp. serves as a volatile inhibitory toxic compound that delimits the growth of pathogen (Voisard et al., 1989; Jayaprakashvel et al., 2011; Jadav, 2021; Baby, 2023). Antibiotics play key roles in pathogen inhibition and have a broad-spectrum activity. Pseudomonas is known to synthesize an array of antibiotics viz., 2,4-diacetylphloroglucinol (2,4-DAPG), phenazine, pyoluteorin, and pyrrolnitrin, pyochelin, etc. that aid in inhibition of pathogen growth (Voisard et al., 1989; Reddy et al., 2009; Compant et al. 2010; Castaldi et al., 2021; Trejo- Raya et al., 2021).
Production of lytic enzymes and induction of ISR: The hydrolytic enzymes viz., cellulases, chitinases, glucanses, proteases, peroxidases, phenylalanine ammonia lyase etc., synthesized by the bacteria, results in cell wall degradation and disruption of metabolic functions, finally leading to disease suppression (Rai, 2011; Suresh et al., 2022). It is also known to possess ACC-deaminase activity that hinder the ethylene levels and thus, disease reduction (Gamalero et al., 2017). These beneficial microbes have yet another capability to induce the systematic resistance in plants, following their application. This paves the way to the enhancement of plant defense against deleterious pathogens (van Loon and Bakker, 2006; Singh et al., 2015; Kannojia et al., 2019).
LIMITATIONS OF THE FORMULATIONS
Even though there are ample reports on the antagonistic ability of highly efficient strains of biocontrol agents, the development of their effective formulation is still not yet fully achieved. The biopesticides are always questioned for their field efficiency, even though they exhibit potential inhibition in vitro (Shaikh and Sayyed, 2015; Keswani et al., 2016). One of the reasons behind this is the reduction in population of active cells, and therefore the performance capability decreases. It even has to adapt to the new environment which is totally heterogeneous in nature and has to interact with already established microflora. This can affect the field performance significantly (Glick et al., 1999). Shelf life is another constraint in developing formulations. The strain of antagonist used, carriers, production methodologies adopted, packaging and transport influences shelf life (Keswani et al., 2016). During storage, there is a possibility of contaminations with undesired microbes, that will deplete the quality and efficiency of the product (Arora et al. 2010). There are the general factors pulling backward the biopesticide industry from growth.
CONCLUSION
Biological control of plant diseases has always been a part of research, when the world seeks out for better alternatives for hazardous chemicals. Pseudomonas spp. served as an efficient antagonist and plant growth promoter, improving the plant health. The review has focused on the bioformulations of this bacterium, its efficacy in tackling the pathogens of major crops, and the mechanisms of action. The efficacy of biopesticides will usually last for about 15 to 20 days while interaction with the targeted pathogens under field conditions. There exists a wide scope to be explored in formulating an efficient biopesticide, which exhibits its maximum potential in field that can benefit the farmers in terms of reducing the cost of cultivation with due emphasis on safeguarding the environment and for sustainable yields.
REFERENCES
Aeron, A., R. C. Dubey, D. K. Maheshwari, P. K. Pandey, V. K. Bajpai andS. C. Kang. 2011. Multifarious activity of bioformulatedPseudomonas fluorescens PS1 and biocontrol of Sclerotinia sclerotiorum in Indian rapeseed (Brassica campestris L.). Eur. J. Plant Pathol. 131: 81-93.
Al-Waily, D.S., L. A. Al-Saad and S. S. Al-Dery. 2018. Formulation of Pseudomonas fluorescens as a biopesticide against soil borne root pathogens. Iraqi J. Agric.l Sci. 49(2):235-242.
Anith K. S., A. S. Vyshakhi,A. Viswananthan, S. Varkey, and S. Aswini. 2016. Population dynamics and efficiency of coconut water based liquid formulation of Pseudomonas fluorescens AMB-8. J. Trop. Agri. 54(2): 184-189.
Arora, N. K., E. Khare andD. K. Maheshwari. 2010. Plant growthpromoting rhizobacteria: constraints inbioformulation, commercialization, and futurestrategies. In: Plant growth andhealth promoting bacteria (Ed. Maheshwari, D. K.), Springer, Berlin, 97–116.
Arya, N., A. Rana, A. Rajwar, M. Sahgal and A. K. Sharma. 2018. Biocontrol efficacy of siderophore producing indigenous Pseudomonas strains against Fusarium wilt in tomato. Natl. Acad. Sci. Lett.41: 133–136.
Baby, A. S. 2023. Standardisation and evaluation of liquid formulations of Pseudomonas fluorescens (Flugge) Migula against soil borne fungal diseases of chickpea. M.Sc. (Agri.) Thesis, University of Agricultural Sciences, Dharwad, Karnataka, India.
Barin, M., F. Asadzadeh, M. Hosseini, E. C. Hammer, R. R.Vetukuri, R. Vahedi. 2022. Optimization of biofertilizer formulation for phosphorus solubilizing by Pseudomonas fluorescens Ur21 via response surface methodology. Processes. 10: 650.
Barman, N.C., M.S Sarker, M. Ahmed, Z. Ahmedand S. Ramachandran. 2023. Optimizing shelf-life of Pseudomonas fluorescens after freeze drying. In:Pseudomonas aeruginosa - new perspectives and applications [working title] (Eds.Dharwesh O. M. and I. Matter). IntechOpen. http://dx.doi.org/10.5772/intechopen.108034.
Bashan, Y. and L. E. Gonzalez. 1999. Long-term survival of the plant-growthpromotingbacteria Azospirillum brasilense and Pseudomonasfluorescens in dry alginate inoculant. Appl.Microbiol.Biotechnol. 51: 262–266.
Bashan, Y., L.E.de-Bashan, S.R. Prabhu, J. Hernandez. 2014. Advances in plant growth-promoting bacterial inoculant technology: formulations and practical perspectives (1998–2013). Plant Soil. 378: 1–33.
Basmal, J., M. E.Aribowo, Nurhayatiand R.Kusumawati. 2019. Growth rate of Pseudomonas fluorescens in liquid fertilizer from brown seaweed (Sargassum sp.) extracts. IOP Conf. Ser.: Earth Environ. Sci. 383: 012027.
Bejarano,A. andG. Puopolo. 2020. Bioformulation of microbial biocontrol agents for a sustainable agriculture. In: How research can stimulate the development of commercial biological control against plant diseasesprogress in biological control (Vol 21) (Eds. A. De Cal, P. Melgarejo and N. Magan), Springer, Cham.
Berninger, T., Ó.González López, A. Bejarano, C. Preininger and A. Sessitsch, 2018. Maintenance and assessment of cell viability in formulation of non‐sporulating bacterial inoculants. Microb.Biotechnol. 11(2): 277-301.
Bhattacharyya, C., R. Roy, P. Tribedi, A. Ghosh and A. Ghosh. 2020. Biofertilizersas substitute to commercial agrochemicals. In: Agrochemicals detection, treatment and remediation (Eds.Majeti N.,P. Vara),Butterworth-Heinemann, 263–290.
Biradar, P. B. J. and G. P. Santhosh. 2018. Cell protectants, adjuvants, surfactant and preservative and their role in increasing the shelf life of liquid inoculant formulations of Pseudomonas fluorescens. Int. J. Pure Appl. Biosci. 6(4): 116-122.
Cabrefiga, J., J. Francés, E. Montesinosand A. Bonaterra. 2014. Improvement of a dry formulation of Pseudomonas fluorescens EPS62e for fire blight disease biocontrol by combination of culture osmoadaptation with a freeze‐drying lyoprotectant. J.Appl.Microbiol. 117(4): 1122-1131.
Cassidy, M. B., H. Lee and J. T.Trevors. 1996. Environmental applications of immobilized microbial cells: a review. J. Ind. Microbiol. 16: 79–101.
Castaldi, S., M. Masi,F. Sautua, A. Cimmino, R. Isticato, M. Carmona, A. Tuzi and A. Evidente. 2021.Pseudomonas fluorescens showing antifungal activity against Macrophomina phaseolina, a severe pathogenic fungus of soybean, produces phenazine as the main active metabolite. Biomolecules. 11(11): 1-13.
Chakravarty, G. 2023. Management of bacterial wilt disease of brinjal by P. fluorescens based bioformulation: experimental investigation. In: Emerging Issues in Agricultural Sciences (Vol. 2) (Ed. Al-Naggar A. M.), B P International, 130-150.
Chakravarty, G. and M. C. Kalita. 2011. Comparative evaluation of organic formulations of Pseudomonas fluorescens based biopesticides and their application in the management of bacterial wilt of brinjal (Solanum melongena L.). Afr. J.Biotechnol. 10(37): 7174-7182.
Chaudhary, T., M. Dixit, R. Gera, A. K. Shukla,A. Prakash, G.Guptaand P. Shukla. 2020. Techniques for improving formulations of bioinoculants. 3 Biotech. 10: 199.
Commare, R.R., R. Nandakumar, A. Kandan, S. Suresh, M. Bharathi, T. Raguchanderand R. Samiyappan. 2002. Pseudomonas fluorescens based bio-formulation for the management of sheath blight disease and leaffolder insect in rice. Crop Prot. 21(8): 671-677.
Compant, S., C. Clément and A. Sessitsch. 2010. Plant growth-promotingbacteria in the rhizo and endosphere of plants: their role,colonization, mechanisms involved and prospects for utilization. Soil Biol.Biochem. 42:669–678.
de Sousa Oliveira, M.I., A. A. Chaibub, T. P. Sousa, M.V.C.B.Cortes, A. C. A. de Souza, E. C. da Conceiçãoand M.C. C. de Filippi. 2020. Formulations of Pseudomonas fluorescens and Burkholderia pyrrocinia control rice blast of upland rice cultivated under no-tillage system. Biol.Control, 144: 104153.
Dobhal, A. 2020. Development of liquid formulation of Pseudomonas fluorescens (Flugge) Migula and their efficacy against spot blotch of wheat. M.Sc. (Agri.) Thesis, University of Agricultural Sciences, Dharwad, Karnataka, India.
Dommergues, Y. R., H. G. Diem and C.Divies. 1979. PolyacrylamideentrappedRhizobium as an inoculant for legumes. Appl. Environ.Microbiol. 37:779–781.
Fathi, F., R. Saberi Riseh, P. Khodaygan,S. Hosseini,Y. A. Skorik. 2021. Microencapsulation of a Pseudomonas Strain (VUPF506) in Alginate–Whey Protein–Carbon Nanotubes and Next-Generation Sequencing identification of this strain. Polymers.13(23): 4269. https://doi.org/10.3390/polym13234269
Gade, R.M., B. H. Chaithanyaand K. C. Khurade. 2014. A comparative study of different carriers for shelf life of Pseudomonas fluorescens. The Bioscan. 9(1): 287-290.
Gamalero, E.,C. Marzachi, L. Galetto, F. Veratti, N. Massa, E.Bona, G. Novello, B. Glick, S. Ali, S. Cantamessa, D’Agostino, G. and Berta, G. 2017. An 1-Aminocyclopropane-1-carboxylate (ACC) deaminase-expressing endophyteincreases plant resistance to FlavescenceDoree phytoplasma infection. Plant Biosyst. 151(2): 331−340.
Ganeshan, G. and M. A. Kumar. 2007.Pseudomonas fluorescens, a potentialbacterial antagonist to control plant diseases. J. Plant Interact. 1(3): 123-134.
Glick, B. R., C. L. Patten, G. Holguin andD. M. Penrose. 1999. Biochemical and genetic mechanisms used by plantgrowth promoting bacteria. Imperial College Press, London
Gopal, M., A. Gupta, K. S. Hameed, R. Chandramohanan and G. V. Thomas. 2019. A simple, quick and contamination-free method for mass-multiplication of plant-beneficial microbes by small and marginal farmers using coconut water and rice gruel medium. Indian J. Agric. Sci. 89(2): 339-343.
Haas, D. and G. Defago. 2005. Biologicalcontrol of soil-borne pathogens byfluorescent Pseudomonads. Nat. Rev.Microbiol. 3: 307-319.
Haggag, W. M. and M. A. El Soud. 2012. Production and optimization of Pseudomonas fluorescens biomass and metabolites for biocontrol of strawberry grey mould. Am. J. Plant Sci. 3(7): 836-845.
Hegde, S. V. 2008. Liquid bio-fertilizers in Indian agriculture. Bio-fertilizer newsletter, 12: 17-22.
Jadav, P. C. 2021. Evaluation of microbial consortia for the management of foot rot of wheat caused by Sclerotium rolfsii Sacc. M. Sc. (Agri.) Thesis, University of Agricultural Sciences, Dharwad, Karnataka, India.
Jambhulkar, P.P. and Sharma, P. 2014. Development of bioformulation and delivery system of Pseudomonas fluorescens against bacterial leaf blight of rice (Xanthomonas oryzaepv. oryzae). J.Environ.Biol. 35(5): 843-849.
Jayaprakashvel, M., R. Muthezhilan, R. Srinivasan, A. Hussain, S. Gobalakrishnan, J. Bhagat, C. Karthigeyan and R. Muthulakshmi. 2010. Hydrogen cyanide mediated biocontrol potential of Pseudomonas sp. AMET1055 isolated from the rhizosphere of coastal sand dune vegetation. J Advanced Biotech. 9(10): 39-42.
Johnson, I., B. Sreenayana, V.P. Suruthi, R.Manikandan, R.Ramjegathesh and M. Karthikeyan. 2022. Rhizosphere population dynamics and biocontrol potential of Pseudomonas fluorescens Pf1 against Wilt and collar rot pathogens in tomato. Pharma Innovat. 11(5): 1042-1051.
Kandan, A., M. Ramiah, V. J. Vasanthi, R. Radjacommare, R. Nandakumar, A. Ramanathanand Samiyappan, R. 2005. Use of Pseudomonas fluorescens-based formulations for management of tomato spotted wilt virus (TSWV) and enhanced yield in tomato. Biocontrol Sci.Technol. 15(6): 553-569.
Kannojia, P.,K. K. Choudhary, A. K. Srivastava andA. K. Singh. (2019). PGPR bioelicitors: induced systemic resistance (ISR) and proteomicperspective on biocontrol. In: PGPR amelioration in sustainableagriculture (Eds. Singh, A. K., A. Kumar andP. K. Singh), Elsevier, 67−84.
Karunya, S.K. andD. Reetha. 2014. Survival of saline tolerant PGPR in different carriers and liquid formulations.Int. J. Adv. Res. Biol.Sci. 1(2): 179-183.
Kedia, V. K., M. Sharma and K. Shantanu. 2023. Pseudomonas sp.: A promising biocontrol agent against selected phytopathogens. J. Mountain Res. 18(1): 245-255.
Keswani, C., K. Bisen, V. Singh, B. K. Sarma and H. B. Singh. 2016. Formulation technology of biocontrol agents: present status and future prospects. In: Bioformulations: for sustainable agriculture (Eds. Arora N. K., S. M. Raff, R. Balestrini),Springer, Varanasi,35–52.
Khabbaz, S.E. and P.A. Abbasi. 2014. Isolation, characterization, and formulation of antagonistic bacteria for the management of seedlings damping-off and root rot disease of cucumber. Can.J. Microbiol. 60(1): 25-33.
Khabbaz, S.E., L. Zhang, L. A. Cáceres, M. Sumarah, A. Wang and P. A. Abbasi. 2015. Characterisation of antagonistic Bacillus and Pseudomonas strains for biocontrol potential and suppression of damping‐off and root rot diseases. Ann. Appl. Biol. 166(3): 456-471.
Kim, I. Y., P. L. Pusey, Y. Zhao, S. S. Korban, H. Choi and K. K. Kim. 2012. Controlled release of Pantoeaagglomerans E325 for biocontrol of fire blight disease of apple. J. Control Release 161: 9–15.
Kumar, A., H. Verma, V. K. Singh, P. P. Singh, S. K. Singh, W. A. Ansari, A. Yadav, P. K. Singhand K. D. Pandey. 2017. Role of Pseudomonas sp. in sustainable agriculture and disease management. In: Agriculturally important microbes for sustainable agriculture (Vol 2) Applications in crop production and protection(Eds. Meena, V., P. Mishra, J. Bisht, A.Pattanayak), 195-215.
Kumar, R., P. Bhargava and D. Sinha. 2021.Pseudomonas based formulation for biocontrol of Sclerotium rolfsii causing collar rot of chickpea. Biological forum, 13(4): 1100-1103.
Loper, J. E. andJ. S. Buyer. 1991. Siderophores in microbialinteractions on plant surfaces. Mol Plant-Microbe Interact. 4:5–13.
Maleki, M., S. Mostafaee, L.Mokhtarnejad and M. Farzaneh. 2010. Characterization ofPseudomonas fluorescens Strain CV6 isolated from cucumber rhizosphere in varamin as a potential biocontrol agent. Aust.J Crop Sci. 4(9): 676-683.
Manikandan, R. and Raguchander, T. 2015. Pseudomonas fluorescens (Pf1) mediated chitinolytic activity in tomato plants against Fusarium oxysporum f. sp. lycopersici. Afr. J. Microbiol. Res. 9: 1331-1337.
Manikandan, R. andT. Raguchander, T. (2014a). Detection of antibiotic compounds from liquid formulation of Pseudomonas fluorescens (Pf1) by TLC and HPLC analysis. Journal of Pure and Applied Microbiology. 8. 4025-4030.
Manikandan, R. and T. Raguchander.(2014b). Fusarium oxysporum f. sp. lycopersici retardation through induction of defensive response in tomato plants using a liquid formulation of Pseudomonas fluorescens (Pf1). Eur J Plant Pathol. 140: 469–480.
Manikandan, R., D. Saravanakumar, L. Rajendran, T. Raguchanderand R. Samiyappan. 2010. Standardization of liquid formulation of Pseudomonas fluorescens Pf1 for its efficacy against Fusarium wilt of tomato. Biological control. 54(2): 83-89.
Mathivanan, N., V. R. Prabavathy and V. R. Vijayanandraj. 2005. Application of talc formulations of Pseudomonas fluorescensMigula and Trichoderma viride Pers. ex SF Gray decrease the sheath blight disease and enhance the plant growth and yield in rice. J Phytopathol. 153(11‐12): 697-701.
Meena, K.S., E. I. Jonathanand P. G. Kavitha. 2012. Viability of Pseudomonas fluorescens in liquid formulation and its effect on plant growth promotion and inhibition of root knot nematode, Meloidogyne incognita. Madras Agric. J. 99(10-12): 850-853.
Meena, B., R. Radhajeyalakshmi, T. Marimuthu, P. Vidhyasekaran and R. Velazhahan. 2002. Biological control of groundnut late leaf spot and rust by seed and foliar applications of a powder formulation of Pseudomonas fluorescens, Biocontrol Sci Technol. 12(2): 195-204.
Mehmood, N., M. Saeed, S. Zafarullah, S. Hyder, Z. F. Rizvi, A. S. Gondal, N. Jamil, R. Iqbal, B. Ali, S. Ercisli and M. Kupe. 2023. Multifaceted impacts of plant-beneficial Pseudomonas spp. in managing various plant diseases and crop yield improvement. ACS omega, 8(25): 22296-22315.
Moënne‐Loccoz, Y., M. Naughton, P. Higgins, J. Powell, B. O'connorand F. O'gara. 1999. Effect of inoculum preparation and formulation on survival and biocontrol efficacy of Pseudomonas fluorescens F113. J. Appl. Microbiol. 86(1): 108-116.
Mohle,K. K., R. K. S. Tiwari, M. Tondeyand K, Kumar. 2019. Shelf life study of Pseudomonas fluorescens in talc based carrier and liquid formulations. Int. J. Chem. Studies. 7(6): 922-925.
Nagachandrabose, S. 2020. Management of potato cyst nematodes using liquid bioformulations of Pseudomonas fluorescens, Purpureocilliumlilacinum and Trichoderma viride. Potato Research, 63(4), pp.479-496.
Nilmat, A., W. Thepbandit, W.Chuaboon and D. Athinuwat. 2023. Pseudomonas fluorescens SP007S formulations in controlling soft rot disease and promoting growth in kale. Agronomy, 13(7): 1856.
Niranjana, S. R., S. Lalitha and P. Hariprasad. 2009. Mass multiplication and formulations of biocontrol agents for use against fusarium wilt of pigeonpea through seed treatment, Int J Pest Manag. 55(4): 317-324.
Novinscak, A. and M. Filion. 2020. Long term comparison of talc-and peat-based phytobeneficialPseudomonas fluorescens and Pseudomonas synxantha bioformulations for promoting plant growth. Front. Sustain. Food Syst. 4: 602911.
Pal, K. K. and B. M. Gardener. 2006. Biological control of plant pathogens. Plant Health Instr. 1–25.
Panpatte, D. G., Y. K. Jhala, H. N. Shela and R. V. Vyas. 2016. Pseudomonas fluorescens: A Promising Biocontrol Agent and PGPR for Sustainable Agriculture. In: Microbial inoculants in sustainable agricultural productivity (Eds. Singh, D. P., H. B. Singh, R. Prabha),Springer, New Delhi, 257–270.
Peeran, M.F., N. Krishnan, P. R. Thangamani, K. Gandhi, T. Raguchander, and P. Kuppusamy. 2014. Development and evaluation of water-in-oil formulation of Pseudomonas fluorescens (FP7) against Colletotrichum musae incitant of anthracnose disease in banana. Eur. J. Plant Pathol. 138: 167-180.
Peighami-Ashnaei, S., A. Sharifi-Tehrani, M. Ahmadzadeh and B. Keivan. 2009. Interaction of different media on production and biocontrol efficacy of Pseudomonasfluorescens P-35 and Bacillus subtilis B-3 against grey mould of apple. Journal of Plant Pathology, 91(1): 65-70.
Pour, M.M., R. Saberi-Riseh, R. Mohammadinejad and A. Hosseini. 2019. Investigating the formulation of alginate-gelatin encapsulated Pseudomonas fluorescens (VUPF5 and T17-4 strains) for controlling Fusarium solani on potato. Int.J. Biol.Macromol. 133: 603-613.
Prabhukarthikeyan, S.R., U. Keerthanaand T. Raguchander. 2018. Antibiotic-producing Pseudomonas fluorescens mediates rhizome rot disease resistance and promotes plant growth in turmeric plants. Microbiol. Res. 210: 65-73.
Prathuangwong, S., D. Athinuwat, W.Chuaboon, T.Chatnaparat and N. Buensanteai. 2013. Bioformulation Pseudomonas fluorescens SP007s against dirty panicle disease of rice. Afr. J Microbiol. Res. 7(47): 5274-5283.
Pushpa, A., P. C. Subhash, M. A. H. Reddy. 2014. Development of liquid formulation for the dual purpose of crop protection and production. J. Environ. Res. Dev. 8:378–383.
Rabindran, R. andP. Vidhyasekaran. 1996. Development of a formulation of Pseudomonas fluorescens PfALR2 for management of rice sheath blight. Crop Prot. 15(8): 715-721.
Rai, V. 2011. Screening of Pseudomonas fluorescens isolates forbiological control of Macrophomina phaseolina root-rot of safflower.Afr. J. Agric. Res.6(29): 6256−6266.
Rani, P., M. Singh, H. Prashadand M. Sharma. 2022. Evaluation of bacterial formulations as potential biocontrol agents against the southern root-knot nematode, Meloidogyne incognita. Egypt. J. Biol. Pest Control. 32(1): 29.
Reddy, B. P., M. S. Reddy and K. V. K. Kumar. 2009. Characterization of antifungal metabolites of Pseudomonas fluorescens and their effect on mycelial growth of Magnaporthe grisea and Rhizoctonia solani. Int. J. Chem. Tech. Res. 1(4): 1490-1493.
Saravanakumar, D., S. Harish, M. Loganathan, R. Vivekananthan, L. Rajendran, T. Raguchander and R. Samiyappan. 2007.Rhizobacterial bioformulation for the effective management of Macrophominaroot rot in mungbean. Arch.Phytopathol. Plant Prot. 40(5): 323–337.
Schoebitz, M.,M. López andA. Roldán.2013. Bioencapsulation of microbial inoculants for bettersoil–plant fertilization. A review. Agron. Sustain. Dev. 33(4): 751-765.
Seenivasan, N. 2017. Combined application of Pseudomonas fluorescens and Purpureocilliumlilacinum liquid formulations to manage Globoderaspp on potato. J. Crop Prot. 6(4): 529-537.
Seenivasan, N., P. M. M. David, P. Vivekanandanand R. Samiyappan. 2012. Biological control of rice root-knot nematode, Meloidogyne graminicola through mixture of Pseudomonas fluorescens strains. Biocontrol Sci. Technol. 22(6): 611-632.
Sekar, J., K. Raju, P. Duraisamy and V. P. Ramalingam. 2018. Potential of finger millet indigenous rhizobacterium Pseudomonas sp. MSSRFD41 in blast disease management—growth promotion and compatibility with the resident rhizomicrobiome. Front. Microbiol. 9:1029.
Selvakumar, G. P., G. H. Panneerselvam, A. N. Bindu and Ganeshamurthy. 2015. Pseudomonads: plant growth promotion and beyond. Plant microbes symbiosis: applied facets (Ed. Arora, N.K.), Springer India, 193–208.
Selvaraj, S., P. Ganeshamoorthi,T. Anand, T. Raghuchander, N. Seenivasan and R. Samiyappan. 2014. Evaluation of a liquid formulation of Pseudomonas fluorescens against Fusarium oxysporum f.sp. cubense and Helicotylenchusmulticinctus in banana plantation. BioControl. 59: 345-359.
Sendhilvel, V., T. Marimuthuand R. Samiappan. 2007. Talc-based formulation of Pseudomonas fluorescens-induced defense genes against powdery mildew of grapevine. Arch.Phytopathol. Plant Prot. 40(2): 81-89.
Senthil, R., S. Selvaraj, T. Anand, T. Raguchanderand R. Samiyappan. 2011. Efficacy of liquid Pseudomonas fluorescens (Pf1) against sugarcane red rot, caused by Colletotrichum falcatum, under field conditions. Int.Sugar J. 113(1356): 888.
Shaikh, S.andR. Sayyed. 2015. Role of plant growth-promoting rhizobacteria and their formulation in biocontrol of plant diseases. In book: Plant microbes symbiosis: applied facets(Ed. Arora, N.K.),Springer India, 337-351.
Sharma, A., B.N.Johri, A.K. Sharma, and B. R. Glick. 2003. Plant growth-promoting bacterium Pseudomonas sp. strain GRP3 influences iron acquisition in mung bean (Vigna radiata L. Wilzeck). Soil Biol.Biochem. 35(7): 887-894.
Shinde, M. S., A. N. Sabalpara and A. Deshmukh. 2017. Mass multiplication and shelf-life study of liquid formulation of Pseudomonas sp. & their effect on PGPR activity and blast disease of finger millet. Green Farming Int. J. 8: 214-217.
Shravani, K., S.Triveni, P. C. Latha and K. D. Chari. 2019. Evaluation of shelf life and quality of carrier and liquid based biofertilizers. Int. J.Microbiol. Res. 11(6): 1598-1601.
Singh, N. P., R. K. Singh, V. S. Meena and R. K. Meena. 2015. Can we use maize (Zea mays) rhizobacteria as plant growth promoter? Vegetos. 28(1): 86-99.
Singleton, P., H. Keyser, E. Sande. 2002. Development and evaluation of liquid inoculants. In: Inoculants and nitrogen fixation of legumes in Vietnam (Ed. Herridge, D.), ACIAR: Canberra, Australia, 109e, 52-66.
Soesanto, L., E. Mugiastuti and K. Khoeruriza. 2019. Granular formulation test of Pseudomonas fluorescens P60 for controling bacterial wilt (Ralstonia solanacearum) of tomato in planta. AGRIVITA. 41(3): 513-523.
Solans, M., J. M. Scervino, M. I.Messuti, G. Vobis and L. G. Wall. 2016. Potential biocontrol actinobacteria: rhizospheric isolates from the Argentine pampas lowlands legumes. J. Basic Microbiol. 54: 585-596.
Suresh, P.,V. Shanmugaiah, R. Rajagopal,K. Muthusamy and V.Ramamoorthy. 2022. Pseudomonas fluorescens VSMKU3054 mediatedinduced systemic resistance in tomato against Ralstonia solanacearum.Physiol. Mol. Plant Pathol.119: 101836.
Tariq, M.,A. Khan, M. Asif, F. Khan, T. Ansari, M.Shariq andM. A. Siddiqui. 2020. Biological control: a sustainable and practical approachfor plant disease management. Acta Agric. Scand. B. Soil Plant Sci. 70:6, 507-524.
Tiyagi, S.A., I. Mahmood, Z. Khan and H. Ahmad. 2011. Biological control of soil-pathogenic nematodes infecting mungbean using Pseudomonas fluorescens. Arch.Phytopathol. Plant Prot. 44(18): 1770-1778.
Trejo-Raya, A.B., V. M.Rodríguez-Romero, S. Bautista-Baños, F. R. Quiroz-Figueroa,R. Villanueva-Arce andE. Durán-Páramo. 2021. Effective in vitrocontrol of two phytopathogens ofagricultural interest using cell-freeextracts of Pseudomonas fluorescensand chitosan. Molecules. 26: 6359.
Trivedi, P. andA. Pandey. 2008. Recovery of plant growth-promotingrhizobacteria from sodium alginate beads after 3 years following storage at 4 degrees C. J. Ind. Microbiol. Biotechnol. 35(3): 205–209.
van Elsas, J. D., J. T. Trevors, D. Jain, A. C. Wolters, C. E. Heijnen and L. S. van Overbeek. 1992. Survival of, and root colonization by, alginate-encapsulated Pseudomonas fluorescens cells following introduction into soil. Biol Fertil Soils. 14: 14–22.
Van Loon, L. C. and P. A. H. M. Bakker. 2006. Root-associatedbacteria inducing systemic resistance. In:Plant-associated bacteria (Eds. Gnanamanickam, S. S.), Springer, Dordrecht, 269–316
Voisard, C., C. Keel, D. Haas and G.Defago. 1989. Cyanideproduction by Pseudomonas fluorescens helps suppressblack root rot of tobacco under gnotobiotic conditions. EMBO J. 8:351–358.
Walsh, U.F., J. P. Morrissey and F. O’Gara. 2001.Pseudomonasfor biocontrol of phytopathogens: from functionalgenomics to commercial exploitation. Curr.Opin.Biotechnol. 12: 289–295.
Yaashikaa, P.R., P. S. Kumar, S. Varjani, S. Tamilselvi and A. Saravanan. 2020. Formulation and combinatorial effect of Pseudomonas fluorescens and Bacillus coagulans as biocontrol agents. Biocatal. Agric.Biotechnol. 30: 101868.
Zhang, L., S. E. Khabbaz, A. Wang, H. Li and P. A. Abbasi. 2015. Detection and characterization of broad‐spectrum antipathogen activity of novel rhizobacterial isolates and suppression of Fusarium crown and root rot disease of tomato. J Appl.Microbiol. 118(3): 685-703.