DOI: https://doi.org/10.56669/MEYQ8963
ABSTRACT
The aim of this study was to compare and evaluate the efficacy of arbuscular mycorrhizal fungi (AMF) in paddy when applied using drones as against the standard practice of manual application as soil drench during the transplanting stage. This study was conducted in Sungai Besar area in Selangor, which is one of the high yielding paddy areas in Malaysia. Although drone application of insecticides, fungicides and plant nutrients are common, well documented and practiced, there is no such studies for mycorrhiza application, whose benefits in aerobic and anaerobic paddies are well reported. To our knowledge, this is the first large scale study ever reported comparing effect of drone application of AMF with that of traditional manual application. Results clearly indicate that drone application of AMF is as good as manual soil drenching in terms of significantly increasing tiller production, grain yield and milling quality and hence could be a viable alternative method for application of arbuscular mycorrhizal fungi in paddy.
Key words: arbuscular mycorrhizal fungi, drone, rice tillers, grain yield
INTRODUCTION
Cereals such as rice, wheat and maize are the most important crops in the world, feeding billions of people every day. Among these, rice (Oryza sativa L.) occupies the top position, feeding more than 50% of the world’s population and accounting for 21% of global calorie intake while using only 11% of global cropland (FAO, 2019). It is the most important staple food crop in Asia, Africa and South and Central America as their main source of calorie intake. Globally, rice is grown in over 100 countries, with a total cultivated area of 162.06 million hectares and annual production of 495.78 million tons of milled rice or about 715 million tons of paddy rice (FAOSTAT, 2021). Paddy is also uniquely placed because it can grow in wet, anaerobic environments that other crops cannot grow or survive. It is considered as a semi-aquatic plant species that is well-adapted to a partially flooded environment. Low land rice, cultivated under submerged conditions, contributes more than 75% of global rice production, and the remainder is from upland rice grown under non-submerged conditions, mostly as rainfed (Maclean et al., 2013, Vallino et al., 2014).
Since the beginning of the 20th century, there has been a significant increase in agricultural yield, concurrent with demographic growth. Within one century, the population growth reached about 6.1 billion in 2000, from 1.5 billion in 1900, which is three times greater than during the entire history of humanity. Since 2003, the world’s population has increased by yet another billion, and given the current growth rates, it is projected to reach 9.4 –10 billion by 2050 (Carvalho, 2017; FAO, 2022). The increase in the world’s population in the 20th century could not have been possible without a parallel increase in food production. One of the major challenges of today’s world is the sustainable production of food for an ever-growing population, which is expected to reach 9.7 billion people by 2050 (United Nations, 2019). The Food and Agriculture Organization (FAO) predicts that food requirements for the year 2050 is estimated to be 50% higher to feed the increased world population which would be four times larger than the current level of production (FAO, 2022). The increase in yield of food commodities during and after the green revolution has been heavily reliant on chemical pesticides and mineral fertilizers (Liu et al., 2015), besides other factors, including improved plant varieties, use of machinery, reduced post-harvest losses etc. Synthetic fertilizers, however, are part of the world’s most energy-intensive production processes and are often dependent on finite resources (Woods et al., 2010). The extensive use of such synthetic fertilizers in food production systems is one of the major factors contributing to global greenhouse gas emissions (Vermeulen et al., 2012), and can have severe adverse effects on global warming, biodiversity, and environmental sustainability (Steffen et al., 2015). Furthermore, there is evidence that agrochemical-based food production systems have reached a plateau in productivity (Lobell et al., 2011; Grassini et al., 2013).
The sustainable intensification (SI) of agriculture is described as a series of practices that helps achieve “yield increase without adverse environmental impacts and without the cultivation of more land” (Royal Society, 2009). Due to the rapidly growing global population, SI in agriculture has gained more attention in recent years (Bello-Schünemann et al., 2017). Also, with around 40% of the world's terrestrial surface being transformed into agriculture (Ramankutty et al., 2008), as of 2018, only about 9% of the world's agricultural land had undergone SI (Pretty et al., 2018). Southeast Asia is a major rice-producing region of the world with a high level of internal consumption, accounting for 26% of rice production and 40% of global rice exports (FAO, 2021). Malaysia’s total rice production area is only about 0.7 million ha, contributing a mere 0.4% of the total global output, which is the lowest in Southeast Asia (Firdaus et al., 2020). Currently, the national rice production and consumption, or the self-sufficiency level staggers around 67 to 70%. To narrow the gap and to reduce the dependency on rice import, the government is targeting 75% rice self-sufficiency by 2025 under the 11th Five Year Malaysia Plan, and 80% self-sufficiency under the National Agrofood Policy 2021-2030 (Khazanah Research Institute, 2019). Closing the yield gap requires the concerted effort of policymakers, researchers, and extension services to facilitate farmers’ access to modern technologies for productivity enhancement.
There are several potential approaches for enhancing the yield and productivity as part of SI tools, of which the major intervention tools are developing high-yielding hybrid rice varieties through plant breeding, improved rice varieties towards biotic and abiotic stress tolerance, plant nutrition management, improved agronomic practices and also judicious use of beneficial biostimulants based on microbial sources (arbuscular mycorrhizal fungi, plant growth promoting rhizobacteria, Trichoderma etc.) as well as non-microbial biostimulant sources such as humic acids, chitosan, amino acids, protein hydrolysates, phosphites, seaweed extract etc. (Rouphael & Colla, 2020). All these tools form key components in sustainable agricultural practices.
The application of beneficial microbes in crop production has been suggested as a cornerstone for the next “Green Revolution” in agriculture (Baez-Rogelio et al., 2017). The use of arbuscular mycorrhizal fungi (AMF) in agriculture has increased in the last decade, due to the several benefits that they can provide to plants (Berruti et al., 2016). Results of studies of AMF in rice plants in the past have been inconsistent. It was often thought that AMF had difficulty forming symbiosis, especially in rice grown under anaerobic conditions, as flooding has generally exhibited a suppressive effect on AMF colonization in rice roots (Vallino et al., 2014; Zhang et al., 2015). Several studies have reported that AMF are rare or absent in rice roots in flooded paddy fields (Ilag et al., 1987; Lumini et al., 2011). On the other hand, several researchers have demonstrated the presence of AMF colonization in rice roots (Vallino et al., 2009; Wang et al., 2015; Chen et al., 2017; Bernaola et al., 2018). Several researches reported the beneficial impact of AMF inoculation on rice plant growth under flooding (e.g., Secilia and Bagyaraj, 1994; Bernaola et al., 2018; Iffah & Christopher, 2021) and non-flooding conditions (e.g., Corrêa et al., 2014; Sánchez et al., 2015; Zhang et al., 2015). Most studies reported that AMF increased rice plant biomass, grain yield, and P uptake under flooding conditions (Secilia and Bagyaraj, 1994; Solaiman and Hirata, 1997; Gewaily, 2019). Our earlier study reported that AMF colonization in rice plants has significantly enhanced tiller production and grain yield, both in direct seeded and transplanted paddy grown under anaerobic condition (Iffah & Christopher, 2021). The integration of mycorrhizae in sustainable and organic farming practices aligns with global trends towards environmentally conscious agriculture, offering a means to reduce chemical inputs, enhance soil biodiversity, and ensure long-term soil fertility.
Mechanization has played an imperative role in improving the cropping intensity and farm productivity in recent times. In paddy cultivation, one of the biggest problems faced by the farmers is the shortage of labor. Unmanned aerial vehicles or drones are emerging as a counter measure for the continuously decreasing agricultural labor population. Today, agricultural drones support a wide range of farming applications. They can perform tasks such as crop monitoring, yield estimation, plant census, weed identification, and mapping of field boundaries. Paddy farming has traditionally been a very labor-intensive activity. In recent years, unmanned aerial vehicles or drones have become an important tool for precision agriculture and paddy is no exception. In Malaysia, drone technology for agriculture is mainly employed in private sectors, especially in the plantation sector. Although the drone industry had established a broad niche of application in agricultural systems in plantation crops, especially oil palm, the impact on Malaysian rice farmers is relatively low. Currently, drones are used to facilitate timely application of fertilizer and plant protection chemicals (Dorairaj & Govender, 2023). As a part of sustainable rice production studies, the purpose of this study is to investigate whether AM fungi can be applied in transplanted paddy using drones and if possible, whether the performance of drone sprayed mycorrhizal inoculants is comparable to the traditional soil drench application in increasing the plant growth and grain yield.
MATERIALS AND METHODS
This study was conducted during the period September to December 2023, in paddy variety MR297, which is a popular variety released by Malaysian Agricultural Research & Development Institute. The experimental site is in Sungai Besar, Selangor situated in the Barat Laut Paddy Project and considered as one of the most important rice production areas in Malaysia. The area was selected due to its plain flat coastal under the Integrated Agricultural Development Authority (IADA) and considered as rice granary of the state of Kuala Selangor. The soil is considered as coastal soil, with mean soil pH 5.5, while the mean organic carbon content is more than 2%. The mean concentration of total nitrogen is 0.25%, available P > 40 mg kg-1 and exchangeable K > 0.1 cmol kg-1. It is located at 3°42′59.0976″N and 101°1′8.382″E latitude. Twenty-five days old paddy seedlings were planted at 2 seedlings per hill with a spacing of 25 × 16.5 cm to accommodate 24 hills per metre square. Good field cultural management and practices were followed during the experiment.
Commercially available AMF consortium (sample provided by Mycorrhizal Applications, USA) containing 900 propagules per gram of formulated product was used in this experiment. This mixture, a sprayable formulation, consists of four endomycorrhizal fungi namely Rhizophagus irregularis, Glomus aggregatum, Funneliformis mosseae, and Claroideoglomus etunicatum containing spores, hyphae and colonized root fragments and a blend of humic acids and seaweed extract as carrier. Our earlier studies have confirmed that soil drench of this formulation at 125 g (112,500 propagules) per ha, enhanced crop growth and yield of paddy when used for direct seeded and transplanted paddy (Iffah & Christopher, 2021) and found to be the best among different application rates tested. Therefore, we used the same application rate of 125 g of product per ha for this study. The experiment had 3 treatments and each treatment had 6 replications with the plot size of 3,000 sq. meter.
Treatment-1: Untreated control.
Treatment-2: AMF consortium applied as soil drench using conventional sprayer.
Treatment-3: AMF consortium application using drones.
Conventional backpack sprayer was used for application of AMF in Treatment-2, at a spray/soil drench volume of 200 L per ha. For drone application, we used model Sprayer Drone E616S. Field water level at transplanting was kept minimum during AMF application stage. Buffer rows (6 planting rows) were maintained between the plots on all the four sides to prevent drifting effect due to drone application.
The fertilizer application of nitrogen, phosphorous and potassium was 275, 66 and 215 kg per ha, respectively. Fifty percent of total amount fertilizer was applied as basal fertilizer during the land preparation; the remaining amount of 50% fertilizer was applied in four split applications, at 10, 20, 65 and 90 DAT. Insect pests, diseases and weeds were closely monitored and controlled timely using approved chemicals. Flag leaf width (cm) and flag leaf length (cm) were measured on the main stem of 15 uniform plants in each replication. Flag leaf area (cm2) was measured one week before harvest and calculated following the formula adapted by Yoshida et al. (1976) as follows:
Flag leaf area (cm2) = Leaf length (cm) × Maximum width (cm)
The experimental plots were harvested leaving the border rows to avoid border effect. Before harvesting the whole plot, the number of total tillers and productive tillers per plant had been determined in 6 sub plots (consist of one square meter) of each plot, and tillers of 15 plants per plot were separated into categories according to the marked hang tag, and examined for panicle length, 1,000-grain weight, seed setting percentage, and numbers of filled and unfilled grains per panicle separately. Subsequently, the entire crop was harvested from the net plot area and the grain was hand threshed, winnowed and sun dried to bring the moisture content to 14 per cent and the yield was recorded net plot wise and computed to kg ha-1. Assessment on grain weight and milling as per Rice Knowledge Bank (IRRI, 2010) were carried at the Laboratory of Food and Security, Universiti Putra Malaysia, Selangor. Analysis of variance were calculated to determine the effect of treatments on the response to variables and treatment means were compared by Tukey test, using Minitab Version 18 test.
RESULTS
Rice tillers are specialized branches bearing panicles and provide nutrients for panicle development (Hanada, 1993; Wang & Li, 2011). Number of tillers and the quality of their growth directly determine the number and development of panicles, and ultimately affect the yield of rice. In our experiment, AMF application by drones and conventional sprayer application, significantly increased the total number of tillers and the number of productive tillers (Table 1). However, there is no significant difference in the number of unproductive tillers among treatments. Unproductive tillers do not produce panicles and often die, and hence are considered at best as useless, or as competitive for resources such as solar energy, assimilates, and nutrients that are important for the productive tillers.
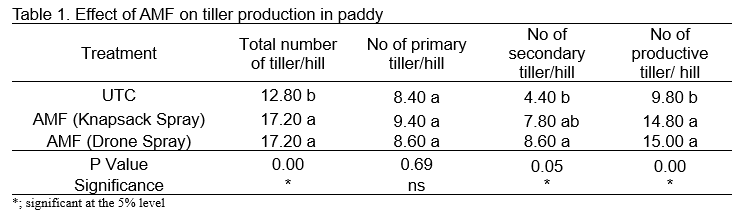
Flag leaf characteristics of different treatments are presented in Table 2. We could not find any significant difference in flag leaf length, width, and the flag leaf area among all the treatments.
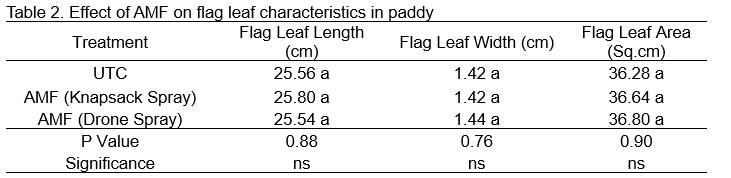
Spikelet number per panicle (SNPP) is one of the most important yield components used to estimate rice yields. The typical rice spikelet contains a single fertile floret and produces only one grain. There is no significant difference in number of spikelets among different treatments (Table 3). Similarly, there is no significant change in filled grains and percentage of filled grains, although numerically higher percentage was recorded in AMF treated plots (74.63% to 76.69%) as against only 67.69% in untreated plants.
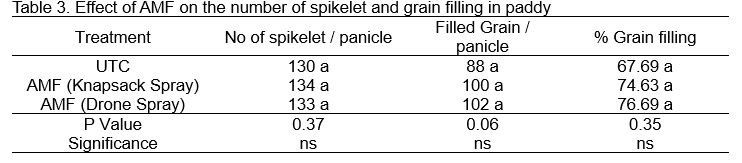
Thousand grain weight (TGW) is an important factor that influences the yield of rice. Increasing TGW can lead to higher rice yields, which is crucial for overall grain yield. Several studies have shown that TGW is positively correlated with grain yield per hectare (Gayathri & Premalatha, 2023). In this experiment, we could not see any significant difference in TGW among treatments, although there is a numerical increase in treatment received AMF application (Table 4). However, significant grain yield increase was recorded in AMF treated plots. Interestingly, highest grain yield increase was recorded in plot received AMF application by drones. Grain yield increase of 10.89% and 12.98 % was recorded in plot received AMF inoculation by knapsack sprayer and drone application, respectively.
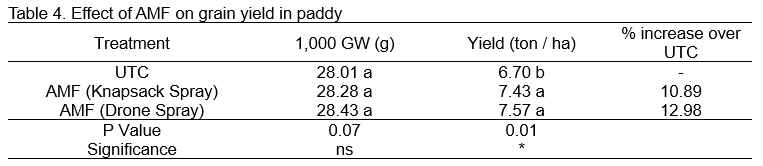
Freshly harvested rice grains, known as “paddy” or “rough” rice, are usually milled before consumption. Paddy typically comprises about 20% husk, 11% bran and 69% starchy endosperm. Milling involves de-hulling the paddy and segregating them as whole (head) rice and broken rice. Several factors potentially affect milling and the yield of head rice. Head rice is used for human consumption, while broken kernels are used for animal feed, rice flour, and the brewing industry (Esa et al., 2013). In this experiment, AMF application seem to have positively impacted milling which resulted in significantly higher head rice percentage. Lowest percentage of head rice (66.93%) was recorded in UTC and highest percentage of 73.18% was recorded in AMF applied using backpack sprayer. Interestingly, there is significant difference in head rice percentage between drone application and conventional backpack application.
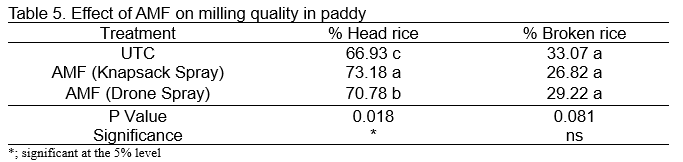
DISCUSSION
Tillering in rice is an important agronomic trait for grain production and a key component of yield for major cereals such as wheat, rice, barley and rye (Sakamoto and Matsuoka, 2004; Sreenivasulu and Schnurbusch, 2012). Productive tillers are derived from tillers that have more than two green leaves or are longer than two-thirds of the plant length at the maximum tillering stage (Matsushima, 1959). Unproductive tillers do not produce panicles and often die, and hence are considered at best as useless, or as competitor for resources such as solar energy, assimilates, and nutrients that are important for the productive tillers; thus, fewer unproductive tillers are desired in rice cultivation and breeding in order to achieve a high yield (Khush, 1995; Ohe and Mimoto, 2002). In the present study, AMF treated plants produced significantly higher number of productive tillers, which is similar results recorded earlier by Nadjilom et al., (2020) and Iffah & Christopher (2021).
Microbial inoculants are an essential building block for resilient and sustainable food production systems and have the potential to increase farm productivity in a sustainable way. Their use reinforces various global challenges and sustainable development goals, such as food safety, food security, and climate change mitigation (D’Hondt et al., 2021). There are not too many studies on AMF symbiosis on rice mainly because rice is mostly grown in waterlogged anaerobic conditions, which usually do not favor AMF colonization. Paddy is reported to have derived from a semi-aquatic ancestor with unique characteristics, and it can regulate AMF colonization under waterlogged conditions by influencing root architecture, anatomy, and physiology, even though AMF are obligate aerobes. In such conditions, rice plants develop aerenchymatous (also known as aeriferous parenchyma) spongy tissues that enable AMF to exchange gases in the atmosphere (Lumini et al., 2011; Kalamulla et al., 2022). Nevertheless, the symbiosis of AMF and rice plants has been reported over a period of time (Secilia and Bagyaraj, 1994; Wangiyana et al., 2006; Lumini et al., 2011; Watanarojanaporn et al., 2013; Vallino et al., 2014). For instance, the system of rice intensification promotes root colonization and diversity of AMF species in rice roots compared to conventional rain-fed rice cultivation systems (Watanarojanaporn et al., 2013). AMF are naturally more abundant in low-input farming and under aerobic conditions than under partly anaerobic and submerged conditions (Lumini et al., 2011; Vallino et al., 2014). While studying on transplanted paddy, Solaiman & Hirata (1997) conclude that the AMF inoculation at the nursery stage under both dry and wet conditions increased growth, grain yield and nutrient acquisition of wetland rice under field conditions. In the present study, inoculated AMF paddy plants produced higher grain yield which was 11-13% higher than those of not inoculated plants. These findings are like previous studies by Zhang et al., (2014) and Iffah & Christopher (2021) where AMF had a significant effect on production and productivity of paddy.
The shortage of labor, especially seasonal workers, has become a common problem and pushed up the labor cost in agricultural production. By leveraging drone technology, farmers can optimize their operations, reduce labor-intensive tasks, and achieve cost-savings while reducing the overall rice production cost and productivity. Results of the present study precisely confirm that AMF could be applied with drones, and we can lower the cost of application.
USE OF DRONES IN RICE CULTIVATION IN MALAYSIA
The use of drones in rice cultivation in Malaysia began gaining traction in the mid-2010s, driven by efforts to modernize agriculture and address challenges like labor shortages and the need for precision. Initially introduced through pilot projects by the Malaysian Agricultural Research and Development Institute (MARDI) and tech companies, drones quickly showcased their ability to efficiently apply fertilizers and pesticides while monitoring crop health. By 2018, drone adoption expanded under government-backed smart farming initiatives, particularly in rice-producing regions like the Muda Agricultural Development Authority (MADA). By 2021, drones had become mainstream, with their use in rice farming further encouraged by government subsidies, private partnerships, and improved technology.
In MADA, popular drone models include the DJI Agras T20 Pro, DJI Agras T30, and DJI Agras MG-1P. The T20 Pro, priced between RM30,000 (US$6,723) and RM35,000 (US$7,843), is favored for large-scale operations with its 20-liter tank. The T30, costing RM50,000 (US$11,205) to RM55,000 (US$12,325), offers even greater capacity, while the more affordable MG-1P, priced at RM25,000 (US$5,602) to RM28,000 (US$6,290), remains reliable for smaller operations. These drones are prized for their ability to cover large fields efficiently, reducing labor costs and improving operational speed.
Drones have proven highly effective in rice farming, capable of covering 10–16 hectares per day. The precision of drone spraying ensures uniform application, reducing pesticide and fertilizer use by 15–30%. Additionally, drones can spray a hectare in 15-20 minutes, whereas manual spraying takes several hours. This efficiency reduces reliance on labor and helps mitigate labor shortages. Drones also offer improvements in yield, with some reports suggesting a 5-10% increase due to better pest and disease management.
Overall, drones have become an essential tool in modernizing rice farming in Malaysia, offering increased efficiency, reduced costs, and improved sustainability in the sector.
The economic comparison of using drones versus manual spraying for rice cultivation in the Muda Agricultural Development Authority (MADA) region highlights significant cost savings and increased profits. When comparing total costs per hectare, manual spraying incurs RM1,900 (US$426), while drone spraying costs RM1,550(US$366), resulting in a savings of RM350(US$78) per hectare. Revenue from rice production, assuming an average yield of 4 metric tons per hectare at a market price of RM1,500 (US$336) per metric ton, is estimated at RM6,000(US$1,345) per hectare.
The use of drones offers economic benefits by saving on labor costs, reducing spraying time, and improving resource efficiency. Over the course of a farming season, the savings per hectare can add up significantly, especially for larger farms. With precise and efficient operations, drones contribute to more consistent yields, making them a profitable long-term investment in rice farming.
CONCLUSION
Use of bio-fertilizer or microbial biostimulant such as AMF is one of the important productivity enhancement supplements which could be used in a sustainable way to increase rice grains and head rice yields. Results of this study also clearly indicate that sprayable AMF formulation could be applied using drones and it is as good as manual soil drenching and hence could be a viable alternative method for application of arbuscular mycorrhizal fungi in paddy. To our knowledge, this is the first ever study reporting on the use of drones for application of AMF inoculants in paddy.
REFERENCES
Baez-Rogelio, A.; Morales-García, Y.E.; Quintero-Hernández, V.; Muñoz-Rojas, J. Next generation of microbial inoculants for agriculture and bioremediation. 2017. Microb. Biotechnol, 10, 19–21.
Bello-Schünemann, J., Cilliers, J., Donnenfeld, Z., Aucoin, C., and Porter, A. 2017. African Futures—Key Trends to 2035. Pretoria: Institute for Security Studies. Policy brief. doi: 10.2139/ssrn.3099362.
Berruti, A., Lumini, E., Balestrini, R and Bianciotto, V. 2016. Arbuscular Mycorrhizal Fungi as Natural Biofertilizers: Let's Benefit from Past Successes. Frontiers in Microbiology. 6:1559. doi: 10.3389/fmicb.2015.01559.
Bernaola L., Cange G., Way M. O., Gore J., Hardke J., Stout M. 2018. Natural colonization of rice by arbuscular mycorrhizal fungi in different production areas. Rice Sci. 25 169–174. 10.1016/j.rsci.2018.02.006.
Carvalho F.P. 2017. Pesticides, environment, and food safety. Food Energy Secur. 6:48–60. doi: 10.1002/fes3.108.
Chen, S., Zhao, H., Zou, C., Li, Y., Chen, Y., Wang, Z. & Ahammed, G. J. 2017. Combined inoculation with multiple arbuscular mycorrhizal fungi improves growth, nutrient uptake and photosynthesis in cucumber seedling. Front Microbiol., 8 (2516), 1-11. https://doi.org/10.3389/fmicb.2017.02516
Corrêa, A., Cruz, C., Pérez-Tienda, J., and Ferrol, N. 2014. Shedding light onto nutrient responses of arbuscular mycorrhizal plants: nutrient interactions may lead to unpredicted outcomes of the symbiosis. Plant Sci. 221, 29–41. doi:10.1016/j.plantsci.2014.01.009
D’Hondt, K., Kostic, T., McDowell, R., Eudes, F., Singh, B.K., Sarkar, S., Markakis, M., Schelkle, B., Maguin, E., and Sessitsch, A. 2021. Microbiome innovations for a sustainable future. Nat. Microbiol. 6, 138–142. https://doi.org/10.1038/.
Dorairaj, D.; Govender, N.T. 2023. Rice and paddy industry in Malaysia: Governance and policies, research trends, technology adoption and resilience. Front. Sustain. Food Syst. 7, 1093605.
Esa, N.M.; Ling, T.B.; Peng, L.S. By-products of Rice Processing. 2013. An Overview of Health Benefits and Applications. Rice Res. Open Access, 1, 1–11.
FAO. FAOSTAT. 2019. Production Data www.fao.org/faostat/en/#data.
FAOSTAT. 2021. Production Data www.fao.org/faostat/en/#data.
FAO. 2022. The State of Food Security and Nutrition in the World 2022. https://www.fao.org/3/cc0639en/cc0639en.
Firdaus, R. B. R., Tan, M. L., Rahmat, S. R., & Gunaratne, S. M. 2020. Paddy, rice and food security in Malaysia: A review of climate change impacts. Cogent Social Sciences, 6, 1-17. https://doi.org/10.1080/23311886.2020.1818373
Gayahtri, N. K. & Premalatha, Y, 2023. Studies on Correlation and Path Analysis in Medium Duration Rice Varieties of Andhra Pradesh, India. Emerging Issues in Agricultural Sciences Vol. 2, 25: 77-88.
Gewaily E. E. (2019). Influence of arbuscular mycorrhizal fungi (AMF) inoculation on the performance of Sakha 107 rice cultivar under different irrigation intervals. Env. Biodiv. Soil Security 3 119–129. 10.21608/jenvbs.2019.15637.1068.
Grassini, P., Eskridge, K. M. & Cassman, K. G. Distinguishing between yield advances and yield plateaus in historical crop production trends. Nat. Commun. 4, 2918 (2013).
Hanada, K. 1993. Tillers. 1. Plant growth and tillering. In: Matsuo T, Hoshikawa K. eds. Science of the rice plant, vol. 1. Tokyo: Food and Agriculture Policy Research Center, 222–229
Iffah, H & Christopher, M, (2021) Effect of in vitro Produced Arbuscular Mycorrhizal Fungi Inoculum on Anaerobic Direct Seeded and Transplanted Paddy. Journal of Agricultural Science; Vol. 13, No. 6; 18-30.
Ilag, L. L., Rosales, A. M., Elazegui, F. A., & Mew, T. W. (1987). Changes in the population of infective endomycorrhizal fungi in a rice-based cropping system. Plant and Soil, 103, 67-73. https://doi.org/10.1007/BF02370669
IRRI. (2010). Rice Knowledge Bank. Retrieved from https://www.knowledgebank.irri.org.
Kalamulla, R., Sandaruwan, D., Karunarathna, S. C., Stephenson, S. L., Tibpromma, S., Elgorban, A. M., Salim Al-Rejaie, Yapa, P. N. and Suwannarach, N. (2022) Assessment of Community Dynamics of Arbuscular Mycorrhizal Fungi in the Rice (Oryza sativa L.) Rhizosphere and Potential Application as Biofertilizer. Sustainability 14, 16537. https://doi.org/10.3390/su142416537.
Khazanah Research Institute, 2019. The Status of the Paddy and Rice Industry in Malaysia. pp 220.
Khush GS. 1995. Breaking the yield frontier of rice. GeoJournal 35, 329–332.
Y. Liu, X. Pan, J. Li A 1961–2010 record of fertilizer use, pesticide application and cereal yields: a review. Agron. Sustain. Dev., 35 (2015), pp. 83-93, 10.1007/s13593-014-0259-9.
Lobell, D. B. & Gourdji, S. M. 2011. The Influence of Climate Change on Global Crop Productivity. Plant Physiology, Vol. 160, pp. 1686–1697.
Lumini, E., Vallino, M., Alguacil, M. M., Romani, M., & Bianciotto, V. (2011). Different farming and water regimes in Italian rice fields affect arbuscular mycorrhizal fungal soil communities. Ecol Appl, 21(5), 1696-1707. https://doi.org/10.1890/10-1542.1
Maclean, J., Hardy, B. and Hettel, G. 2013. Rice Almanac: Source book for one of the most important economic activities on earth. IRRI.
Matsushima S. 1959. Theories and techniques in rice cultivation – theory on yield analysis and its application. Tokyo: Yokendo. [In Japanese.]
Nadjilom, Y., Takoukam, S., Toukam, Issa, M. & Ngakou. A. 2020. Field Evaluation of Growth and Yield of Two Local Rice Varieties (Tox-728-1 and Madjitolngar) in Response to Indogenous Mycorrhizal Inoculation in South-Chad. American Journal of Plant Sciences, 11, 1175-1192. www.scirp.org/journal/ajps.
Ohe M, Mimoto H. (2002) Appropriate time of deep-water treatment for regulation of rice growth. Japanese Journal of Crop Science 71, 335–342. [In Japanese.]
Pretty, J., Benton, T. G., Bharucha, Z. P., Dicks, L. V., Butler Flora, C., Godfray, H. C. J., et al. (2018). Global assessment of agricultural system redesign for sustainable intensication. Nat Sustain 1, 441–446. doi: 10.1038/s41893-018-0114-0.
Ramankutty, N., Evan, A. T., Monfreda, C., and Foley, J. A. (2008). Farming the planet: 1. Geographic distribution of global agricultural lands in the year 2000. Glob Biogeochem. Cycles 22. doi: 10.1029/2007gb002952.
Rouphael, Y. & Colla, G. (2020) Biostimulants in agriculture – Editorial. Frontiers in Plant Science. 11 (40) 1-7.
Royal Society (2009) Geoengineering the climate: science, governance, and uncertainty. London: Royal Society. http://royal society.org/policy/Publications/2009/geoengineering climate.
Sakamoto, T. & Matsuoka, M. (2004) Generating high-yielding varieties by genetic manipulation of plant architecture. Curr. Opin. Biotech. 15, 144-147. DOI:10.1016/j.copbio.2004.02.003.
Sánchez, M. R., Baños, Y. S., Hernández, Y. M., Martínez, A. Y., Benitez, M., Bharat, B. V. and Chávez, Y. P. (2015) Arbuscular mycorrhizal symbiosis in rice (Oryza sativa L.) plants in flooded and non-flooded conditions. Acta Agronómica. 64 (3), p 211-217. doi: http:// dx.doi.org/10.15446/acag.v64n3.43087.
Secilia J., Bagyaraj D. J. (1994). Selection of efficient vesicular-arbuscular mycorrhizal fungi for wetland rice-a preliminary screen. Mycorrhiza 4 265–268. 10.1007/s005720050031.
Solaiman, M., Hirata, H. Effect of arbuscular mycorrhizal fungi inoculation of rice seedlings at the nursery stage upon performance in the paddy field and greenhouse. Plant and Soil 191, 1–12 (1997). https://doi.org/10.1023/A:1004238028617.
Sreenivasulu, N., Schnurbusch, T.. 2012. A genetic playground for enhancing grain number in cereals. Trends Plant Sci. 17(2): 91–101. doi: 10.1016/j.tplants.2011.11.003 http://gateway.webofknowledge.com/gateway/Gateway.cgi?GWVersion=2&SrcApp=PARTNER_APP&SrcAut.
Steffen, W., Richardson, K., Rockström, J., Cornell, S.E., Fetzer, I., Bennett, E.M., Biggs, R., Carpenter, S.R., de Vries, W., de Wit, C.A., et al. Sustainability, Planetary boundaries: guiding human development on a changing planet. Science, 347 (2015), p. 1259855, 10.1126/science.1259855.
United Nations World population prospects 2019: Highlights Dep. Econ. Soc. Aff. (2019).
United Nations. 2022. World population prospects 2022: summary of results. New York: United Nations, Department of Economic and Social Affairs, Population Division.
Vallino, M.; Greppi, D.; Novero, M.; Bonfante, P.; Lupotto, E. Rice root colonisation by mycorrhizal and endophytic fungi in aerobic soil. Ann. Appl. Biol. 2009, 154, 195–204.
Vallino, M.; Fiorilli, V.; Bonfante, P. Rice flooding negatively impacts root branching and arbuscular mycorrhizal colonization, but not fungal viability. Plant Cell Environ. 2014, 37, 557–572.
Vermeulen, S.J., Campbell, B.M. & Ingram, J.S.I. (2012) Climate change and food systems. Annu. Rev. Environ. Resour., 37, pp. 195-222, 10.1146/annurev-environ-020411-130608.
Wang P., Jiang H., Boeren S., Dings H., Kulikova O., Bisseling T., et al. (2021). A nuclear-targeted effector of Rhizophagus irregularis interferes with Histone 2B mono-ubiquitination to promote arbuscular mycorrhization. New Phytol. 230 1142–1155. 10.1111/nph.17236.
Wang, Y., & Li, J. (2011). Branching in rice. Current Opinion in Plant Biology, 14(1), 94–99. https://doi.org/10.1016/j.pbi.2010.11.002.
Wang Y., Li T., Li Y., Björn L. O., Rosendahl S., Olsson P. A., et al. (2015). Community dynamics of arbuscular mycorrhizal fungi in high-input and intensively irrigated rice cultivation systems. Appl. Environ. Microb. 81 2958–2965. 10.1128/aem.03769-14.
Wangiyana W, Cornish P.S, Morris E.C. Arbuscular mycorrhizal fungi dynamics in contrasting cropping systems on vertisol and regosol soils of Lombok, Indonesia. Exp Agric. 2006;42(4):427–439. doi: 10.1017/S0014479706003826.
Watanarojanaporn, N.; Boonkerd, N.; Tittabutr, P.; Longtonglang, A.; Young, J.P.W.; Teaumroong, N. Effect of rice cultivation systems on indigenous arbuscular mycorrhizal fungal community structure. Microbes Environ. 2013, 28, 316–324.
Woods, J., A. Williams, J.K. Hughes, M. Black, R. Murphy Energy and the food system. Philos. Trans. R. Soc. Lond., 365 (2010), pp. 2991-3006, 10.1098/rstb.2010.0172.
Zhang, S.; Wang, L.; Ma, F.; Bloomfield, K.J.; Yang, J.; Atkin, O.K. Is Resource Allocation and Grain Yield of Rice Altered by Inoculation with Arbuscular Mycorrhizal Fungi? J. Plant Ecol. 2014, 8, 436–448.
Zhang S., Wang L., Ma F., Bloomfield K. J., Yang J., Atkin O. K. (2015). Is resource allocation and grain yield of rice altered by inoculation with arbuscular mycorrhizal fungi? J. Plant Ecol. 8 436–448. 10.1093/jpe/rtu025.
Use of Agricultural Drones for Application of Arbuscular Mycorrhizal Fungi Inoculants on Transplanted Rice Seedlings and Its Performance on Growth and Productivity
DOI: https://doi.org/10.56669/MEYQ8963
ABSTRACT
The aim of this study was to compare and evaluate the efficacy of arbuscular mycorrhizal fungi (AMF) in paddy when applied using drones as against the standard practice of manual application as soil drench during the transplanting stage. This study was conducted in Sungai Besar area in Selangor, which is one of the high yielding paddy areas in Malaysia. Although drone application of insecticides, fungicides and plant nutrients are common, well documented and practiced, there is no such studies for mycorrhiza application, whose benefits in aerobic and anaerobic paddies are well reported. To our knowledge, this is the first large scale study ever reported comparing effect of drone application of AMF with that of traditional manual application. Results clearly indicate that drone application of AMF is as good as manual soil drenching in terms of significantly increasing tiller production, grain yield and milling quality and hence could be a viable alternative method for application of arbuscular mycorrhizal fungi in paddy.
Key words: arbuscular mycorrhizal fungi, drone, rice tillers, grain yield
INTRODUCTION
Cereals such as rice, wheat and maize are the most important crops in the world, feeding billions of people every day. Among these, rice (Oryza sativa L.) occupies the top position, feeding more than 50% of the world’s population and accounting for 21% of global calorie intake while using only 11% of global cropland (FAO, 2019). It is the most important staple food crop in Asia, Africa and South and Central America as their main source of calorie intake. Globally, rice is grown in over 100 countries, with a total cultivated area of 162.06 million hectares and annual production of 495.78 million tons of milled rice or about 715 million tons of paddy rice (FAOSTAT, 2021). Paddy is also uniquely placed because it can grow in wet, anaerobic environments that other crops cannot grow or survive. It is considered as a semi-aquatic plant species that is well-adapted to a partially flooded environment. Low land rice, cultivated under submerged conditions, contributes more than 75% of global rice production, and the remainder is from upland rice grown under non-submerged conditions, mostly as rainfed (Maclean et al., 2013, Vallino et al., 2014).
Since the beginning of the 20th century, there has been a significant increase in agricultural yield, concurrent with demographic growth. Within one century, the population growth reached about 6.1 billion in 2000, from 1.5 billion in 1900, which is three times greater than during the entire history of humanity. Since 2003, the world’s population has increased by yet another billion, and given the current growth rates, it is projected to reach 9.4 –10 billion by 2050 (Carvalho, 2017; FAO, 2022). The increase in the world’s population in the 20th century could not have been possible without a parallel increase in food production. One of the major challenges of today’s world is the sustainable production of food for an ever-growing population, which is expected to reach 9.7 billion people by 2050 (United Nations, 2019). The Food and Agriculture Organization (FAO) predicts that food requirements for the year 2050 is estimated to be 50% higher to feed the increased world population which would be four times larger than the current level of production (FAO, 2022). The increase in yield of food commodities during and after the green revolution has been heavily reliant on chemical pesticides and mineral fertilizers (Liu et al., 2015), besides other factors, including improved plant varieties, use of machinery, reduced post-harvest losses etc. Synthetic fertilizers, however, are part of the world’s most energy-intensive production processes and are often dependent on finite resources (Woods et al., 2010). The extensive use of such synthetic fertilizers in food production systems is one of the major factors contributing to global greenhouse gas emissions (Vermeulen et al., 2012), and can have severe adverse effects on global warming, biodiversity, and environmental sustainability (Steffen et al., 2015). Furthermore, there is evidence that agrochemical-based food production systems have reached a plateau in productivity (Lobell et al., 2011; Grassini et al., 2013).
The sustainable intensification (SI) of agriculture is described as a series of practices that helps achieve “yield increase without adverse environmental impacts and without the cultivation of more land” (Royal Society, 2009). Due to the rapidly growing global population, SI in agriculture has gained more attention in recent years (Bello-Schünemann et al., 2017). Also, with around 40% of the world's terrestrial surface being transformed into agriculture (Ramankutty et al., 2008), as of 2018, only about 9% of the world's agricultural land had undergone SI (Pretty et al., 2018). Southeast Asia is a major rice-producing region of the world with a high level of internal consumption, accounting for 26% of rice production and 40% of global rice exports (FAO, 2021). Malaysia’s total rice production area is only about 0.7 million ha, contributing a mere 0.4% of the total global output, which is the lowest in Southeast Asia (Firdaus et al., 2020). Currently, the national rice production and consumption, or the self-sufficiency level staggers around 67 to 70%. To narrow the gap and to reduce the dependency on rice import, the government is targeting 75% rice self-sufficiency by 2025 under the 11th Five Year Malaysia Plan, and 80% self-sufficiency under the National Agrofood Policy 2021-2030 (Khazanah Research Institute, 2019). Closing the yield gap requires the concerted effort of policymakers, researchers, and extension services to facilitate farmers’ access to modern technologies for productivity enhancement.
There are several potential approaches for enhancing the yield and productivity as part of SI tools, of which the major intervention tools are developing high-yielding hybrid rice varieties through plant breeding, improved rice varieties towards biotic and abiotic stress tolerance, plant nutrition management, improved agronomic practices and also judicious use of beneficial biostimulants based on microbial sources (arbuscular mycorrhizal fungi, plant growth promoting rhizobacteria, Trichoderma etc.) as well as non-microbial biostimulant sources such as humic acids, chitosan, amino acids, protein hydrolysates, phosphites, seaweed extract etc. (Rouphael & Colla, 2020). All these tools form key components in sustainable agricultural practices.
The application of beneficial microbes in crop production has been suggested as a cornerstone for the next “Green Revolution” in agriculture (Baez-Rogelio et al., 2017). The use of arbuscular mycorrhizal fungi (AMF) in agriculture has increased in the last decade, due to the several benefits that they can provide to plants (Berruti et al., 2016). Results of studies of AMF in rice plants in the past have been inconsistent. It was often thought that AMF had difficulty forming symbiosis, especially in rice grown under anaerobic conditions, as flooding has generally exhibited a suppressive effect on AMF colonization in rice roots (Vallino et al., 2014; Zhang et al., 2015). Several studies have reported that AMF are rare or absent in rice roots in flooded paddy fields (Ilag et al., 1987; Lumini et al., 2011). On the other hand, several researchers have demonstrated the presence of AMF colonization in rice roots (Vallino et al., 2009; Wang et al., 2015; Chen et al., 2017; Bernaola et al., 2018). Several researches reported the beneficial impact of AMF inoculation on rice plant growth under flooding (e.g., Secilia and Bagyaraj, 1994; Bernaola et al., 2018; Iffah & Christopher, 2021) and non-flooding conditions (e.g., Corrêa et al., 2014; Sánchez et al., 2015; Zhang et al., 2015). Most studies reported that AMF increased rice plant biomass, grain yield, and P uptake under flooding conditions (Secilia and Bagyaraj, 1994; Solaiman and Hirata, 1997; Gewaily, 2019). Our earlier study reported that AMF colonization in rice plants has significantly enhanced tiller production and grain yield, both in direct seeded and transplanted paddy grown under anaerobic condition (Iffah & Christopher, 2021). The integration of mycorrhizae in sustainable and organic farming practices aligns with global trends towards environmentally conscious agriculture, offering a means to reduce chemical inputs, enhance soil biodiversity, and ensure long-term soil fertility.
Mechanization has played an imperative role in improving the cropping intensity and farm productivity in recent times. In paddy cultivation, one of the biggest problems faced by the farmers is the shortage of labor. Unmanned aerial vehicles or drones are emerging as a counter measure for the continuously decreasing agricultural labor population. Today, agricultural drones support a wide range of farming applications. They can perform tasks such as crop monitoring, yield estimation, plant census, weed identification, and mapping of field boundaries. Paddy farming has traditionally been a very labor-intensive activity. In recent years, unmanned aerial vehicles or drones have become an important tool for precision agriculture and paddy is no exception. In Malaysia, drone technology for agriculture is mainly employed in private sectors, especially in the plantation sector. Although the drone industry had established a broad niche of application in agricultural systems in plantation crops, especially oil palm, the impact on Malaysian rice farmers is relatively low. Currently, drones are used to facilitate timely application of fertilizer and plant protection chemicals (Dorairaj & Govender, 2023). As a part of sustainable rice production studies, the purpose of this study is to investigate whether AM fungi can be applied in transplanted paddy using drones and if possible, whether the performance of drone sprayed mycorrhizal inoculants is comparable to the traditional soil drench application in increasing the plant growth and grain yield.
MATERIALS AND METHODS
This study was conducted during the period September to December 2023, in paddy variety MR297, which is a popular variety released by Malaysian Agricultural Research & Development Institute. The experimental site is in Sungai Besar, Selangor situated in the Barat Laut Paddy Project and considered as one of the most important rice production areas in Malaysia. The area was selected due to its plain flat coastal under the Integrated Agricultural Development Authority (IADA) and considered as rice granary of the state of Kuala Selangor. The soil is considered as coastal soil, with mean soil pH 5.5, while the mean organic carbon content is more than 2%. The mean concentration of total nitrogen is 0.25%, available P > 40 mg kg-1 and exchangeable K > 0.1 cmol kg-1. It is located at 3°42′59.0976″N and 101°1′8.382″E latitude. Twenty-five days old paddy seedlings were planted at 2 seedlings per hill with a spacing of 25 × 16.5 cm to accommodate 24 hills per metre square. Good field cultural management and practices were followed during the experiment.
Commercially available AMF consortium (sample provided by Mycorrhizal Applications, USA) containing 900 propagules per gram of formulated product was used in this experiment. This mixture, a sprayable formulation, consists of four endomycorrhizal fungi namely Rhizophagus irregularis, Glomus aggregatum, Funneliformis mosseae, and Claroideoglomus etunicatum containing spores, hyphae and colonized root fragments and a blend of humic acids and seaweed extract as carrier. Our earlier studies have confirmed that soil drench of this formulation at 125 g (112,500 propagules) per ha, enhanced crop growth and yield of paddy when used for direct seeded and transplanted paddy (Iffah & Christopher, 2021) and found to be the best among different application rates tested. Therefore, we used the same application rate of 125 g of product per ha for this study. The experiment had 3 treatments and each treatment had 6 replications with the plot size of 3,000 sq. meter.
Treatment-1: Untreated control.
Treatment-2: AMF consortium applied as soil drench using conventional sprayer.
Treatment-3: AMF consortium application using drones.
Conventional backpack sprayer was used for application of AMF in Treatment-2, at a spray/soil drench volume of 200 L per ha. For drone application, we used model Sprayer Drone E616S. Field water level at transplanting was kept minimum during AMF application stage. Buffer rows (6 planting rows) were maintained between the plots on all the four sides to prevent drifting effect due to drone application.
The fertilizer application of nitrogen, phosphorous and potassium was 275, 66 and 215 kg per ha, respectively. Fifty percent of total amount fertilizer was applied as basal fertilizer during the land preparation; the remaining amount of 50% fertilizer was applied in four split applications, at 10, 20, 65 and 90 DAT. Insect pests, diseases and weeds were closely monitored and controlled timely using approved chemicals. Flag leaf width (cm) and flag leaf length (cm) were measured on the main stem of 15 uniform plants in each replication. Flag leaf area (cm2) was measured one week before harvest and calculated following the formula adapted by Yoshida et al. (1976) as follows:
Flag leaf area (cm2) = Leaf length (cm) × Maximum width (cm)
The experimental plots were harvested leaving the border rows to avoid border effect. Before harvesting the whole plot, the number of total tillers and productive tillers per plant had been determined in 6 sub plots (consist of one square meter) of each plot, and tillers of 15 plants per plot were separated into categories according to the marked hang tag, and examined for panicle length, 1,000-grain weight, seed setting percentage, and numbers of filled and unfilled grains per panicle separately. Subsequently, the entire crop was harvested from the net plot area and the grain was hand threshed, winnowed and sun dried to bring the moisture content to 14 per cent and the yield was recorded net plot wise and computed to kg ha-1. Assessment on grain weight and milling as per Rice Knowledge Bank (IRRI, 2010) were carried at the Laboratory of Food and Security, Universiti Putra Malaysia, Selangor. Analysis of variance were calculated to determine the effect of treatments on the response to variables and treatment means were compared by Tukey test, using Minitab Version 18 test.
RESULTS
Rice tillers are specialized branches bearing panicles and provide nutrients for panicle development (Hanada, 1993; Wang & Li, 2011). Number of tillers and the quality of their growth directly determine the number and development of panicles, and ultimately affect the yield of rice. In our experiment, AMF application by drones and conventional sprayer application, significantly increased the total number of tillers and the number of productive tillers (Table 1). However, there is no significant difference in the number of unproductive tillers among treatments. Unproductive tillers do not produce panicles and often die, and hence are considered at best as useless, or as competitive for resources such as solar energy, assimilates, and nutrients that are important for the productive tillers.
Flag leaf characteristics of different treatments are presented in Table 2. We could not find any significant difference in flag leaf length, width, and the flag leaf area among all the treatments.
Spikelet number per panicle (SNPP) is one of the most important yield components used to estimate rice yields. The typical rice spikelet contains a single fertile floret and produces only one grain. There is no significant difference in number of spikelets among different treatments (Table 3). Similarly, there is no significant change in filled grains and percentage of filled grains, although numerically higher percentage was recorded in AMF treated plots (74.63% to 76.69%) as against only 67.69% in untreated plants.
Thousand grain weight (TGW) is an important factor that influences the yield of rice. Increasing TGW can lead to higher rice yields, which is crucial for overall grain yield. Several studies have shown that TGW is positively correlated with grain yield per hectare (Gayathri & Premalatha, 2023). In this experiment, we could not see any significant difference in TGW among treatments, although there is a numerical increase in treatment received AMF application (Table 4). However, significant grain yield increase was recorded in AMF treated plots. Interestingly, highest grain yield increase was recorded in plot received AMF application by drones. Grain yield increase of 10.89% and 12.98 % was recorded in plot received AMF inoculation by knapsack sprayer and drone application, respectively.
Freshly harvested rice grains, known as “paddy” or “rough” rice, are usually milled before consumption. Paddy typically comprises about 20% husk, 11% bran and 69% starchy endosperm. Milling involves de-hulling the paddy and segregating them as whole (head) rice and broken rice. Several factors potentially affect milling and the yield of head rice. Head rice is used for human consumption, while broken kernels are used for animal feed, rice flour, and the brewing industry (Esa et al., 2013). In this experiment, AMF application seem to have positively impacted milling which resulted in significantly higher head rice percentage. Lowest percentage of head rice (66.93%) was recorded in UTC and highest percentage of 73.18% was recorded in AMF applied using backpack sprayer. Interestingly, there is significant difference in head rice percentage between drone application and conventional backpack application.
DISCUSSION
Tillering in rice is an important agronomic trait for grain production and a key component of yield for major cereals such as wheat, rice, barley and rye (Sakamoto and Matsuoka, 2004; Sreenivasulu and Schnurbusch, 2012). Productive tillers are derived from tillers that have more than two green leaves or are longer than two-thirds of the plant length at the maximum tillering stage (Matsushima, 1959). Unproductive tillers do not produce panicles and often die, and hence are considered at best as useless, or as competitor for resources such as solar energy, assimilates, and nutrients that are important for the productive tillers; thus, fewer unproductive tillers are desired in rice cultivation and breeding in order to achieve a high yield (Khush, 1995; Ohe and Mimoto, 2002). In the present study, AMF treated plants produced significantly higher number of productive tillers, which is similar results recorded earlier by Nadjilom et al., (2020) and Iffah & Christopher (2021).
Microbial inoculants are an essential building block for resilient and sustainable food production systems and have the potential to increase farm productivity in a sustainable way. Their use reinforces various global challenges and sustainable development goals, such as food safety, food security, and climate change mitigation (D’Hondt et al., 2021). There are not too many studies on AMF symbiosis on rice mainly because rice is mostly grown in waterlogged anaerobic conditions, which usually do not favor AMF colonization. Paddy is reported to have derived from a semi-aquatic ancestor with unique characteristics, and it can regulate AMF colonization under waterlogged conditions by influencing root architecture, anatomy, and physiology, even though AMF are obligate aerobes. In such conditions, rice plants develop aerenchymatous (also known as aeriferous parenchyma) spongy tissues that enable AMF to exchange gases in the atmosphere (Lumini et al., 2011; Kalamulla et al., 2022). Nevertheless, the symbiosis of AMF and rice plants has been reported over a period of time (Secilia and Bagyaraj, 1994; Wangiyana et al., 2006; Lumini et al., 2011; Watanarojanaporn et al., 2013; Vallino et al., 2014). For instance, the system of rice intensification promotes root colonization and diversity of AMF species in rice roots compared to conventional rain-fed rice cultivation systems (Watanarojanaporn et al., 2013). AMF are naturally more abundant in low-input farming and under aerobic conditions than under partly anaerobic and submerged conditions (Lumini et al., 2011; Vallino et al., 2014). While studying on transplanted paddy, Solaiman & Hirata (1997) conclude that the AMF inoculation at the nursery stage under both dry and wet conditions increased growth, grain yield and nutrient acquisition of wetland rice under field conditions. In the present study, inoculated AMF paddy plants produced higher grain yield which was 11-13% higher than those of not inoculated plants. These findings are like previous studies by Zhang et al., (2014) and Iffah & Christopher (2021) where AMF had a significant effect on production and productivity of paddy.
The shortage of labor, especially seasonal workers, has become a common problem and pushed up the labor cost in agricultural production. By leveraging drone technology, farmers can optimize their operations, reduce labor-intensive tasks, and achieve cost-savings while reducing the overall rice production cost and productivity. Results of the present study precisely confirm that AMF could be applied with drones, and we can lower the cost of application.
USE OF DRONES IN RICE CULTIVATION IN MALAYSIA
The use of drones in rice cultivation in Malaysia began gaining traction in the mid-2010s, driven by efforts to modernize agriculture and address challenges like labor shortages and the need for precision. Initially introduced through pilot projects by the Malaysian Agricultural Research and Development Institute (MARDI) and tech companies, drones quickly showcased their ability to efficiently apply fertilizers and pesticides while monitoring crop health. By 2018, drone adoption expanded under government-backed smart farming initiatives, particularly in rice-producing regions like the Muda Agricultural Development Authority (MADA). By 2021, drones had become mainstream, with their use in rice farming further encouraged by government subsidies, private partnerships, and improved technology.
In MADA, popular drone models include the DJI Agras T20 Pro, DJI Agras T30, and DJI Agras MG-1P. The T20 Pro, priced between RM30,000 (US$6,723) and RM35,000 (US$7,843), is favored for large-scale operations with its 20-liter tank. The T30, costing RM50,000 (US$11,205) to RM55,000 (US$12,325), offers even greater capacity, while the more affordable MG-1P, priced at RM25,000 (US$5,602) to RM28,000 (US$6,290), remains reliable for smaller operations. These drones are prized for their ability to cover large fields efficiently, reducing labor costs and improving operational speed.
Drones have proven highly effective in rice farming, capable of covering 10–16 hectares per day. The precision of drone spraying ensures uniform application, reducing pesticide and fertilizer use by 15–30%. Additionally, drones can spray a hectare in 15-20 minutes, whereas manual spraying takes several hours. This efficiency reduces reliance on labor and helps mitigate labor shortages. Drones also offer improvements in yield, with some reports suggesting a 5-10% increase due to better pest and disease management.
Overall, drones have become an essential tool in modernizing rice farming in Malaysia, offering increased efficiency, reduced costs, and improved sustainability in the sector.
The economic comparison of using drones versus manual spraying for rice cultivation in the Muda Agricultural Development Authority (MADA) region highlights significant cost savings and increased profits. When comparing total costs per hectare, manual spraying incurs RM1,900 (US$426), while drone spraying costs RM1,550(US$366), resulting in a savings of RM350(US$78) per hectare. Revenue from rice production, assuming an average yield of 4 metric tons per hectare at a market price of RM1,500 (US$336) per metric ton, is estimated at RM6,000(US$1,345) per hectare.
The use of drones offers economic benefits by saving on labor costs, reducing spraying time, and improving resource efficiency. Over the course of a farming season, the savings per hectare can add up significantly, especially for larger farms. With precise and efficient operations, drones contribute to more consistent yields, making them a profitable long-term investment in rice farming.
CONCLUSION
Use of bio-fertilizer or microbial biostimulant such as AMF is one of the important productivity enhancement supplements which could be used in a sustainable way to increase rice grains and head rice yields. Results of this study also clearly indicate that sprayable AMF formulation could be applied using drones and it is as good as manual soil drenching and hence could be a viable alternative method for application of arbuscular mycorrhizal fungi in paddy. To our knowledge, this is the first ever study reporting on the use of drones for application of AMF inoculants in paddy.
REFERENCES
Baez-Rogelio, A.; Morales-García, Y.E.; Quintero-Hernández, V.; Muñoz-Rojas, J. Next generation of microbial inoculants for agriculture and bioremediation. 2017. Microb. Biotechnol, 10, 19–21.
Bello-Schünemann, J., Cilliers, J., Donnenfeld, Z., Aucoin, C., and Porter, A. 2017. African Futures—Key Trends to 2035. Pretoria: Institute for Security Studies. Policy brief. doi: 10.2139/ssrn.3099362.
Berruti, A., Lumini, E., Balestrini, R and Bianciotto, V. 2016. Arbuscular Mycorrhizal Fungi as Natural Biofertilizers: Let's Benefit from Past Successes. Frontiers in Microbiology. 6:1559. doi: 10.3389/fmicb.2015.01559.
Bernaola L., Cange G., Way M. O., Gore J., Hardke J., Stout M. 2018. Natural colonization of rice by arbuscular mycorrhizal fungi in different production areas. Rice Sci. 25 169–174. 10.1016/j.rsci.2018.02.006.
Carvalho F.P. 2017. Pesticides, environment, and food safety. Food Energy Secur. 6:48–60. doi: 10.1002/fes3.108.
Chen, S., Zhao, H., Zou, C., Li, Y., Chen, Y., Wang, Z. & Ahammed, G. J. 2017. Combined inoculation with multiple arbuscular mycorrhizal fungi improves growth, nutrient uptake and photosynthesis in cucumber seedling. Front Microbiol., 8 (2516), 1-11. https://doi.org/10.3389/fmicb.2017.02516
Corrêa, A., Cruz, C., Pérez-Tienda, J., and Ferrol, N. 2014. Shedding light onto nutrient responses of arbuscular mycorrhizal plants: nutrient interactions may lead to unpredicted outcomes of the symbiosis. Plant Sci. 221, 29–41. doi:10.1016/j.plantsci.2014.01.009
D’Hondt, K., Kostic, T., McDowell, R., Eudes, F., Singh, B.K., Sarkar, S., Markakis, M., Schelkle, B., Maguin, E., and Sessitsch, A. 2021. Microbiome innovations for a sustainable future. Nat. Microbiol. 6, 138–142. https://doi.org/10.1038/.
Dorairaj, D.; Govender, N.T. 2023. Rice and paddy industry in Malaysia: Governance and policies, research trends, technology adoption and resilience. Front. Sustain. Food Syst. 7, 1093605.
Esa, N.M.; Ling, T.B.; Peng, L.S. By-products of Rice Processing. 2013. An Overview of Health Benefits and Applications. Rice Res. Open Access, 1, 1–11.
FAO. FAOSTAT. 2019. Production Data www.fao.org/faostat/en/#data.
FAOSTAT. 2021. Production Data www.fao.org/faostat/en/#data.
FAO. 2022. The State of Food Security and Nutrition in the World 2022. https://www.fao.org/3/cc0639en/cc0639en.
Firdaus, R. B. R., Tan, M. L., Rahmat, S. R., & Gunaratne, S. M. 2020. Paddy, rice and food security in Malaysia: A review of climate change impacts. Cogent Social Sciences, 6, 1-17. https://doi.org/10.1080/23311886.2020.1818373
Gayahtri, N. K. & Premalatha, Y, 2023. Studies on Correlation and Path Analysis in Medium Duration Rice Varieties of Andhra Pradesh, India. Emerging Issues in Agricultural Sciences Vol. 2, 25: 77-88.
Gewaily E. E. (2019). Influence of arbuscular mycorrhizal fungi (AMF) inoculation on the performance of Sakha 107 rice cultivar under different irrigation intervals. Env. Biodiv. Soil Security 3 119–129. 10.21608/jenvbs.2019.15637.1068.
Grassini, P., Eskridge, K. M. & Cassman, K. G. Distinguishing between yield advances and yield plateaus in historical crop production trends. Nat. Commun. 4, 2918 (2013).
Hanada, K. 1993. Tillers. 1. Plant growth and tillering. In: Matsuo T, Hoshikawa K. eds. Science of the rice plant, vol. 1. Tokyo: Food and Agriculture Policy Research Center, 222–229
Iffah, H & Christopher, M, (2021) Effect of in vitro Produced Arbuscular Mycorrhizal Fungi Inoculum on Anaerobic Direct Seeded and Transplanted Paddy. Journal of Agricultural Science; Vol. 13, No. 6; 18-30.
Ilag, L. L., Rosales, A. M., Elazegui, F. A., & Mew, T. W. (1987). Changes in the population of infective endomycorrhizal fungi in a rice-based cropping system. Plant and Soil, 103, 67-73. https://doi.org/10.1007/BF02370669
IRRI. (2010). Rice Knowledge Bank. Retrieved from https://www.knowledgebank.irri.org.
Kalamulla, R., Sandaruwan, D., Karunarathna, S. C., Stephenson, S. L., Tibpromma, S., Elgorban, A. M., Salim Al-Rejaie, Yapa, P. N. and Suwannarach, N. (2022) Assessment of Community Dynamics of Arbuscular Mycorrhizal Fungi in the Rice (Oryza sativa L.) Rhizosphere and Potential Application as Biofertilizer. Sustainability 14, 16537. https://doi.org/10.3390/su142416537.
Khazanah Research Institute, 2019. The Status of the Paddy and Rice Industry in Malaysia. pp 220.
Khush GS. 1995. Breaking the yield frontier of rice. GeoJournal 35, 329–332.
Y. Liu, X. Pan, J. Li A 1961–2010 record of fertilizer use, pesticide application and cereal yields: a review. Agron. Sustain. Dev., 35 (2015), pp. 83-93, 10.1007/s13593-014-0259-9.
Lobell, D. B. & Gourdji, S. M. 2011. The Influence of Climate Change on Global Crop Productivity. Plant Physiology, Vol. 160, pp. 1686–1697.
Lumini, E., Vallino, M., Alguacil, M. M., Romani, M., & Bianciotto, V. (2011). Different farming and water regimes in Italian rice fields affect arbuscular mycorrhizal fungal soil communities. Ecol Appl, 21(5), 1696-1707. https://doi.org/10.1890/10-1542.1
Maclean, J., Hardy, B. and Hettel, G. 2013. Rice Almanac: Source book for one of the most important economic activities on earth. IRRI.
Matsushima S. 1959. Theories and techniques in rice cultivation – theory on yield analysis and its application. Tokyo: Yokendo. [In Japanese.]
Nadjilom, Y., Takoukam, S., Toukam, Issa, M. & Ngakou. A. 2020. Field Evaluation of Growth and Yield of Two Local Rice Varieties (Tox-728-1 and Madjitolngar) in Response to Indogenous Mycorrhizal Inoculation in South-Chad. American Journal of Plant Sciences, 11, 1175-1192. www.scirp.org/journal/ajps.
Ohe M, Mimoto H. (2002) Appropriate time of deep-water treatment for regulation of rice growth. Japanese Journal of Crop Science 71, 335–342. [In Japanese.]
Pretty, J., Benton, T. G., Bharucha, Z. P., Dicks, L. V., Butler Flora, C., Godfray, H. C. J., et al. (2018). Global assessment of agricultural system redesign for sustainable intensication. Nat Sustain 1, 441–446. doi: 10.1038/s41893-018-0114-0.
Ramankutty, N., Evan, A. T., Monfreda, C., and Foley, J. A. (2008). Farming the planet: 1. Geographic distribution of global agricultural lands in the year 2000. Glob Biogeochem. Cycles 22. doi: 10.1029/2007gb002952.
Rouphael, Y. & Colla, G. (2020) Biostimulants in agriculture – Editorial. Frontiers in Plant Science. 11 (40) 1-7.
Royal Society (2009) Geoengineering the climate: science, governance, and uncertainty. London: Royal Society. http://royal society.org/policy/Publications/2009/geoengineering climate.
Sakamoto, T. & Matsuoka, M. (2004) Generating high-yielding varieties by genetic manipulation of plant architecture. Curr. Opin. Biotech. 15, 144-147. DOI:10.1016/j.copbio.2004.02.003.
Sánchez, M. R., Baños, Y. S., Hernández, Y. M., Martínez, A. Y., Benitez, M., Bharat, B. V. and Chávez, Y. P. (2015) Arbuscular mycorrhizal symbiosis in rice (Oryza sativa L.) plants in flooded and non-flooded conditions. Acta Agronómica. 64 (3), p 211-217. doi: http:// dx.doi.org/10.15446/acag.v64n3.43087.
Secilia J., Bagyaraj D. J. (1994). Selection of efficient vesicular-arbuscular mycorrhizal fungi for wetland rice-a preliminary screen. Mycorrhiza 4 265–268. 10.1007/s005720050031.
Solaiman, M., Hirata, H. Effect of arbuscular mycorrhizal fungi inoculation of rice seedlings at the nursery stage upon performance in the paddy field and greenhouse. Plant and Soil 191, 1–12 (1997). https://doi.org/10.1023/A:1004238028617.
Sreenivasulu, N., Schnurbusch, T.. 2012. A genetic playground for enhancing grain number in cereals. Trends Plant Sci. 17(2): 91–101. doi: 10.1016/j.tplants.2011.11.003 http://gateway.webofknowledge.com/gateway/Gateway.cgi?GWVersion=2&SrcApp=PARTNER_APP&SrcAut.
Steffen, W., Richardson, K., Rockström, J., Cornell, S.E., Fetzer, I., Bennett, E.M., Biggs, R., Carpenter, S.R., de Vries, W., de Wit, C.A., et al. Sustainability, Planetary boundaries: guiding human development on a changing planet. Science, 347 (2015), p. 1259855, 10.1126/science.1259855.
United Nations World population prospects 2019: Highlights Dep. Econ. Soc. Aff. (2019).
United Nations. 2022. World population prospects 2022: summary of results. New York: United Nations, Department of Economic and Social Affairs, Population Division.
Vallino, M.; Greppi, D.; Novero, M.; Bonfante, P.; Lupotto, E. Rice root colonisation by mycorrhizal and endophytic fungi in aerobic soil. Ann. Appl. Biol. 2009, 154, 195–204.
Vallino, M.; Fiorilli, V.; Bonfante, P. Rice flooding negatively impacts root branching and arbuscular mycorrhizal colonization, but not fungal viability. Plant Cell Environ. 2014, 37, 557–572.
Vermeulen, S.J., Campbell, B.M. & Ingram, J.S.I. (2012) Climate change and food systems. Annu. Rev. Environ. Resour., 37, pp. 195-222, 10.1146/annurev-environ-020411-130608.
Wang P., Jiang H., Boeren S., Dings H., Kulikova O., Bisseling T., et al. (2021). A nuclear-targeted effector of Rhizophagus irregularis interferes with Histone 2B mono-ubiquitination to promote arbuscular mycorrhization. New Phytol. 230 1142–1155. 10.1111/nph.17236.
Wang, Y., & Li, J. (2011). Branching in rice. Current Opinion in Plant Biology, 14(1), 94–99. https://doi.org/10.1016/j.pbi.2010.11.002.
Wang Y., Li T., Li Y., Björn L. O., Rosendahl S., Olsson P. A., et al. (2015). Community dynamics of arbuscular mycorrhizal fungi in high-input and intensively irrigated rice cultivation systems. Appl. Environ. Microb. 81 2958–2965. 10.1128/aem.03769-14.
Wangiyana W, Cornish P.S, Morris E.C. Arbuscular mycorrhizal fungi dynamics in contrasting cropping systems on vertisol and regosol soils of Lombok, Indonesia. Exp Agric. 2006;42(4):427–439. doi: 10.1017/S0014479706003826.
Watanarojanaporn, N.; Boonkerd, N.; Tittabutr, P.; Longtonglang, A.; Young, J.P.W.; Teaumroong, N. Effect of rice cultivation systems on indigenous arbuscular mycorrhizal fungal community structure. Microbes Environ. 2013, 28, 316–324.
Woods, J., A. Williams, J.K. Hughes, M. Black, R. Murphy Energy and the food system. Philos. Trans. R. Soc. Lond., 365 (2010), pp. 2991-3006, 10.1098/rstb.2010.0172.
Zhang, S.; Wang, L.; Ma, F.; Bloomfield, K.J.; Yang, J.; Atkin, O.K. Is Resource Allocation and Grain Yield of Rice Altered by Inoculation with Arbuscular Mycorrhizal Fungi? J. Plant Ecol. 2014, 8, 436–448.
Zhang S., Wang L., Ma F., Bloomfield K. J., Yang J., Atkin O. K. (2015). Is resource allocation and grain yield of rice altered by inoculation with arbuscular mycorrhizal fungi? J. Plant Ecol. 8 436–448. 10.1093/jpe/rtu025.