DOI: https://doi.org/10.56669/KSHW3457
ABSTRACT
Keeping a tab on the agricultural production systems on a maintained level is a challenge for the future as the Indian population is increasing at a rapid pace. Plant diseases cause considerable losses in crop production and storage. Chemical pesticides are commonly used to control pests, which cause harmful impacts on the environment and non-target living systems, including human beings. Biopesticides are natural and a better substitute for chemical pesticides and provide an alternative for crop protection worldwide. The trend of biopesticides consumption in India has shown a drastic increase in use over time which stood at 8,647 and 8,898 metric tons in 2020-21 and 2021-22, respectively. Although the value of eco-friendly pest management in sustainable agriculture has been well recognized, only very little is being adapted at the field level. But making it reach to the field with a suitable delivery method maintaining consistent performance is the next most important challenge. The bioagents cannot be applied as spore suspension in field but are applied as powdered or liquid formulation primarily through seed treatment, soil application, root dip or foliar application. In India, as compared to chemical pesticides, biopesticides production, utilization, and consumption are much lower due to a lack of research advancements, innovation and policies. To increase the use of biopesticides at the country level, it is necessary to understand their mode of action, contribution to sustainable agriculture and their effects on human health and plant diseases. Thus, in this article we have discussed about overview of biopesticides, their classification, formulations, application, commercialization, current status and prospects.
Keywords: Application and Commercialization, Biopesticides, Formulations, India
INTRODUCTION
Agriculture is an anthropogenic activity but adversely affected by various pests such as thousands of insects, fungi, viruses, bacteria, nematodes, weeds and other living forms, leading to crop yield and production quality reduction (Kumar, 2012). With the advent of agriculture, plant diseases became a problem. Over the past 50 years, the most common method for pest control has been the extensive use of chemical pesticides (Peshin et al., 2009; Peshin and Zhang, 2014; Zhang et al., 2011; Zhang, 2018). However, the high effectiveness and ease of utilization of these chemicals can result in environmental contamination and the presence of pesticide residues in food, in addition to social and economic problems. By using agrochemicals, agriculture productivity has increased rapidly. In India, 381 g/ha of chemical and synthetic pesticides is being consumed annually and the rate of consumption rising from 2 to 5% yearly. However, the pesticides consumption is relatively lower in India than the worldwide consumption of 500 g/ha (Vendan, 2016). Chemical and synthetic pesticides are used in excessive amounts to control pests in crop fields and it also deteriorates the soil fertility and ecosystem (Zhang et al., 2011; Zhang, 2018). But it is the most effective tool for integrated pest management (IPM) (Kumari et al., 2014). However, they also have adverse impacts on water quality, soil health, product quality, and developed problems such as insecticide resistance, genetic variation in plants, toxic residues, and food safety. The usage of pesticides may damage the indigenous microorganisms, disturb the soil ecosystem, also reduce the soil enzymatic activities that act as a "biological index" of soil fertility, and may also affect human health via the food chain (Antonious, 2003; Ingram et al., 2005; Littlefield-Wyeret al., 2008; Monkiedje and Spiteller, 2002; Wang et al., 2006). It has become necessary to develop substitutes for these synthetic agro-inputs due to the evolution of pesticide resistance in some pest species and concerns about the safety of chemical residues. Currently the need is to produce maximum from the diminishing natural resources and protect the produce from post-harvest losses without adversely affecting the environment (Yadav et al., 2022). In this context, biological control through the use of natural antagonistic microorganisms has emerged as a promising alternative.
Biopesticide is a mass-produced, biologically based agent manufactured from a living microorganism or a natural product and which is sold for the control of plant pests (Organisation for Economic Co-operation and Development (OECD), 2009). Biopesticides are made from the substances that control pests by non-toxic mechanisms and have been used in different forms since human civilization. Biopesticides have more potential benefits for agriculture as well as public health programs. Biopesticides consist of various microbial pesticides, biochemicals generated from microbes and other natural sources. These are usually made by growing and concentrating naturally existing organisms and their metabolites, such as bacteria and other microorganisms, fungus, nematodes, etc. These are frequently considered vital components of IPM programs and have gained a lot of practical attention as alternatives to chemical and synthetic pesticides (Glare et al., 2012). Biopesticides could be derived from animals (e.g., nematodes), plants such as Chrysanthemum, Azadirachta (Neem), and microorganisms (e.g., Bacillus thuringiensis, Trichoderma, Pseudomonas), and include living organisms (natural enemies), their product (phytochemicals, microbial products) which can be used for the management of pest injurious (Mazidet al., 2011). The potential benefits of the utilization of biopesticides in agriculture and public health programs are considerable. The present paper provides an overview of biopesticides and their classification, formulations, application, current status and prospects.
BIOPESTICIDES
Biopesticides are pesticides derived from microorganisms or natural products that control pests through different mechanisms of action (Tijjani et al., 2016). Plants, insects and microorganisms are the primary sources of biopesticides which are cheaper, readily available, demonstrate various modes of action and are degradable. They are products or by-products derived from microorganisms (Bacillus thuringiensis, Verticillium lecanii), insects (Trichogramma spp.), animals (nematode), plant parts or extracts (Azadirachta indica) (Pavela, 2014). In the past few decades, biopesticides are the best substitute against chemical and synthetic pesticides in managing pests. They are currently used in the post- and pre-harvest control of diseases and crop pests (Yadav, 2017; Kour et al., 2020). Biopesticides are target-specific and are nontoxic to the environment and humans. The mode of action of biopesticides is specific and operates by targeting pests. Nowadays, biopesticides have played a vital role in the agro-market and are widely utilised in organic farming (Seiber et al., 2014; Nawaz et al., 2016; Lengai and Muthomi, 2018).
Biopesticides play a vital role in crop protection. They are compatible with other chemical pesticides and are also utilized in integrated crop management (ICM) practices throughout the world. Due to advancements in research and development, biopesticides have raised sustainability and reduced the pollution caused by chemical pesticides. Production of biopesticides is challenging due to the dissimilarity of the active and integrated ingredients. Moreover, when utilized as a component of IPM programs, biopesticides achieve an equivalent level of crop yield by reducing the load of chemical pesticides (Aneja et al., 2016; Satapathy, 2018; Zhang and Liu, 2022).
Biopesticides are easily available in nature, easily biodegradable, show different modes of actions, are less expensive and possess less toxicity to live organisms. Therefore, it was realized that biological control is the only means of a safe, cost-effective, and eco-friendly method to control the widespread resistance of chemical insecticides towards pests. Although the value of eco-friendly pest management in sustainable agriculture has been well recognized, only very little is being adapted at the field level. (Mishra et al., 2020). Globally, research on the application and stability of diverse biopesticides can help to assist sustainable agriculture (Kumar et al., 2019; Yadav and Yadav, 2019).
They are categorized into (a) microbial biopesticides containing microorganisms controlling diseases and insects, (b) biochemical pesticides (plant-derived), (c) plant-incorporated protectants (Kumar et al., 2019), and (d) beneficial insects (Osman et al., 2015) (Fig. 1).
Microbial pesticides
Microbial pesticides are products derived from various microorganisms (e.g. bacterium, fungus, virus, nematodes or protozoan) that are used as an active ingredient to control pests. Microbial products may consist of the organisms themselves and/or the metabolites they produce. They can control many different kinds of pests, although each separate active ingredient is relatively specific for its target pest.
Biochemical pesticides
Biochemical pesticides are naturally occurring compounds derived from various insect growth regulators, plant extracts, pheromones, oils and pyrethrins. They are characterized by a non-toxic mode of action that may affect the growth and development of a pest and its ability to reproduce. Biochemical pesticides are of following types:
Plant-Incorporated Protectants (PIPs)
Plant-Incorporated Protectants are the genes and proteins, which are introduced into plants by genetic engineering. They allow the genetically modified plant to protect itself from pests, like certain insects or viruses. These self-made pesticides are called "plant-incorporated protectants" (PIPs). PIP-producing crops are sometimes called "genetically modified" (GM) or "genetically engineered" (GE). For example, some plants produce insect-killing proteins within their tissues. They can do this because genes from Bacillus thuringiensis were inserted into the plant’s DNA.
Beneficial insects
Human cultures and civilizations have been maintained in countless ways through these beneficial insects, they regulate the pest population of many harmful pest species, produce natural products, and they also dispose the waste and recycle the organic nutrients. The generalized intensification of agriculture and the use of broad-spectrum pesticides decrease the diversity of natural enemy populations and increase the likelihood of pest outbreaks. Indeed, pesticide use has been shown to be associated with a large decrease in natural pest control services. Thus, enhancement of agro-ecosystem appears to be one of the best ways in which we can decrease the use of chemical pesticides for pest and disease control and it will increase the sustainability of crop production. These beneficial insects act by preying on pest insects or by parasitizing pest insects (parasitoids).
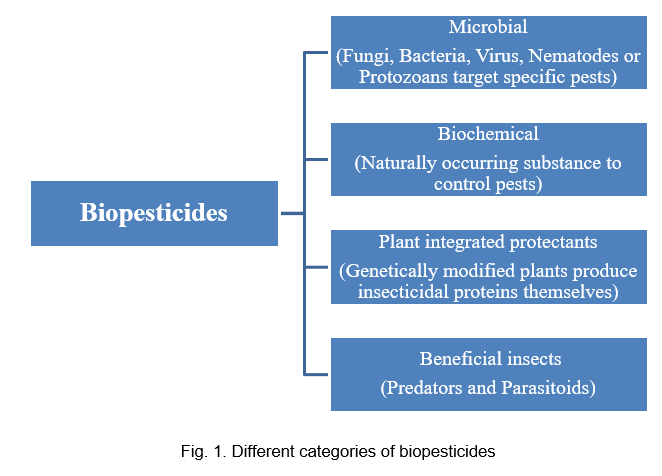
STATUS OF BIOPESTICIDES IN INDIA
The biocontrol concept of plant diseases has been started in India for an extended time (Schmutterer, 1985). The neem tree (Azadirachta indica A. Juss) and its derivatives, such as leaf extract, oil, and seed cake, have been used as fertilizers to minimise the risk of post-harvest loss in stored storage cereals (Brahmachari, 2004; Isman, 1997). During the 1960s, with a target of judicious use of pesticides in agriculture, the concept of IPM had also arisen (Smith and van den Bosch, 1967). However, in India, a major technological breakthrough in biocontrol occurred when conventional insecticides failed to control Helicoverpa armigera, Spodoptera litura and other cotton pests (Kranthi et al., 2002).
Around the world total biopesticides’ production is 3,000 tons per year and their use is enhancing steadily by 10% every year (Gupta and Dixit, 2010; Kumar and Singh, 2015). Marrone (2007) states that about 1,400 biopesticide products are prepared and sold worldwide. Over 200 biopesticides are sold in the US market as compared to only 60 similar products available in the European Union (EU) (EPA, 2012). In comparison, Asia lacks biopesticides and uses only 5% of biopesticides sold globally (Bailey et al., 2010; Hubbard et al., 2014).
Biopesticides are registered and regulated under the Insecticides Act, 1968. The India biopesticides market size was US$64.73 million in 2021. The market is expected to grow from US$69.62 million in 2022 to US$130.37 million by 2029, exhibiting a CAGR of 9.38% during the forecast period. Fortune Business Insights™ presents this information in its report titled “India Biopesticides Market, 2022-2029”. For sustainable farming, National Farmer Policy 2007 has promoted the utilization of biopesticides (Dar et al., 2019). In India, biopesticides have been registered under the Insecticide Act, 1968 India, which shows different biopesticides formulated in various industries (Table 1; Fig. 2). The trend of biopesticides consumption in India has shown a drastic increase in uses over time which stood at 8,647 and 8,898 tons in 2020-21 and 2021-22, respectively (Fig. 3).
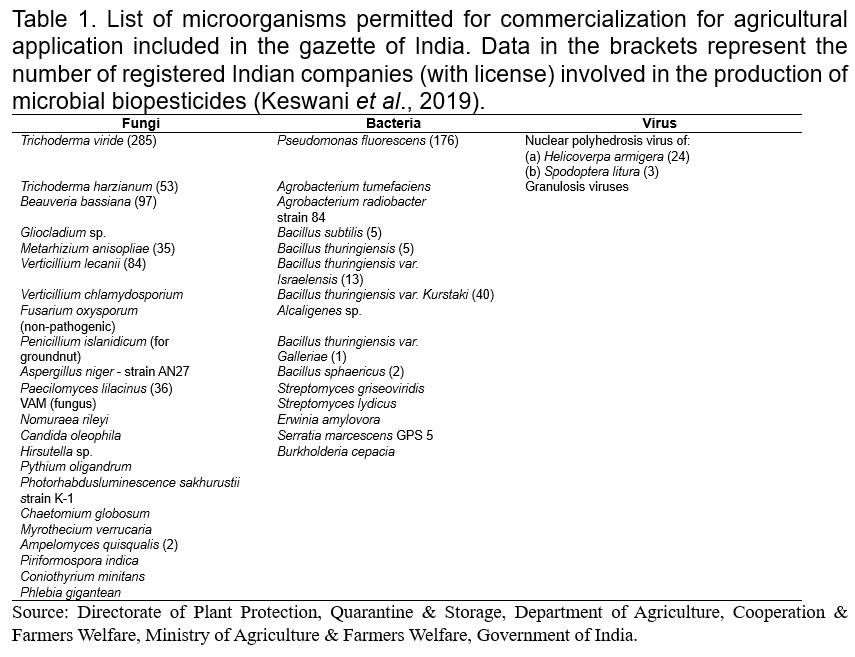
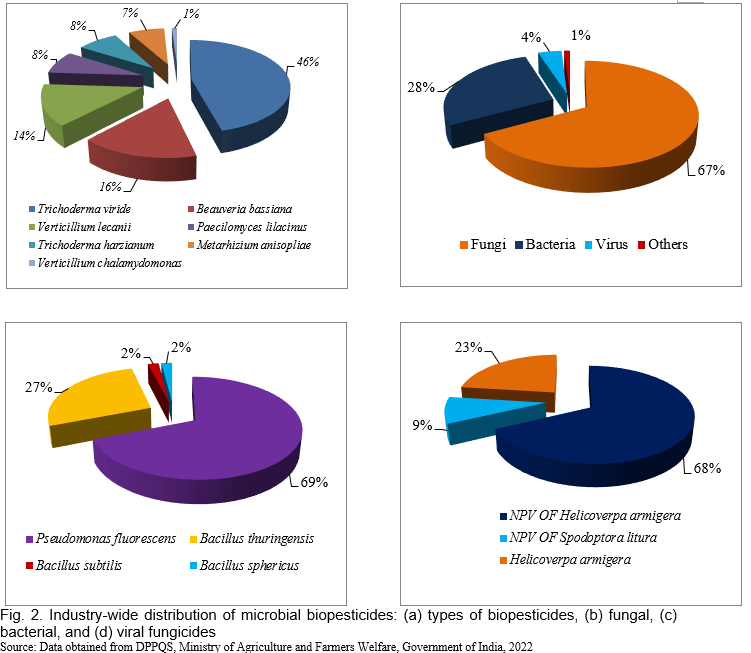
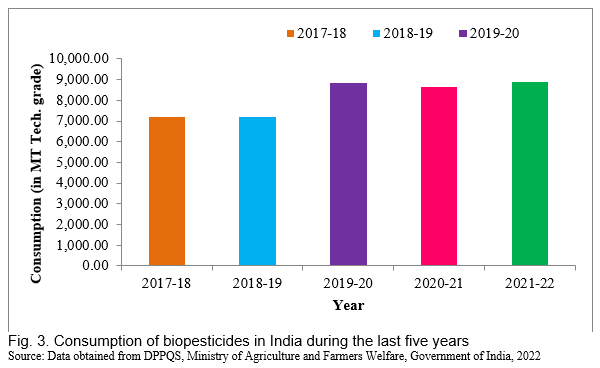
In recent years, there has been a tremendous increase in the use of biopesticides, and the area of cultivation of biopesticides has been rapidly increasing. In India, West Bengal, Rajasthan, and Maharashtra used the maximum amount of biopesticides in 2021-22. Figs. 4, 5 and 6 represent the consumption and area under the use of chemical pesticides and bio-pesticides in various crops of India during 2017-18 to 2021-22. The rapid growth in the biopesticide market is based on the advantages such as inherently less harmful, reduced environmental load, affecting only one specific pest or a few pests in some cases, degradable therefore decrease exposure to the biota, thus avoiding the pollution problems, also effective in small quantities, and nontoxic to humans.
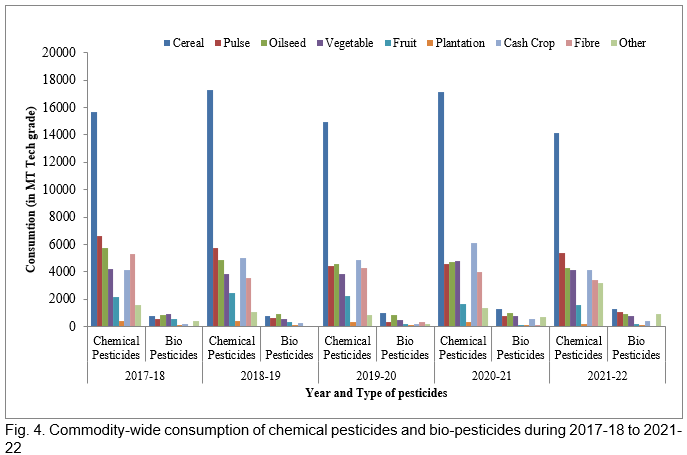
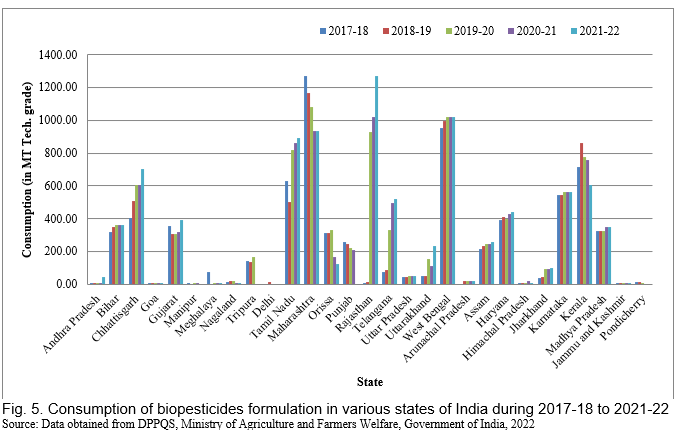
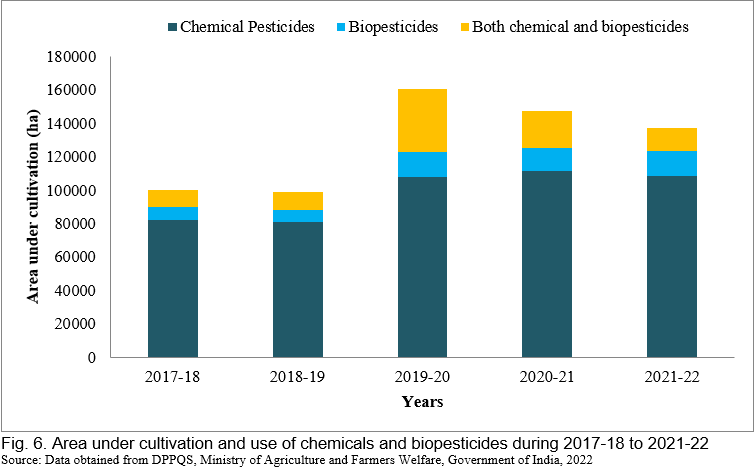
FORMULATIONS OF BIOPESTICIDES
Biopesticides are mainly plants or living organisms’ best extracts. So, during the formulation and storage process, these are to be maintained at the level of acceptance. In the process of biopesticide formulation, adjuvants and different carriers are mixed with phytochemicals to improve the storage stability, protect from the environmental conditions, and improve bioactivity. The major factors to be considered in the formulation process are: 1. stabilization and storage, 2. protection against adverse environmental conditions, 3. easy application, 4. improvement of bioactivity, and 5. maximum interaction with target pest.
Development of a bioformulation is necessary to commercialize biocontrol technologies by industries. The commercial use of bioagents requires inoculum that retains high cell viability and can easily be transported and applied to seed. It needs extensive studies for large-scale multiplication of a biocontrol agent, which include suitable and inexpensive medium, method of fermentation (solid or liquid), type of formulation (wettable powder, liquid, granular), nature of filler material, delivery systems, optimum shelf life, and storage conditions. Application guidelines are set by considering all these aspects of a bioformulation. Delivery of free cell form is usually impractical to achieve satisfactory bioremediative effect because microbes are encumbered by biotic and abiotic stresses from the environment (Ting et al., 2010). The aims of formulating viable cells are to ensure that adequate cell viability is sustained to increase the efficacy of the cells and to facilitate the delivery and handling processes (Filho et al., 2001). A bioformulation can improve product stability and shelf life and also protect microbial inoculants against different environmental conditions and provide initial food source (Jambhulkar and Sharma, 2014). Application of microbial inoculants either to increase crop health or to manage plant diseases depends on the development of commercial formulations with suitable carriers that support the survival of microorganism for considerable length of time (Aeron et al., 2011).
The formulations are broadly classified into solids and liquids; however, there are several subcategories of bioformulation that are presently accessible and utilized across India.
Solid formulation
The most prevalent type of solid bio formulations comprises of granules, micro granules, wettable powders (WPs), wettable granules (WGs), water-dispersible granules (WDGs), and dust. They are developed by mixing a binder, a dispersant, a wetting agent, etc. (Knowles, 2008). Granular formulations include dry particles with the active ingredient, carrier and binder. The concentration of active gradients in granule (GR) is 5%–20%. Apart from GR-based formulation, WPs have a prolonged shelf life and generally contain 50% – 80% technical powder, 15% – 45% filler, 1% – 10% dispersant and 3%–5% surfactant by weight (Brar et al., 2006). In contrast, dust-type formulated products have a finely ground mixture of the active ingredient (usually 10%) with particle sizes ranging from 50 to 100 µm. The other types of formulated products are the modification of the types and include WG/WDG that make WP more environmentally friendly, non-dusty and quickly soluble in water, but WG and WDG have a higher concentration of the dispersing agent. The WDG has an excellent shelf life and has been widely employed against the control of nematodes.
Liquid formulations
Liquid formulations are aqueous suspensions made in oil, water or oil and water (emulsion) (Schisler et al., 2004). A typical aqueous formulation contains 10% – 40% microorganisms, 35% – 65% carrier liquid (oil or water), 3% – 8% surfactant, 1% – 3% suspender and dispersant 1% – 5% (Brar et al., 2006). The liquid formulations are available in a variety of forms like suspension concentrates (SCs), oil miscible flowable concentrate (OF), ultralow volume (ULV) suspension (SU), and oil dispersion (OD). SCs are made by incorporating solid active ingredients among all these liquid formulations.
Liquid formulations have numerous merits like: (i) high cell count, (ii) prolonged shelf life of over a year due to the occurrence of microorganism in a dormant cyst state, which becomes active in the field after application, (iii) enhanced protection from environmental stresses and improved field efficacy, and (iv) enhancing the activity of the organism at the target site by increasing its contact with the target insect pest or pathogen (Vendan and Thangaraju, 2006).
Novel formulations
The newer formulations are a step up from traditional formulation technologies and these improved formulation types can provide products with a competitive edge, increased shelf life and better field efficacy. These sorts of formulations are often known as new-generation formulations that are environment-friendly in nature (Hazra, 2015). Some of these are illustrated below:
Encapsulation
In formulation technology, encapsulating microorganisms in a polymeric matrix is presently under experimentation. Encapsulation is creating a film or encasing microbial cells in a polymeric material to yield beads that are permeable to minerals, gases, and metabolites while permitting cellular activity to persist inside the beads (Bashan et al., 2014; John et al., 2011). Two approaches are employed depending on the mass of the beads that are produced - macro encapsulation (involving beads that are millimeters to centimeters in size) and microencapsulation (involving beads that are 1–1000 μm in size) (Nordstierna et al., 2010). Bio-encapsulation as a carrier provides a protective environment during field and storage and assists the slow release of microbes. With the usage of encapsulation, particularly suitable for soil applications, microorganisms may be prevented from biotic and abiotic stress factors like contaminants, antagonists or dryness and may proliferate at the site of application due to the beneficial microenvironment provided by the formulation (Vemmer and Patel, 2013).
Among the available polymers, alginate has been the most preferred material for most of the encapsulated formulations. According to Vassilev et al. (2005), there are at least 1,350 combinations of natural, semi-synthetic, and synthetic polymers for the entrapment of biomaterials, but the majority of techniques involved in situ entrapment by using natural polysaccharide gels which include alginates, agar, and carrageenan. The formulation provides a temporary shelter for the encapsulated strain from the soil environment and microbial competitors, both hostile to any change in the biological makeup of the soil. Any premature release of microbes from these encapsulated forms is detrimental because the main purpose of these formulations is to sustain the entrapped cells in an active phase for as long as feasible at high concentrations as nutrients and oxygen are easily accessible to a strain due to simple diffusion, the shelf life increases. The viability of microbes is higher in the case of encapsulation as controlled release to the external environment minimizes the wastage of microbial cells. Minimum storage space is required as it is immobilized in micro or macro beads.
Tablet formulation
Tablets are "preformed solids of uniform shape and dimensions, usually circular, with either flat or convex face, the distance between faces being less than the diameter". A tablet is a solid mass of uniform shape and size of an optimal blend of active ingredients (AIs) and adjuvants (Kala et al., 2020). These tablets offer numerous benefits for the industrial processes in terms of physical-chemical stability and diminished manufacturing costs, as well as beneficial to the consumer concerning easy handling and dosage precision.
The formulation is easy to handle and free from dust due to its compressed form. It offers a premeasured dose rate; thus, there is no need for weighing and making other measurements for the formulation preparation as it can be enumerated by counting the number of tablets. It is suitable in case a low dose is required (Sharma et al., 2005).
Microemulsions
Microemulsions (MEs) are homogeneous and isotropic dispersions with low viscosity, optical transparency, thermodynamic stability and an internal (dispersed) phase having typical sizes of 10-200 nm. Microemulsions are thermodynamically stable transparent dispersions of two immiscible liquids and are stable over a wide temperature range. They have a fine droplet size of fewer than 0.05 microns (50 nanometres). The total concentration of surfactants for a microemulsion can be as high as 10–30% or more, compared with about 5% for a typical o/w emulsion. Microemulsions have relatively low active ingredient concentrations, but the active ingredient's high surfactant content and solubilization may give rise to enhanced biological activity. The oil-in-water formulation was prepared by mixing the surfactant mixed oil phase with the spore suspension in the aqueous phase.
Microemulsions are easy to prepare and scalability with reduced external energy input. The shelf life of the active ingredient is also extended. There is a need to improve the solubilization of poorly water-soluble compounds as well as increase its absorption due to its smaller size.
Oil-water emulsion /oil dispersion
Oils can considerably boost the efficacy of entomopathogens against insects, hyperparasitic fungi and mycoherbicides. Over the last decade, oil dispersions (ODs) have confirmed an increasing relevance. Oil dispersions are suspensions of active ingredients in a water-insoluble solution or oil. In contrast to other liquid formulations, OD is a stable suspension of active ingredient(s) in water-immiscible solvent or oil. For the usage of fungus-containing ODs, specialized defence methods are required.
An experiment was conducted in which oil was used as a carrier to prepare liquid formulations of Trichoderma. Different oil-based formulations of Pseudomonas fluorescens were developed by employing oils like soybean oil, canola oil and groundnut oil to evaluate the viability of bacteria in the formulations (Dobhal et al., 2021). Oil-based formulations of Trichoderma viride using different oils, viz., paraffin oil, soybean oil, and mineral oil were prepared (Akshata et al., 2018). An oil-based formulation of Beauveria bassiana (Bb 112) was prepared and targeted against onion thrips tabaci (Murugasridevi et al., 2021). Several previous studies have emphasized the importance of oil in bio-preparations in contrast to water to enhance the fungal activity for insect pest infection, including the fungus B. bassiana (Prithiva et al., 2017; Sangamithra et al., 2015). The findings that fungal conidia packaged in oils have better infectivity than standard water-based suspensions have enhanced the possibility for mycopesticide development. Oil-based products are preferred for foliar spray and have improved entomopathogen efficacy. Oil evaporation is low; thus, the formulation retains its efficacy for a longer period and can be used as an emulsion. Oil traps water around the organism and slows water evaporation once applied. This is particularly beneficial for microorganisms sensitive to desiccation (Sridevi et al., 2018).
Nanoformulation
Nanotechnology has the potency to improve the delivery of microbial pesticides by utilizing nanosized preparations or formulations based on nanomaterial. Compared to conventional pesticides and associated formulations, nanomaterial-based formulations offer improved efficiency, increased solubility, induction of systemic action owing to smaller particle size and increased mobility, and reduced toxicity due to the removal of organic solvents. Pesticides developed from the nanoformulation technique are called nanopesticides. Nano-pesticides are prepared by very small particles of active pesticide ingredients or other nanostructured molecules with pesticide properties. Researchers have developed different types of nanopesticides like nanocapsule formulations, nanoemulsion, nanogel, nanospheres, and metal and metal oxide nanoparticles (Kah and Hofmann, 2014). They aid in increasing the apparent solubility of a poorly soluble active ingredient or releasing the active ingredient in a slow or targeted manner, thus protecting the active ingredient against premature degradation.
Nanoformulations may provide new strategies to improve the stability of bioagents. The controlled use of biologically active compounds (like agrochemicals) could be possible by developing a smart delivery system using biomaterials (Djiwanti and Kaushik, 2019). The potential of biodegradable and biocompatible nanomaterials like chitosan or clay as enzyme stabilizing and delivery agents is being investigated in a study. Nanoformulations stabilizing the Myrothecium enzyme complex were prepared using chitosan (250–350 nm) and montmorillonite clay nanoparticles (NPs) (100–200 nm) (Ghormade et al., 2011).
With the aid of nanotechnology, it can enhance the efficacy and accessibility of bioherbicide formulations for field application (Pallavi and Sharma, 2017). Nanoformulations are the outcome of a brand-new area of investigation; if used in weed biological control, it can lead to breakthroughs as nanoparticles with high surface areas need less bioherbicide. A nanoformulation method gaining much attention is nanoencapsulation, which is the coating of numerous components into another material at different sizes in the nano-range. Nanocapsule technology, which incorporates nanosized fungicidal or pesticide molecules, is one of the methods for developing pesticide formulations that kill pests more readily and rapidly (Guan et al., 2008).
METHODS OF APPLICATION OF BIOPESTICIDES
Biocontrol agents are delivered through several means based on the survival nature and mode of infection of the pathogen. It is generally delivered through seed treatment, root dip, soil application and irrigation water. An ideal formulation is expected to facilitate the delivery of the living biocontrol agents in its active state, at the right place, at the right time. While the formulated microbial products must be effective at the site of action and compatible with agronomic practices, they should be easy to apply to and adhere to plant parts such as seeds, tubers, cuttings, seedlings, transplants, and mature plants or be available in the soil medium. The method of application forms an important factor that decides the long term effect of any given bioagent (Hegde and Jahagirdar, 2020).
When living microorganisms are introduced, they may also augment natural beneficial populations to further reduce the damage caused by targeted pathogens (Heydari and Pessarakli, 2010). Biological control agents can be applied using different methods such as seed treatment, root inoculation, cutting and seedling root dip, soil treatment, plant inoculation, furrow application and wound application.
Seed treatment
Biological formulations applied to seeds greatly help to deliver the agents to the spermosphere of plants, where, in general, extremely conducive environments prevail. The BCAs are therefore provided an excellent opportunity to survive, multiply, persist, and exercise control of soilborne phytopathogens. Seed treatment has the potential to deliver microbial agents “in the right amount, at the right place and at the right time” (Prashant et al., 2016). At the time of planting seedlings, the formulated products can be used directly (powders, liquids) without stickers. Powders for seed treatment are formulated by mixing an active ingredient, inert carrier to facilitate product adherence to seeds by mixing seeds with formulated product Additives such as gum arabic and xanthan gum are used to prolong the survival of microbial agents applied to seeds. Alginate hydrogel, used as a seed encapsulation material, maintains the entity in a viable state and protects it from other stresses. Seed priming, in which seeds are mixed with an organic carrier and then moisture content, is brought to a level just below that required for seed treatment which has been used to deliver bioagents. In another process of seed treatment, an industrial film-coating process which was developed for the application of chemicals and biological crop protection agents is being utilized for the application of Trichoderma spp. on radish and cucumber seeds through a film coating.
Treating seeds with bacterial cultures will improve plant production and productivity by protecting plants against phythopathogens. Seed bacterization with peat-based formulation of P. fluorescens strain at the rate of 10 g/kg seed reduced rice blast and sheath blight disease (Vidhyasekaran et al., 1997). Meena et al. (2001) reported that seed treatment with powder formulation of P. fluorescens resulted in significant reduction in root rot incidence of groundnut under field conditions. Treatment of tomato seeds with powder formulation of PGPR (Bacillus subtilis, B. pumilus) reduced symptom severity of tomato mosaic virus and increased the fruit yield (Murphy et al., 2000).
Seedling root dip
This method is suitable for the vegetable crops and rice where transplanting is practiced (Singh and Zaidi, 2002). Nandakumar et al. (2001) reported that P. fluorescens strain mixtures by dipping the rice seedlings in bundles in water containing talc-based formulation of strain mixtures (20 g/l) for 2 h and later transplanting it to the main field suppressed sheath blight incidence. Application of P. fluorescens strain mixtures by dipping the seedling of rice in bucket of water containing talk based formulation containing mixture of 20 g/l for 2h and later transplanting it in the field helps to control sheath blight of rice (Nandakumar et al., 2001).
Seed bio-priming
Biopriming, a seed treatment system that integrates the biological and physiological aspects of disease control, involves coating the seed with fungal or bacterial biocontrol agents. It is a method of treating seeds with microbial inoculants and incubating under warm and moist conditions until just prior to radical emergence. Priming is one of the simple techniques which improve the vigour, seedling establishment, and plant efficiency in the field. Priming allows the early DNA transcription and RNA and protein synthase which repair the damaged parts of the seeds and reduce metabolic exudation. Biopriming may also reduce the amount of biocontrol agents that is applied to seeds (Ramanujam et al., 2010). Nayaka et al. (2008) bioprimed maize seeds with conidial suspension of Trichoderma harzianum for the control of Fusarium verticillioides and fumonisins in maize. It was found that the pure culture of T. harzianum was more effective in reducing the F. verticillioides and fumonisin incidence, followed by talc formulation.
Biopriming could also reduce the amount of biocontrol agents that is applied to the seed. Seed biopriming is successfully used in tomato, brinjal, soybean and chickpea in Tarai region of Uttaranchal (Mishra et al., 2013). Three rhizosphere competent microbial strains, viz., P. fluorescens OKC, Trichoderma asperellum T42 and Rhizobium sp. RH4, individually and in combination in bioprimed seeds of chickpea and rajma in pots and fields showed higher germination percentage and better plant growth in both the crops compared to non-bioprimed control plants. It was also observed that the combined application of the microbes enhanced seed germination and plant growth better than their individual application.
Seed encapsulation
It is a specialized seed-coating process which involves enveloping the seed, microbes, and possibly few other components such as pesticides or micronutrients, in a gelatinous or polymergel matrix, thereby prolonging the survival of microbial inoculants on seed. The gellike matrix allows the cell to remain viable with its catalytic ability for longer duration. This method of delivery system has a distinct advantage of being user friendly and environmentally safe, since the active ingredients are effectively sealed until they are released during germination. Major factors that need to be taken care of while adopting this technique are seed inoculum density, coating stability, both for microbes viability and coat integrity, in association with user feasibility and cost of production. Encapsulation of Bacillus megaterium was attempted by Sivakumar et al. (2014), with bacterial alginate by enriching the bead microenvironment with humic acid, and high viability of encapsulated bacteria with minimum cell loss after 5 months of storage was observed, thereby achieving successful plant-growth promotion of rice seedlings. This novel technique clearly demonstrates that inoculation of encapsulated microbial inoculants promotes plant growth and is feasible for application in agricultural industry.
Foliar spray application
Liquid formulations are being commonly applied on foliar parts of the plants for control of foliar pathogens. The efficacy of the foliar application mainly governs by the microclimate of the crop canopy. The crop canopy has varied concentration of nutrients like amino acids, organic acids, and sugars exuded through stomata, lenticels, hydathodes, and wounds. It affects the efficacy and survival of antagonists in phylloplane. Rice blast (Pyricularia oryzae) can be effectively controlled by foliar spray of talc-based powder formulation of P. fluorescens strain Pf1 (1 kg ha-1). The effectiveness of spraying persisted up to 2 weeks. When the bacterial product was sprayed on plants grown from treated seed, the effectiveness was higher than when spraying was carried out without any prior seed treatment (Vidhyasekaran et al., 1997). Trichoderma species can be applied as foliar spray to control diseases affecting above-ground parts. Biological control of foliar diseases is not so developed as biocontrol of soil-borne diseases. Today liquid bio formulations with high potency, cost-effective with good suspension properties, and good stability are available and being successfully adopted.
Application of Bacillus subtilis to bean leaves reduces the incidence of bean rust. Foliar application of P. fluorescent reduces the severity of bean rust and leaf spot under field conditions. Rice blast (P. oryzae) can be effectively controlled by foliar spray of talc based powder formulation of P. fluorescent strain Pf1 (1 kg/ha). The effectiveness of spraying persisted up to 15 days. When the bacterial product was sprayed on plants grown from treated seed, the effectiveness was higher than when spraying was carried out without any prior seed treatment. The dosage and frequency of application has to be standardized based on the crop value, which could be a reliable and practical approach. Selected strains from many genera of bacteria isolated from these suppressive soils have the potential to reduce plant diseases when applied to the plant root environment (Weller et al., 2002).
Soil application
Soil acts as repertoire of both beneficial and pathogenic microbes; delivering of microbial inoculants to soil will increase the population dynamics of augmented bacterial antagonists and thereby suppress the establishment of pathogenic microbes on to the infection court. Many species of Trichoderma have also been formulated extensively, using cellulosic carriers and binders and modern thin-film coating techniques, in an attempt to introduce them into the rhizosphere regions of seedlings to protect them from diseases such as Rhizoctonia solani and Pythium ultimum. Though drenching of soil with aqueous suspensions of bioagent propagules was carried out, there will not be any even distribution of bioagents in the soil. Soil drenching with T. harzianum has given good control of stem rot of groundnut caused by Sclerotium rolfsii (Kulkarni and Anahosur, 1994). An aqueous drench containing conidia of T. harzianum controlled wilt of chrysanthemum by preventing reinvasion by Fusarium oxysporum. Wilt disease of sunflower was found to be suppressed when P. cepacia strain N24 was applied to the seedbeds at the rate of 500 ml per m2 under greenhouse conditions (Hebber et al., 1991). A technique of enrichment of farmyard manure (FYM) with Trichoderma culture for soil and nursery bed application is widely accepted and appreciated by farmers for soil treatment against soil-borne pathogens. This technique involves less labour and time to multiply Trichoderma culture to manifold for soil application. Vidhyasekaran and Muthamilan (1995) stated that soil application of peat-based formulation of P. fluorescens (Pf1) at the rate of 2.5 Kg of formulation mixed with 25 Kg of well decomposed farm yard manure, in combination with seed treatment, increased rhizosphere colonization of Pf1 and suppressed chickpea wilt caused by Fusarium oxysporum f.sp. ciceris. Application of Trichoderma harzianum Th3-enriched farm yard manure in soil, along with seed treatment, before sowing of chickpea to ward off against root rot caused by Rhizoctonia solani exceptionally reduce the disease and increased yield (Jambhulkar et al., 2015).
Fluid drilling technology
Fluid drilling, also referred to as fluid sowing or gel seeding, is the technology of sowing seeds that have been germinated, using a gel to suspend and transfer them to the seedbed. This delivery system involves the incorporation of biocontrol agents into fluid drill gels. The major advantage of sowing germinated seed compared to dry seed is earlier and more uniform emergence. The gel protects the exposed radicle from mechanical damage and also provides the growing seedling with an initial water source. Unfortunately, the gel tends to attract microorganisms, including soilborne pathogens, which may result in an increased incidence of disease. Unfortunately, the gel tends to attract microorganisms, including soilborne pathogens, which may result in an increased incidence of disease (Prashant et al., 2016).
Microbigation
Applying microbial biocontrol agents to control weeds, soil pathogens, and soil insect through drip irrigation system is called “microbigation”. The conidial suspensions (106 conidia ml-1) can pass through the drippers without causing clogging, regardless of their size, and remained viable. A further advantage could be the limitation of the applied doses to the crop root zone and not the whole field, and therefore a reduction of the costs for treatment (Charudattan, 2001).
Coaggregation assay
Coaggregation is the use of multiple microbial consortia for multipronged attack against phytopathogens and to thrive together in unique ecological niches in ideal proportions, instead of using a single strain, for a single trait. It is a bacteria–bacteria, fungus–fungus, or fungus–bacteria interaction, and the interactions are highly specific that only few or certain species are consortial partners. Sivakumar and Joe (2008) attempted coaggregation of Azorhizobium caulinodans with A. brasilense, A. chroococcum, Bacillus megatherium, and Pseudomonas fluorescens to develop coaggregates with multiple benefits using seed powders of many plants, viz., Moringa oleifera, Strychnos potatorum and Sappindus emarginatus. There is wide scope of using coaggregates to deliver microbial inoculants for obtaining multiple benefits in different crops against various soil-borne pathogens. Studies on coaggregates open up the possibilities for further investigation of the genetic basis of effective coaggregation and also the nature of cellular mechanism.
Consortia application
To improve biocontrol efficacies achieved through the use of a single BCA, there has been increasing interest recently among researchers in using mixtures of BCAs to exploit potential synergistic effect among them. Biocontrol agents applied individually are not likely to perform consistently against all pathogens of the crop or under diverse crop conditions. A combination of biocontrol agents is more likely to have a greater variety of traits responsible for the suppression of one or more pathogens, and also it is likely to have these traits expressed over a wide range of environmental conditions. Accumulating evidence from literature has shown that compatible multiple strains appear to be an important prerequisite for the desired effectiveness of strains and more consistent disease suppression (Ganeshmoorthi et al., 2008). Compatible strains of P. fluorescens (Pf1, Py15 and Fp7) and Bacillus subtilis strains (EPCO 16 and EPC 5) were found to effectively inhibit the growth of Alternaria solani in tomato crop (Sundaramoorthy and Balabaskar, 2012). Similarly, experiments for the biological control of the bacterial blight pathogen revealed that different species of Bacillus applied to rice plants as a seed treatment before sowing, a root dip prior to transplantation, and two foliar sprays prior to inoculation could afford up to 59 % suppression of the disease. These treatments could also bring about a twofold increase in plant height and grain yield (Vasudevan and Gnanamanickam, 2000). Efforts are in progress, including formulation of synergy hypothesis in relation to biocontrol mechanism to exploit microbial mixture for uses in biocontrol of plant diseases.
COMMERCIALIZATION OF BIOPESTICIDES
Commercialization and growers’ acceptance of the biopesticides has been slow to develop, mainly due to the variation in efficacy under the range of the environmental conditions likely to occur in the field. This problem can only be overcome by better understanding the environmental parameters that limit biological control. In addition to this problem, there has been relatively little investment in the development commercially viable products for biological control, partly due to the cost of developing, testing efficacy, and risky, registering and marketing such a product. In recent years many small and large entrepreneurs have entered into the commercial production of biopesticides resulting into the entry of various biopesticides products into the Indian market. Commercialization of biocontrol products is a multi-step process involving a wide range of activities: (1) isolation of micro-organism from the natural ecosystem; (2) Evaluation of bio-agent both in vitro and under glass house conditions; (3) Testing of the best isolate under field conditions; (4) Mass production; (5) Formulation; (6) Delivery; (7) Compatibility; and (8) Registration and release (Prashant et al., 2016).
Most of these are sold commercially as one or more products. The technology of commercialization is still in its initial phase. Many technological problems were overcome and shifts in thinking occurred for these products to reach the shelves. Some of the commercially available biocontrol products available in the market are shown in Table 2.
Table 2: Some of the commercial formulations of biopesticides available in India (Prashant et al., 2016; Saxena et al., 2021)
Product
|
Bioagent(s)
|
Target organism
|
Delivery system/formulation
|
Developing agency
|
Antagon-TV
|
Trichoderma viride
|
Rhizoctonia solani and Macrophomina phaseolina
|
Seed treatment,
soil application
|
Green Tech Agro Products, Coimbatore
|
ARKA Krishi All Rounder and ARKA Plant Growth Booster
|
Pseudomonas fluorescens IIHR Pf-2 (NAIMCC-SB-0038/ITCC B0034) and Trichoderma harzianum IIHR Th-2 (NAIMCC-SF-0033/ITCC 6888)
|
Nematodes: Root knot nematode (Meloidogyne incognita) Bacterial pathogens–Ralstonia solanacearum and Erwinia carotovora Fungal pathogens –Fusarium oxysporum f. sp. Vasinfectum and Fusarium solani
|
Liquid formulation
|
ICAR-Indian Institute of Horticultural Research, Bengaluru
|
ARKA Krishi Kawach
|
Purpureocillium lilacinum (=Paecilomyces lilacinus) IIHR- Pl-2 (NAIMCC-SF-0034/ITCC 6887)
|
Root knot nematode (Meloidogyne incognita) Crops: Brinjal, tomato, carrot and okra
|
Wettable powder
|
ICAR-Indian Institute of Horticultural Research, Bengaluru
|
ARKA Krishi Rakshak
|
Pochonia chlamydosporia (=Verticillium chlamydosporium) IIHR- Vc-3(NAIMCC-SF-0035/ITCC 6898)
|
Root knot nematode (Meloidogyne incognita).
Crops: Brinjal, tomato, carrot and okra
|
Carrier based formulation;
2 × 106 cfu/g
|
ICAR-Indian Institute of Horticultural Research, Bengaluru
|
ARKA Krishi Veera
|
Trichoderma viride ICAR-IIHR Tv-5 (NAIMCC-SF-0032/ITCC 6889)
|
Root knot nematode (Meloidogyne incognita), Fungal pathogens–Fusarium oxysporum f.sp. vasinfectum, F. oxysporum f. sp. lycopersici, Sclerotium rolfsii and F. solani
|
Wettable powder
|
ICAR-Indian Institute of Horticultural Research, Bengaluru
|
Biocon
|
Pseudomonas fluorescens
|
Bacterial wilt and rot diseases
|
Spray
|
Tockalai Experimental Station, Tea Research Association, Jorhat, ASSAM
|
Bioguard
|
Trichoderma viride
|
Fusarium
|
Spray
|
Krishi Rasayan Export Pvt. Ltd. Solan (HP)
|
Bioshield
|
Pseudomonas fluorescens
|
Fusarium, Rhizoctonia,
Pythium, Colletotrichum and Phytophthora
|
Seed treatment, spray
|
POABS Biotech, Kuttoor, Kerala
|
Biotik
|
Metarhizium anisopliae
|
Termites, red ants, root
grubs, grasshoppers
|
Seed treatment, spray, soil application
|
SS Biotech Guwahati Assam
|
Bioderma
|
Trichoderma viride + Trichoderma harzianum
|
Pythium, Rhizoctonia,
Phytophthora and Fusarium
|
Seed treatment and spray
|
Biotech International Ltd., New Delhi, India
|
Bioguard Bt-L
|
Bacillus thuringiensis var. kurstaki DOR Bt-127 (MTCC 5976/NAIMCC-B-01463)
|
Spodoptera litura in soybean
|
Liquid suspension concentrate; 1 × 1011 cfu/mL
|
ICAR-Indian Institute of Oilseeds Research, Rajendranagar, Hyderabad
|
Bio-Pulse
|
Trichoderma harzianum (NAIMCC-SF-0036) and Bacillus amyloliquefaciens (NAIMCC-SB-0052
|
Wilt of chickpea, lentil, pea, pigeon pea; damping off/seedling mortality in papaya; Target fungi: Rhizoctonia, Sclerotium, Sclerotinia, Fusarium, Pythium, Ralstonia, Macrophomina, Bipolaris and Phoma
|
Talc formulation;
1 × 107 cfu/g each
|
ICAR-National Bureau of Agriculturally Important Microorganisms, Mau
|
Biowilt-X
|
Trichoderma harzianum
|
Fusarium oxysporum f.sp.
ciceris and F. udum,
Meloidogyne incognita,
and wilt disease complex (Fusarium + Meloidogyne)
|
Seed treatment
|
Dept. of Plant Pathology, AMU, Aligarh
|
Bionem-X
|
Pochonia chlamydosporia
|
Biocomp-X
|
Pseudomonas fluorescens
|
Ecoderma
|
Trichoderma viride
|
Fusarium, Rhizoctonia,
Pythium and Phytophthora
|
Seed treatment, drenching, soil application, seedling dip
|
Margo Biocontrol Pvt. Ltd., Bangalore
|
Ecofit
|
Trichoderma viride
|
R. solani and Macrophomina phaseolina
|
Seed treatment
|
Hoechast and Schering AgrEvo Ltd., Mumbai
|
Eco-Pesticide
|
Pseudomonas fluorescens
(NAIMCC-SB-0053)
|
Spot blotch of wheat, sheath blight of rice and wilt of tomato and chickpea
|
Talc based; 1 × 108 cfu/g
|
ICAR-National Bureau of Agriculturally Important Microorganisms, Mau
|
Funginil
|
Trichoderma harzianum
|
Botrytis, Pythium, Fusarium, Macrophomina,
Rhizoctonia and Sclerotinia
|
Seed treatment, soil application
|
Crop Health Bioproduct Research Centre, Gaziabad
|
Gliostar
|
Gliocladium. spp.
|
Fusarium, Rhizoctonia,
Sclerotium, and Pythium
|
Seed treatment, drenching
|
GBPUAT, Pantnagar
|
ICAR-Fusicont
|
Trichoderma reesei CSR-T-3 (NAIMCC-SF-0030)
|
Fusarium wilt of banana (Fusarium oxysporum f. sp. cubense Tropical race 4 and race 1)
|
Wettable powder
|
ICAR-Central Soil Salinity Research Institute, Karnal
|
Kalisena SD
Kalisena SL
|
Aspergillus niger AN-27
|
Pythium, Fusarium,
Macrophomina,
Rhizoctonia and Sclerotinia
|
Seed treatment, foliar spray, soil application
|
Cadila Pharmaceuticals
Limited, Ahmedabad
|
Manjari Rakshak
|
Trichoderma afroharzianum (NAIMCC-F-01938)
|
Powdery mildew of grapes
|
Liquid; 5 × 108 cfu/mL
|
ICAR-National Research Centre for Grapes, Pune
|
Manjari Vineguard
|
Trichoderma asperelloides 5R (NAIMCC-SF-0026)
|
Powdery mildew of grapes
|
Liquid formulation
|
ICAR-National Research Centre for Grapes, Pune
|
Maru sena 3
|
Bacillus firmus ICARCAZRI
AZ-1 (MCC 0122)
|
Macrophomina phaseolina in legumes and oil seed crops
|
Carrier based formulation; 1 × 108 cfu/g
|
ICAR- Central Arid Zone Research Institute, Jodhpur
|
Monitor
|
Trichoderma sp.
|
Fusarium, Rhizoctonia,
Sclerotium and Pythium
|
Seed treatment and spray
|
Agricultural and Biotech Pvt. Ltd. Gujarat
|
Mycoguard Bb-L
|
Beauveria bassiana (ITCC 4513)
|
Helicoverpa armigera in pigeon pea
|
Liquid suspension Concentrate
|
ICAR-Indian Institute of Oilseeds Research, Rajendranagar, Hyderabad
|
Myco-Jaal
|
Beauveria bassiana
|
Diamond black moth
|
Spray
|
Pest Control of India, Bangalore
|
NRRI-BBLF
|
Beauveria bassiana TF6
(MTCC 12233)
|
Rice leaf folder
(Cnaphalocrocis medinalis)
|
Talc formulation;
1 × 109 cfu/g
|
ICAR-National Rice Research Institute, Cuttack
|
NRRI-MALF
|
Metarhizium anisopliae
TF19 (MTCC 11644)
|
Rice leaf folder
(Cnaphalocrocis medinalis)
|
Talc formulation;
1 × 109 cfu/g
|
ICAR-National Rice Research Institute, Cuttack
|
Pant Biocontrol
Agent-1 (Biowilt-X)
|
Trichoderma harzianum
|
Pythium, Fusarium,
Macrophomina,
Rhizoctonia and Sclerotinia
|
Seed treatment,
soil application
|
G. B. Pant University of Agriculture Technology,
Pantnagar
|
Pant Biocontrol
Agent-2
|
Pseudomonas fluorescens
|
Fusarium
|
Seed treatment,
soil application
|
G. B. Pant University
of Agriculture Technology,
Pantnagar
|
Phule Trichokill
|
Trichoderma sp.
|
Fusarium, Rhizoctonia and Sclerotium
|
Seed treatment
|
Department of Plant
Pathology, MPKV, Rahuri
|
Pusa Th3
|
Trichoderma harzianum
|
Fusarium, Rhizoctonia and Sclerotium
|
Seed treatment,
soil application
|
Division of Plant Pathology, IARI, Pusa, New Delhi
|
Pusa 5SD
|
Trichoderma harzianum IARI P-4(MTCC 5371)
|
Fusarium oxysporum f. sp. ciceris, Sclerotium rolfsii and Sclerotinia sclerotiorum in chickpea;
Rhizoctonia solani and R. bataticola in chickpea and mungbean; Fusarium
oxysporum f. sp. lycopersici in tomato; Pythium ultimum and R. solani in French bean
|
Wettable powder;
108 cfu/g
|
Indian Council of Agricultural Sciences, New Delhi
|
Shatpada Aphid Kill
|
Beauveria bassiana ICAR-NBAIR Bb-5a (NAIMCC-F-00396)
|
Aphids in chilli and brinjal (Aphis gossypii); cabbage (Brevicoryne brassicae) and cowpea (Aphis craccivora)
|
Oil formulation
|
ICAR-National Bureau of Agricultural Insect Resources, Bengaluru
|
Shatpada All Rounder
|
Pseudomonas fluorescens
NBAIR-PFDWD
|
Thrips spp. in capsicum
And Fusarium wilt of red gram
|
Talc based; 1 × 108 cfu/g
|
ICAR-National Bureau of Agricultural Insect Resources, Bengaluru
|
Shatpada Armour
|
Bacillus thuringiensis var. kurstaki ICAR-NBAIR BT25
|
Armyworm (Spodoptera
frugiperda) in maize
|
Liquid; 1 × 108 cfu/mL
|
ICAR-National Bureau of Agricultural Insect Resources, Bengaluru
|
Shatpada Master Blaster
|
Bacillus albus NBAIR-BATP
|
Fall armyworm
(Spodoptera frugiperda) of maize, tomato pin worm (Tuta absoluta) and Fusarium wilt of cucumber (Fusarium oxysporum f. sp. cucumerinum)
|
Talc based; 1 × 108 cfu/g
|
Indian Council of Agricultural Sciences, New Delhi
|
Shatpada Sucking pest Hit
|
Lecanicillium lecanii ICAR-NBAIR Vl-8 (NAIMCC-F-01851)
|
Aphids in chilli (Aphis gossypii) and cowpea (Aphis craccivora)
|
Oil formulation
1 × 108 cfu/mL
|
ICAR-National Bureau of Agricultural Insect Resources, Bengaluru
|
Shatpada Rugose Whitefly Kill
|
Isaria fumosorosea ICAR-NBAIRP fu5 (NAIMCC-F-02139)
|
Rugose Spiralling Whitefly (Aleurodicus rugioperculatus) in coconut and oil palm
|
Talc; 1 × 108 cfu/g; Oil formulation; 1 × 108 cfu/mL
|
ICAR-National Bureau of Agricultural Insect Resources, Bengaluru
|
Shatpada Terminator
|
Bacillus thuringiensis var. kurstaki ICAR-NBAIR BTG4
|
Legume pod borer (Helicoverpa armigera,
Maruca sp.), diamondback moth (Plutellaxy lostella), spotted stem borer (Chilo partellus), rice leaf folder (Cnaphalocrocis medinalis), brinjal shoot borer (Leucinodes orbonalis) and redhairy caterpillar (Amsacta albistriga)
|
Liquid; 1 × 108 cfu/mL
|
ICAR-National Bureau of Agricultural Insect Resources, Bengaluru
|
Soil Guard
|
Trichoderma viride
|
Fusarium, Rhizoctonia,
Pythium, Colletotrichum and Phytophthora
|
Seed treatment,
soil application
|
POABS Biotech,
Kuttoor, Kerala
|
Sun Agro
Derma
|
Trichoderma viride
|
Fusarium, R. solani,
Macrophomina phaseolina and Colletotrichum
|
Seed treatment, seedling root dip, soil application
|
Sun Agro Chemicals,
Chennai
|
Sun Agro
Derma H
|
Trichoderma harzianum
|
Tricho-X
|
Trichoderma viride
|
Fusarium, Rhizoctonia,
Sclerotium, Pythium
|
Seed treatment, foliar spray, soil application
|
Excel Industries
Limited, Mumbai
|
Trichoderma
|
Trichoderma sp.
|
Fusarium, Rhizoctonia,
Sclerotium and Pythium
|
Seed treatment
|
Innovative Pest control Lab,
Bangalore
|
Trichostar
|
Trichoderma harzianum
|
Fusarium, Rhizoctonia,
Sclerotium and Pythium
|
Seed treatment
|
GBPUAT, Pantnagar
|
Triguard Th-L
|
Trichoderma harzianum Th4d (NAIMCC-F-02188)
|
Phytophthora seedling blight, Macrophomina root rot and Fusarium wilt of safflower and gray mold of castor, Alternaria aster leaf blight and powdery mildew of sunflower
|
Liquid suspension concentrates
|
ICAR-Indian Institute of Oilseed Research, Rajendranagar, Hyderabad
|
Triguard Th-P
|
Trichoderma harzianum Th4 (NAIMCC-F-02188)
|
Phytophthora seedling blight, Macrophomina root rot and Fusarium wilt of safflower and Fusarium wilt of castor and Aspergillus root rot in groundnut
|
Wettable powder
|
ICAR-Indian Institute of Oilseed Research, Rajendranagar, Hyderabad
|
Commercialization challenges of biopesticides in India
India is giving more emphasis on using biopesticides rather than chemical pesticides. The major factor which drives the use of biopesticides is the concern of sustainable agriculture. Various factors help in the growth of biopesticide markets like increasing adoption of organic products, concerned about the harmful effects of using synthetic pesticides, and the management of integrated pest management. In the biopesticides market, various companies are competing to hold the largest share in the market and focus on the quality of product and promotion.
Central and state agricultural universities and various ICAR institutes are major government agencies involved in commercial production of different biopesticides. Data derived from DPPQS shows that amongst the government biocontrol units, production is carried out only by a few of them. In the southern region, Tamil Nadu Agricultural University (TNAU), Coimbatore, Central Plantation Crops Research Institute (CPCRI), Indian Institute of Horticultural Research, Bangalore, Central Research Institute for Dryland Agriculture, Hyderabad, Directorate of Oilseed Research (ICAR), Hyderabad and Kerala Agricultural University (KAU), Kerala are known to have dedicated biopesticide production units. In the northeast, Assam Agriculture University and Central Agricultural University, Manipur are producing biopesticides against invasive pests. In north, Indian Agricultural Research Institute (IARI), New Delhi, Punjab Agricultural University (PAU), Punjab, G. B. Pant University of Agriculture & Technology (GBPUA & T), Uttarakhand are involved in the production of biopesticides. Whereas in Central Uttar Pradesh, Indian Institute of Sugarcane Research (IISR) and Central Institute for Subtropical Horticulture, and Directorate of Plant Protection Quarantine & Storage in Lucknow, which works under The Central Integrated Pest Management Centre are the major government agencies involved in manufacturing of biopesticides. Apart from these, with governmental support, several Krishi Vigyan Kendras (KVK) and State biocontrol labs have also been developed, which are producing biopesticides for managing the local demand. National Agricultural Cooperative Marketing Federation of India (NAFED) has also started to promote the use of biopesticides.
In India, most of the biopesticide production takes place in public sector units. It is estimated that the public sector contributes to almost 70% of the biopesticides production. Major companies like Biotech International Ltd. (New Delhi), International Panaacea Ltd., New Delhi, Ajay Biotech (India) Ltd., Pune, Bharat Biocon Pvt. Ltd. (Chhattisgarh), Microplex Biotech & Agrochem Pvt (Mumbai), Excel Crop Care Ltd., Mumbai and Govinda Agro Tech Ltd. (Nagpur), Jai Biotech Industries, Satpur, Nasik, Ganesh Biocontrol System, Rajkot, Gujarat Chemicals and Fertilizers Trading Company, Baroda, Gujarat Eco Microbial Technologies Pvt. Ltd., Vadodara, Chaitra Agri Organics, Mysore, Deep Farm Inputs (P) Ltd., Kan Biosys Pvt. Ltd., Pune Indore Biotech Inputs & Research Pvt. Ltd., Indore, Romvijay Biotech Pvt. Ltd., Pondichery Neyattinkara, Kerala, Devi Biotech (P) Ltd., Madurai, T. Stanes & Company Ltd., Coimbatore, Harit Bio Control Lab., Yavatmal and Hindustan Bioenergy Ltd., Lucknow. Some foreign companies have also entered in biopesticides production, but most of them are working in collaboration with Indian companies. For example, Lupin Agrochemicals, a Bombay based company, is now working with US-based Abbott Laboratories. Sugar and distillery companies such as KCP Sugar & Industries Corporation Ltd. (Andhra Pradesh), Rajshree Sugars & Chemicals Ltd. (Tamil Nadu), New Swadeshi Sugar Mills (Bihar), and Bannari Amman Sugars Ltd. (Tamil Nadu) are investing in developing the market of biopesticides. It indicates that the demand for biopesticides is gradually increasing (Kandar, 2021).
There are various challenges to commercialization of biopesticides in the Indian market, and the responsibility to address these challenges lies with the private sector but also with other important arms of the society like academia, state and central government agencies, public and private researchers and funding bodies, marketing professionals, etc. In fact, all the sectors involved in disease control and pest management must join hands and address these commercialization issues together (Fig. 7).
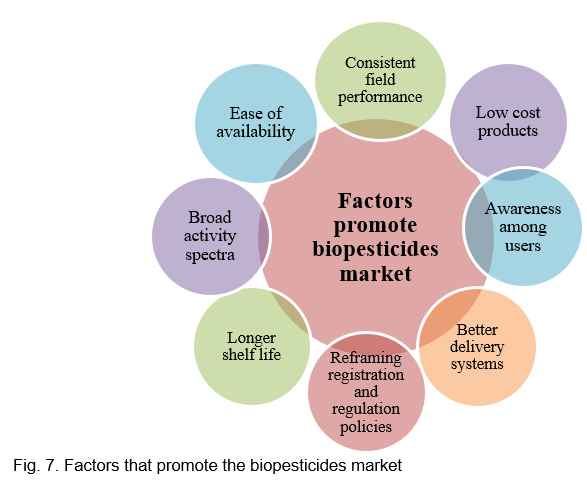
Various factors affecting the commercialization of biopesticides in the Indian agricultural market are as follows.
Awareness and information gap
The lack of awareness, knowledge, and confidence in farmers is one of the chief reasons behind the lagging of these eco-friendly pest control alternatives. Biopesticide packs carry detailed instructions and precautions for storage and usage. In spite of this, usage methods are often not clear to farmers. Sometimes the farmers lack the essential skills required for adopting the biopesticides in their agricultural farms.
Low reliability and inconsistent field performance
Lack of stability on the effect of biopesticides has been the primary concern for farmers. Since most of the microorganisms are living, several factors like temperatures, moisture, pH, exposure to ultraviolet radiation, and soil factors adversely affect their efficacy (Arora et al., 2010). Furthermore, the production of biopesticides is prone to contamination, which results in low count of active microorganisms, thus reducing the efficacy of microorganisms and leading to inconsistent field performance.
Poor quality and shelf life
Contamination and low cell count are the main concerns of the farmers and distributers (Alam, 2000). It is difficult to maintain the sterile conditions for a longer period. Contamination results in death of active microorganisms, and due to low microbial count, their performance is poorly deprived and uneven. This further leads to low shelf life and inconsistent performance of product in field conditions.
Huge investment and lack of profit
Biopesticides are high-technology products which are developed and maintained under completely sterile conditions. A huge capital investment has to be made not only in the initial development stages but also during its packaging, storage, and distribution. Screening of suitable strains and R&D issues add to the budget. Large scale screening of strains with biological activity is still required (Bashan, 1998). In general, firms with larger production facilities are expected to invest more on networks to understand and access the market. Besides, there is high risk and less profit associated with it. All these factors make biopesticide production a costly business, and companies will only develop these products if there is a long-term profit in doing so.
Health and ecological risks
Biopesticides may pose some adverse health effects if these are not used according to the guidelines mentioned on the labels of the commercial product. Biopesticides containing Bacillus thuringiensis as active ingredient are not reported to show any major adverse effects on human health, but in some cases, occupational exposure has confirmed health risks (Doekes et al., 2004). Studies on fungal biopesticides report that spore of entomopathogenic fungi such as Trichoderma, M. anisopliae, and B. bassiana may cause allergies in immune-compromised patients to farmers (Darbro and Thomas, 2009; Keswani et al., 2014).
FUTURE PROSPECTS
Despite several challenges, we believe that the microbial pesticide market in India has a bright future. Biopesticide research on the subcontinent is at a relatively early stage but evolving rapidly with increasing focus on identifying effective indigenous isolates, improving formulation and manufacturing technologies that reduce costs and enhance the shelf life of the products. Additional markets need to be explored. For example, there is little emphasis on the application of biopesticides for post-harvest use, such as grain silos and food storage systems where reside concerns and pest losses are an issue. Since rural famers in India receive little agricultural education or training, universities, federal and state agencies, and pesticide manufacturers and distributers need to work with farmers and other stakeholders to improve their knowledge and acceptance of biopesticides. Some existing initiatives may play a role in development, marketing, sale and use of microbial biopesticides. Examples include farmer field school programs, and outreach programs of pesticide suppliers who deal with farmers (input dealers). These provide opportunities to educate farmers about IPM and to differentiate between genuine and unregistered or fake biopesticides (Kaur and Kaur, 2018). In 2003, the National Institute of Agricultural Extension Management (MANAGE), an organization of the Ministry of Agriculture and Farmers Welfare, Government of India, initiated a Diploma in Agricultural Extension Services for Input Dealers (DAESI) program. The program disseminates crop production and protection technologies to farmers through input dealers, where most farmers obtain their pesticides. So far, over 4,000 candidates have been trained under this program. Demonstration of successful microbial biopesticide technologies among more progressive farmers may enhance local adoption. Consequently, most biopesticides in India are used by farmers in semi- and peri-urban areas. The current registration process for biopesticides needs to be reviewed. Current toxicological data requirements are out-dated for these products and dossier submission requirements should be streamlined for microbial biopesticides to reduce costs and accelerate the registration process. Similarly, legislation should address the patenting process for microbial products. Based on known product quality-control issues, and the proliferation of unregistered products, the effectiveness of current Central Insecticides Board and Registration Committee (CIBRC) policies to maintain the quality, accessibility and affordability of biopesticides may also require review. The adoption of future international registration standards for biopesticides may ultimately help facilitate this process (FAO and WHO, 2017).
CONCLUSION
The increasing concern of consumers on the one hand and government on the other hand about the problems associated with synthetic chemicals for pest control and on food safety has led growers to find new eco-friendly methods to replace the current chemical-based practices. The use of biopesticides as supplement has emerged as promising alternative to chemical pesticides and their demand is rising steadily in all parts of the India. Organic farming and agricultural produce free from pesticide residue would positively permit greater acceptance of biopesticides among the farmers. Biopesticide research is young and evolving and requires more attention and reliability. The challenges in broad usage of biopesticides in India are related to the efficacy, shelf-life, production methods, narrow range of host or target pathogens/pests, poor performance in the field, problems in the delivery system, economics, and regulations. In this context, discovery of new substances and research on formulation and delivery would boost commercialization and use of biopesticides. However, a commitment to the development of high quality products, a more responsive registration process, government support for research and extension and productive collaboration among researchers, industry, and farmers will help biopesticides to gain more mainstream acceptance in sustainable crop production practices.
REFERENCES
Aeron, A., R. C. Dubey, D. K. Maheshwari, P. Pandey, V. K. Bajpai, S. C. Kang. 2011. Multifarious activity of bioformulated Pseudomonas fluorescens PS1 and biocontrol of Sclerotinia sclerotiorum in Indian rapeseed (Brassica campestris L.). Eur. J. Plant Pathol. 131: 81–93.
Akshata, L. T., D. D. Guldekar, S. R. Potdukhe, S. S. Kale and A. Kumar. 2018. Shelf life study and antagonistic activity of Trichoderma viride in different oil formulations. Int. J. Curr. Microbiol. Appl. Sci. 7(11): 225–230.
Alam, G. 2000. A study of biopesticides and biofertilisers in Haryana, India.Gatekeeper series no. 93 IIED, London.http://pubs.iied.org/pdfs/6348IIED.pdf.
Aneja, K. R., S. A. Khan and A. Aneja. 2016. Biopesticides an eco-friendly pest management approach in agriculture: status and prospects. Kavaka. 47: 145–154.
Antonious, G. F. 2003.Impact of soil management and two botanical insecticides on urease and invertase activity. J. Environ. Sci. Health. 38(4): 479–488.
Arora, N. K., E. Khare and D. K. Maheshwari. 2010. Plant growth promoting rhizobacteria: constraints in bioformulation, commercialization and future strategies. In: Maheshwari, D. K. (ed) Plant growth and health promoting bacteria. Springer, Berlin, 97–116.
Bailey, K. L., S. M. Boyetchko and T. Längle. 2010. Social and economic drivers shaping the future of biological control: a Canadian perspective on the factors affecting the development and use of microbial biopesticides. Biological Control. 52(3): 221–229.
Bashan, Y. 1998. Inoculants of plant growth promoting bacteria use in agriculture. Biotech. Adv. 6: 729–770.
Bashan, Y., L. E. de-Bashan, S. R. Prabhu and J. P. Hernandez. 2014. Advances in plant growth-promoting bacterial inoculant technology: Formulations and practical perspectives (1998–2013). Plant Soil. 378(1): 1–33.
Brahmachari, G. 2004. Neem-An omnipotent. Chembiochem. 5: 408–421.
Brar, S. K., M. Verma, R. D. Tyagi and J. R. Valéro. 2006. Recent advances in downstream processing and formulations of Bacillus thuringiensis based biopesticides. Process Biochem. 41(2): 323–342.
Charudattan, R. 2001. Biological control of weeds by means of plant pathogens: significance for integrated weed management in modern agro-ecology. BioControl. 46: 229–260.
Dar, A. S., Z. H. Khan, A. A. Khan, S. B. Ahmad. 2019. Biopesticides-its prospects and limitations: An overview. Perspective in Animal Ecology and Reproduction.296-314, Astral International, New Delhi, India.
Darbro, J. M. and M. B. Thomas. 2009. Spore persistence and likelihood of aeroallergenicity of entomopathogenic fungi used for mosquito control. American J. Trop. Med. Hyg. 80: 992–997.
Djiwanti, S. R. and S. Kaushik. 2019. Nanopesticide: Future application of nanomaterials in plant protection. In: Prasad R (ed) Plant nanobionics, Nanotechnology in the Life Sciences. Springer, 255–298.
Dobhal, A., G. M. Hegde, S. Jahagirdar and K. Mirajkar. 2021. Exploring the potential of oil based formulation of Pseudomonas fluorescens against Bipolarissorokiniana causing spot blotch of wheat and its quality content. Paper presented at virtual national symposium on “Sustainable plant health management amidst covid pandemic: Challenges and strategies, ICAR-Central Plantation Crops Research Institute, Kasaragod, 1–3 December, 2021.
Doekes, G., P. Larsen, T. Sigsgaard and J. Baelum. 2004. IgE sensitization to bacterial and fungal biopesticides in a cohort of Danish greenhouse workers: the BIOGART study. American J. Ind. Med. 46: 404–407.
Environmental Protection Agency (EPA) USA. 2012. Regulating Biopesticides.
FAO and WHO. 2017. International Code of Conduct on Pesticide Management: Guidelines for the Registration of Microbial, Botanical and Semiochemical Pest Control Agents for Plant Protection and Public Health Uses. Food and Agriculture Organization of the United Nations World Health Organization, Rome, pp. 2017.
Filho, A. B., A. B. Alves, N. T. August, R. M. Pereira and L. F. A. Alves. 2001. Stability and persistence of two formulations containing Anticarsiagemmatalis Nuclear Polyhedrovirus (AgMNPV). J. Neotrop. Entomol. 30: 411–416.
Ganeshmoorthi, P., T. Anand, V. Prakasam, M. Bharani, N. Ragupathi, et al. 2008. Plant growth promoting rhizobacteria (PGPR) bioconsortia mediates induction of defense related proteins against infection of root rot pathogen in mulberry plants. J. Plant Interact. 3: 233–244.
Ghormade, V., M. V. Deshpande and K. M. Paknikar. 2011. Perspectives for nano-biotechnology enabled protection and nutrition of plants. Biotechnol. Adv. 29(6): 792–803.
Glare, T., J. Caradus, W. Gelernter, T. Jackson, N. Keyhani, J. Köhl, P. Marrone, L. Morin and A. Stewart. 2012. Have biopesticides come of age? Trends in Biotechnol. 30(5): 250–258.
Guan, G., B. Liu, Z. Wang and Z. Zhang. 2008. Imprinting of molecular recognition sites on nanostructures and its applications in chemosensors. Sensors. 8(12): 8291–8320.
Gupta, S. and A. K. Dikshit. 2010. Biopesticides: An ecofriendly approach for pest control. J. Biopest. 3: 186–188.
Hazra, D. K. 2015. Recent advancement in pesticide formulations for user and environment friendly pest management. Int. J. Res. Rev. 2: 35–40.
Hebber, P., O. Berge, T. S. Heulin and P. Singh. 1991. Bacterial antagonists of sunflower (Helianthusannuus L.) fungal pathogens. Plant Soil. 133:131–140.
Hegde, G. M. and S. Jahagirdar, 2020.Trichoderma: A potential millennium microbe for sustainable management of field and horticulture crop diseases: An overview. J. Farm Sci. 33(2): 159–172.
Heydari, A. and M. Pessarakli. 2010. A review on biology control of fungal plant pathogen using microbial antagonists. J. Biol. Sci. 10: 273–290.
Hubbard, M., R. K. Hynes, M. Erlandson, K. L. Bailey. 2014. The biochemistry behind biopesticide efficacy. Sustainable Chemical Processes. 2(1): 1–8.
Ingram, C. W., M. S. Coyne, D. W. Williams. 2005. Effects of commercial diazinon and imidacloprid on microbial urease activity in soil and sod. J. Environ. Quality. 34(5):1573–1580.
Isman, M. B. 1997. Neem and other botanical insecticides: barriers to commercialisation. Phytoparasitica. 25(4): 339-344.
Jambhulkar, P. P. and P. Sharma. 2014. Development of bioformulation and delivery system of Pseudomonas fl uorescens against bacterial leaf blight of rice (Xanthomonas oryzae pv. oryzae ). J. Environ. Biol. 35(5): 843–849.
Jambhulkar, P. P., P. Sharma and M. L. Meghwal. 2015. Additive effect of soil application with Trichoderma enriched FYM along with seed treatment and drenching with Trichoderma formulation for management of wet root rot caused by Rhizoctonia solani in chickpea. J. Pure Appl. Microbiol. 9(1): 405–412.
John, R. P., R. D. Tyagi, S. K. Brar, R. Y. Surampalli and D. Prévost. 2011. Bio-encapsulation of microbial cells for targeted agricultural delivery. Crit. Rev. Biotechnol. 31(3): 211–226.
Kah, M. and T. Hofmann. 2014. Nanopesticide research: Current trends and future priorities. Environ. Int. 63: 224–235.
Kala, S., N. Sogan and A. Agarwal, et al. 2020. Biopesticides: Formulations and delivery techniques. In: Natural remedies for pest, disease and weed control. Academic Press, Elsevier, 209–220.
Kandar, P. 2021. Phytochemicals and biopesticides: Development, current challenges and effects on human health and diseases. J. Biomed. Res. 2(1): 3–15.
Kaur, K. and P. Kaur. 2018. Agricultural extension approaches to enhance the knowledge of farmers. Int. J. Curr. Microbiol. Appl. Sci. 7: 2367–2376.
Keswani, C., H. Dilnashin, H. Birla and S. P. Singh. 2019. Regulatory barriers to Agricultural Research commercialization: A case study of biopesticides in India. Rhizosphere. 11:100155.
Keswani, C., S. Mishra, B. K. Sarma, S. P. Singh and H. B. Singh. 2014. Unraveling the efficient application of secondary metabolites of various Trichoderma. Appl Microbiol Biotechnol. 98: 533–544.
Knowles, A. 2008.Recent developments of safer formulations of agrochemicals. Environmentalist. 28: 35–44.
Kour. D., K. L. Rana, A. N. Yadav, N. Yadav, M. Kumar, V. Kumar, et al. 2020. Microbial biofertilizers: bioresources and eco- friendly technologies for agricultural and environmental sustainability. Biocatal. Agric. Biotechnol. 23: 101487. https://doi.org/10.1016/j.bcab.2019.101487.
Kranthi, K. R., D. Russell, R. Wanjari, M. Kherde, S. Munje, N. Lavhe and N. Armes. 2002. In-season changes in resistance to insecticides in Helicoverpaarmigera (Lepidoptera: Noctuidae) in India. J. Economic Entomol. 95(1): 134–142.
Kulkarni, S. A. and Anahosur, K. H. 1994.Effect of age of groundnut plant to infection of Sclerotium rolfsii Sacc.a causal agent of stem rot disease. Karnataka J. Agric. Sci. 7:367–368.
Kumar, A., A. K. Chaturvedi, K. Yadav, K. P. Arunkumar, S. K. Malyan, P. Raja and A. N. Yadav. 2019. Fungal phytoremediation of heavy metal-contaminated resources: current scenario and future prospects. In: Recent Advancement. In: White Biotechnology Through Fungi. 437-461, Springer.
Kumar, S. 2012. Biopesticides: a need for food and environmental safety. J. Biofert. Biopest. 3(4): 1–3.
Kumar, S. and A. Singh. 2015. Biopesticides: present status and the future prospects. J. Fertilizers and Pesticides, 6(2): 100–129.
Kumari, S., S. C. Kumar, M. N. Jha, R. Kant, U. Singh and P. Kumar. 2014. Microbial pesticide: A boom for sustainable agriculture. Int. J. Scientific Engg. Res. 5(6): 1394–1397.
Lengai, G. M. W. and J. W. Muthomi. 2018. Biopesticides and Their Role in Sustainable Agricultural Production. J. Biosci. Med. 6(6): 7–41.
Littlefield-Wyer, J. G., P. Brooks, M. Katouli. 2008. Application of biochemical fingerprinting and fatty acid methyl ester profiling to assess the effect of the pesticide Atradex on aquatic microbial communities. Environ. Pollution. 153(2): 393–400.
Marrone, P. G. 2007. Barriers to adoption of biological control agents and biological pesticides. CAB Reviews: Perspectives. In: Agriculture, Veterinary Science, Nutrition and Natural Resources 2(15). CABI Publishing, UK.
Mazid, S., J. C. Kalida and R. C. Rajkhowa. 2011. A review on the use of biopesticides in insect pest management. Int. J. Sci. Adv. Technol. 1(7): 169–178.
Mishra, D. S., A. Kumar, C. R. Prajapati, A. K. Singh and S. D. Sharma. 2013. Identification of compatible bacterial and fungal isolate and their effectiveness against plant disease. J. Environ. Biol. 34: 183–189.
Mishra, J., V. Dutta and N. K. Arora. 2020. Biopesticides in India: technology and sustainability linkages. Biotech. 10(5): 1–12.
Monkiedje, A. and M. Spiteller. 2002. Effects of the phenylamide fungicides, mefenoxam and metalaxyl on the microbiological properties of a sandy loam and a sandy clay soil. Biol. Fertility Soils. 35(6): 393–398
Murphy, J. F., G. W. Zehnder, D. J. Schuster, E. J. Sikora, J. E. Polston and J. W. Kloepper (2000). Plant growth-promoting rhizobacteria mediated protection in tomato against tomato mottle virus. Plant Dis. 84: 779–784.
Murugasridevi, K., S. Jeyarani, K. Ramaraju and S. M. Kumar. 2021. Oil based formulation of Beauveria bassiana (BB 112) against onion Thrips tabaci Lindeman. Indian J. Entomol. e20405. DOI:10.55446/IJE.2021.20.
Nandakumar, R., S. Babu, R. Viswanathan, J. Sheela and R. Samiyappan. 2001 Induction of systemic resistance in rice against sheath blight diseases by plant growth promoting rhizobacteria. Soil Biol. Biochem. 33: 603–612.
Nawaz, M., J. I. Mabubu and H. Hua. 2016. Current status and advancement of biopesticides: Microbial and botanical pesticides. J. Entomol. Zool. Stud. 4(2): 241–246.
Nayaka, S. R., A. C. Niranjana, S. Uday Shankar, M. S. Niranjanraj, H. S. Reddy, C. N. Prakash and Mortensen. 2008. Archives Phytopath. Plant Prot., 43(3): 264–282.
Organisation for Economic Co-operation and Development (OECD). 2009. Series on pesticides No. 448. Report of Workshop on the Regulation of Biopesticides: Registration and Communication Issues. http://www.oecd.org/dataoecd/3/Collego55/43056580.pdf.
Osman, G. 2015. Already R, Assaeedi A, Althubiani A. Bioinsecticide Bacillus thuringiensis a comprehensive review.Egyptian J. Biological Pest Control. 25: 271–288.
Pallavi, D. Chandra and A. K. Sharma. 2017. Commercial microbial products: Exploiting beneficial plant-microbe interaction. In: Singh D, Singh H, Prabha R (eds) Plant-microbe interactions in agro-ecological perspectives. Springer, Singapore. 643–699.
Pavela, R. 2014. Limitation of plant biopesticides. In: Advances in Plant Biopesticides. 347–359, Springer, New Delhi, India.
Peshin, R, R. S. Bandral, W. J. Zhang, et al. 2009. Integrated Pest Management: A Global Overview of History, Programs and Adoption. In: Integrated Pest Management: Innovation-Development Process (Vol. 1) (Peshin R, Dhawan AK, eds). 1-50, Springer, Netherlands .
Peshin, R. and W. J. Zhang. 2014. Integrated Pest Management and Pesticide Use. In: Integrated Pest Management: Pesticide Problems (Vol. 3) (eds, Pimentel D, Peshin R,). 1-46, Springer, Netherlands.
Prashant, P., Jambhulkar, P. Sharma and R. Yadav. 2016. Delivery Systems for Introduction of Microbial Inoculants in the Field, D.P. Singh et al. (eds.), Microbial Inoculants in Sustainable Agricultural Productivity, 199–218.
Prithiva, J. N., N. Ganapathy and S. Jeyarani. 2017. Efficacy of different formulations of Beauveria bassiana (Bb 112) against Bemisia tabaci on tomato. J. Entomol. Zool. Stud. 5(4): 1239–1243.
Ramanujam, B., R. D. Prasad, S. Sriram and R. Rangeswaran. 2010. Mass production, formulation, quality control and delivery of Trichoderma for plant disease management. J. Plant Protec. Sci. 2(2): 1–8.
Sangamithra, S., S. Jeyararani, K. Ramaraju. 2015. Compatibility of different oils with Beauveria bassiana, a potential entomopathogenic fungus. The Bios. 10(3): 1113–1117.
Satapathy, S. 2018. Regulatory norms and quality control of bio-pesticides in India. Int. J. Curr. Microbiol. Appl. Sci. 7(11): 3118–3122.
Saxena, A. K., Chakdar H, M. Kumar. et al. .2021. ICAR technologies: Biopesticides for eco-friendly pest management. Indian Council of Agricultural Research, New Delhi.
Schisler, D. A., P. J. Slininger, R. W. Behle and M. A. Jackson. 2004. Formulation of Bacillus sp. for biological control of plant diseases. Phytopathol. 94: 1267–1271.
Schmutterer, H. 1985. The Neem Tree Azadirachtia indica A. Juss and Other Meliaceous Plants: Sources of Unique Natural Products For Integrated Pest Management, Medicine, Industry And Other Purposes. VCH Weinheim, Germany.
Sharma, S. K., A. K. Upadhyay, M. A. Haque, et al. 2005. Village-Scale evaluation of mosquitoe nets treated with a tablet formulation of deltamethrin against malaria vector. Med. Vet. Entomol. 19(3): 286–292.
Singh, U. S. and N. W. Zaidi. 2002. Current Status of formulation and delivery of fungal and bacterial antagonists for disease management in India, In Microbial Biopesticide Formulations and Application (EdsRabindra, R. J. S. S. Hussaini and B. Ramanujam) Project Directorate of Biological Control, Bangalore pp. 168–179.
Sivakumar, P. K. and M. M. Joe. 2008. Development of coaggregated cells as bioinoculants using plant seed powders-A novel delivery system for rice grown under lowland condition. Agric. Conspec. Sci. 73(4): 253–257.
Sivakumar, P. K., R. Parthasarthi and V. P. Lakshmipriya, 2014. Encapsulation of plant growth promoting inoculant in bacterial alginate beads enriched with humic acid. Int. J. Curr. Microbiol. App. Sci. 3(6): 415–422.
Smith, R. F. and R. Van den Bosch. 1967. Integrated control. In: Pest control: Biological, Physical and Selected Chemical Methods (eds, Kilgore W. W. and R. L. Doutt). 295–340, Academic Press, New York, USA.
Sridevi, K. M., S. Jeyarani and K. Ramaraju. 2018. Evaluation of oil-based formulation of Beauveriabassiana (Bb 112) (Bals.) Vuill.and delivery methods for the management of chilli thrips, Scirtothripsdorsalis Hood. J. Biol. Control. 32(1): 62–67.
Sundaramoorthy, S. and P. Balabaskar. 2012. Consortial effect of endophytic and plant growth promoting rhizobacteria for the management of early blight of tomato Incited by Alternaria Solani . J. Plant Pathol. Microbiol. 3: 145.
Tijjani, A., K. A. Bashir, I. Mohammed, A. Muhammad, A. Gambo and H. Musa. 2016. Biopesticides for pest's control: A review. J. Biopest. Agri. 3(1): 6–13.
Ting, A. S. Y., S. W. Mah and C. S. Tee. 2010. Identification of volatile metabolites from fungal endophytes with biocontrol potential towards Fusarium oxysporum f. sp. cubense race 4. American J. Agr. Biol. Sci. 5: 177–182. 10.3844/ajabssp.2010.177.182.
Vassilev, N., I. Nikolaeva and M. Vassileva. 2005. Polymer-based preparation of soil inoculants: Applications to arbuscular mycorrhizal fungi. Rev. Environ. Sci. Biotechnol. 4: 235–243.
Vasudevan, P. and S. S. Gnanamanickam. 2000. Progress and prospects for biological suppression of rice diseases with bacterial antagonists. Biological control and plant growth promoting rhizobacteria (PGPR) for sustainable agriculture, Hyderabad, India, April 3–4.
Vemmer, M. and A. V. Patel. 2013. Review of encapsulation methods suitable for microbial biological control agents. Biol. Control. 67(3): 380–38.
Vendan, R. T. and M. Thangaraju. 2006. Development and standardization of liquid formulation for Azospiriliumbioinoculant. Indian J. Microbiol. 46(4): 379.
Vendan, S. E. 2016. Current scenario of biopesticides and eco-friendly insect pest management in India. South Indian J. Biol. Sci. 2(2): 268–271.
Vidhyasekaran, P. and Muthamilan, M. 1995. Development of formulations of Pseudomonas fluorescens for control of chickpea wilt. Plant Dis. 79: 782–786.
Vidhyasekaran, P., R. Rabindran, M. Muthamilan, K. Nayar, K. Rajappan, N. Subramanian and K. Vasumathi. 1997. Development of powder formulation of Pseudomonas fluorescens for control of rice blast. Plant Pathol. 46: 291–297.
Wang, M. C., M. Gong, H. B. Zang, X. M. Hua, J. Yao, Y. J. Pang and Y. H. Yang. 2006. Effect of methamidophos and urea application on microbial communities in soils as determined by microbial biomass and community level physiological profiles. J. Environ. Sci. Health. 41(4): 399–413.
Weller, D. M., J. M. Raaijmakers, B. B. McSpadden Gardner and L. S. Thomashow. 2002. Microbial populations responsible for specific soil suppressiveness to plant pathogens. Ann. Rev. Phytopathol. 40: 308–348.
Yadav, A. N., R. Kumar, S. Kumar, V. Kumar, T. Sugitha, B. Singh, et al. 2017. Beneficial microbiomes: biodiversity and potential biotechnological applications for sustainable agriculture and human health. J. Appl. Biol. Biotechnol. 5: 45–5.
Yadav, N. and A. N. Yadav. 2019. Biodegradation of biphenyl compounds by soil microbiomes. Biodiversity Int. J. 3: 37–40.
Yadav, R., S. Singh and A. N. Singh. 2022. Biopesticides: Current status and future prospects. Proc. Int. Acad. Ecol. Environ. Sci. 12(3): 211–233.
Zhang, W. J. 2018. Global pesticide use: Profile, trend, cost / benefit and more. Proceedings of the International Academy of Ecology and Environmental Sciences, 8(1): 1–27.
Zhang, W. J. and G. H. Liu. 2022. A Mathematical Model to Simulate the Development of Insecticide Resistance: Assessment of IPM Technologies for Reducing Insecticide Resistance. In: Advances in Integrated Pest Management Technology: Innovative and Applied Aspects (Tanda, A. S., ed). 121-143, Springer Nature, Switzerland.
Zhang, W. J., F. B. Jiang and J. F. Ou. 2011. Global pesticide consumption and pollution: with China as a focus. Proc. Int. Acad. Ecol. Environ. Sci. 1(2): 125–144.
Formulation, Application and Commercialization of Biopesticides in India
DOI: https://doi.org/10.56669/KSHW3457
ABSTRACT
Keeping a tab on the agricultural production systems on a maintained level is a challenge for the future as the Indian population is increasing at a rapid pace. Plant diseases cause considerable losses in crop production and storage. Chemical pesticides are commonly used to control pests, which cause harmful impacts on the environment and non-target living systems, including human beings. Biopesticides are natural and a better substitute for chemical pesticides and provide an alternative for crop protection worldwide. The trend of biopesticides consumption in India has shown a drastic increase in use over time which stood at 8,647 and 8,898 metric tons in 2020-21 and 2021-22, respectively. Although the value of eco-friendly pest management in sustainable agriculture has been well recognized, only very little is being adapted at the field level. But making it reach to the field with a suitable delivery method maintaining consistent performance is the next most important challenge. The bioagents cannot be applied as spore suspension in field but are applied as powdered or liquid formulation primarily through seed treatment, soil application, root dip or foliar application. In India, as compared to chemical pesticides, biopesticides production, utilization, and consumption are much lower due to a lack of research advancements, innovation and policies. To increase the use of biopesticides at the country level, it is necessary to understand their mode of action, contribution to sustainable agriculture and their effects on human health and plant diseases. Thus, in this article we have discussed about overview of biopesticides, their classification, formulations, application, commercialization, current status and prospects.
Keywords: Application and Commercialization, Biopesticides, Formulations, India
INTRODUCTION
Agriculture is an anthropogenic activity but adversely affected by various pests such as thousands of insects, fungi, viruses, bacteria, nematodes, weeds and other living forms, leading to crop yield and production quality reduction (Kumar, 2012). With the advent of agriculture, plant diseases became a problem. Over the past 50 years, the most common method for pest control has been the extensive use of chemical pesticides (Peshin et al., 2009; Peshin and Zhang, 2014; Zhang et al., 2011; Zhang, 2018). However, the high effectiveness and ease of utilization of these chemicals can result in environmental contamination and the presence of pesticide residues in food, in addition to social and economic problems. By using agrochemicals, agriculture productivity has increased rapidly. In India, 381 g/ha of chemical and synthetic pesticides is being consumed annually and the rate of consumption rising from 2 to 5% yearly. However, the pesticides consumption is relatively lower in India than the worldwide consumption of 500 g/ha (Vendan, 2016). Chemical and synthetic pesticides are used in excessive amounts to control pests in crop fields and it also deteriorates the soil fertility and ecosystem (Zhang et al., 2011; Zhang, 2018). But it is the most effective tool for integrated pest management (IPM) (Kumari et al., 2014). However, they also have adverse impacts on water quality, soil health, product quality, and developed problems such as insecticide resistance, genetic variation in plants, toxic residues, and food safety. The usage of pesticides may damage the indigenous microorganisms, disturb the soil ecosystem, also reduce the soil enzymatic activities that act as a "biological index" of soil fertility, and may also affect human health via the food chain (Antonious, 2003; Ingram et al., 2005; Littlefield-Wyeret al., 2008; Monkiedje and Spiteller, 2002; Wang et al., 2006). It has become necessary to develop substitutes for these synthetic agro-inputs due to the evolution of pesticide resistance in some pest species and concerns about the safety of chemical residues. Currently the need is to produce maximum from the diminishing natural resources and protect the produce from post-harvest losses without adversely affecting the environment (Yadav et al., 2022). In this context, biological control through the use of natural antagonistic microorganisms has emerged as a promising alternative.
Biopesticide is a mass-produced, biologically based agent manufactured from a living microorganism or a natural product and which is sold for the control of plant pests (Organisation for Economic Co-operation and Development (OECD), 2009). Biopesticides are made from the substances that control pests by non-toxic mechanisms and have been used in different forms since human civilization. Biopesticides have more potential benefits for agriculture as well as public health programs. Biopesticides consist of various microbial pesticides, biochemicals generated from microbes and other natural sources. These are usually made by growing and concentrating naturally existing organisms and their metabolites, such as bacteria and other microorganisms, fungus, nematodes, etc. These are frequently considered vital components of IPM programs and have gained a lot of practical attention as alternatives to chemical and synthetic pesticides (Glare et al., 2012). Biopesticides could be derived from animals (e.g., nematodes), plants such as Chrysanthemum, Azadirachta (Neem), and microorganisms (e.g., Bacillus thuringiensis, Trichoderma, Pseudomonas), and include living organisms (natural enemies), their product (phytochemicals, microbial products) which can be used for the management of pest injurious (Mazidet al., 2011). The potential benefits of the utilization of biopesticides in agriculture and public health programs are considerable. The present paper provides an overview of biopesticides and their classification, formulations, application, current status and prospects.
BIOPESTICIDES
Biopesticides are pesticides derived from microorganisms or natural products that control pests through different mechanisms of action (Tijjani et al., 2016). Plants, insects and microorganisms are the primary sources of biopesticides which are cheaper, readily available, demonstrate various modes of action and are degradable. They are products or by-products derived from microorganisms (Bacillus thuringiensis, Verticillium lecanii), insects (Trichogramma spp.), animals (nematode), plant parts or extracts (Azadirachta indica) (Pavela, 2014). In the past few decades, biopesticides are the best substitute against chemical and synthetic pesticides in managing pests. They are currently used in the post- and pre-harvest control of diseases and crop pests (Yadav, 2017; Kour et al., 2020). Biopesticides are target-specific and are nontoxic to the environment and humans. The mode of action of biopesticides is specific and operates by targeting pests. Nowadays, biopesticides have played a vital role in the agro-market and are widely utilised in organic farming (Seiber et al., 2014; Nawaz et al., 2016; Lengai and Muthomi, 2018).
Biopesticides play a vital role in crop protection. They are compatible with other chemical pesticides and are also utilized in integrated crop management (ICM) practices throughout the world. Due to advancements in research and development, biopesticides have raised sustainability and reduced the pollution caused by chemical pesticides. Production of biopesticides is challenging due to the dissimilarity of the active and integrated ingredients. Moreover, when utilized as a component of IPM programs, biopesticides achieve an equivalent level of crop yield by reducing the load of chemical pesticides (Aneja et al., 2016; Satapathy, 2018; Zhang and Liu, 2022).
Biopesticides are easily available in nature, easily biodegradable, show different modes of actions, are less expensive and possess less toxicity to live organisms. Therefore, it was realized that biological control is the only means of a safe, cost-effective, and eco-friendly method to control the widespread resistance of chemical insecticides towards pests. Although the value of eco-friendly pest management in sustainable agriculture has been well recognized, only very little is being adapted at the field level. (Mishra et al., 2020). Globally, research on the application and stability of diverse biopesticides can help to assist sustainable agriculture (Kumar et al., 2019; Yadav and Yadav, 2019).
They are categorized into (a) microbial biopesticides containing microorganisms controlling diseases and insects, (b) biochemical pesticides (plant-derived), (c) plant-incorporated protectants (Kumar et al., 2019), and (d) beneficial insects (Osman et al., 2015) (Fig. 1).
Microbial pesticides
Microbial pesticides are products derived from various microorganisms (e.g. bacterium, fungus, virus, nematodes or protozoan) that are used as an active ingredient to control pests. Microbial products may consist of the organisms themselves and/or the metabolites they produce. They can control many different kinds of pests, although each separate active ingredient is relatively specific for its target pest.
Biochemical pesticides
Biochemical pesticides are naturally occurring compounds derived from various insect growth regulators, plant extracts, pheromones, oils and pyrethrins. They are characterized by a non-toxic mode of action that may affect the growth and development of a pest and its ability to reproduce. Biochemical pesticides are of following types:
Plant-Incorporated Protectants (PIPs)
Plant-Incorporated Protectants are the genes and proteins, which are introduced into plants by genetic engineering. They allow the genetically modified plant to protect itself from pests, like certain insects or viruses. These self-made pesticides are called "plant-incorporated protectants" (PIPs). PIP-producing crops are sometimes called "genetically modified" (GM) or "genetically engineered" (GE). For example, some plants produce insect-killing proteins within their tissues. They can do this because genes from Bacillus thuringiensis were inserted into the plant’s DNA.
Beneficial insects
Human cultures and civilizations have been maintained in countless ways through these beneficial insects, they regulate the pest population of many harmful pest species, produce natural products, and they also dispose the waste and recycle the organic nutrients. The generalized intensification of agriculture and the use of broad-spectrum pesticides decrease the diversity of natural enemy populations and increase the likelihood of pest outbreaks. Indeed, pesticide use has been shown to be associated with a large decrease in natural pest control services. Thus, enhancement of agro-ecosystem appears to be one of the best ways in which we can decrease the use of chemical pesticides for pest and disease control and it will increase the sustainability of crop production. These beneficial insects act by preying on pest insects or by parasitizing pest insects (parasitoids).
STATUS OF BIOPESTICIDES IN INDIA
The biocontrol concept of plant diseases has been started in India for an extended time (Schmutterer, 1985). The neem tree (Azadirachta indica A. Juss) and its derivatives, such as leaf extract, oil, and seed cake, have been used as fertilizers to minimise the risk of post-harvest loss in stored storage cereals (Brahmachari, 2004; Isman, 1997). During the 1960s, with a target of judicious use of pesticides in agriculture, the concept of IPM had also arisen (Smith and van den Bosch, 1967). However, in India, a major technological breakthrough in biocontrol occurred when conventional insecticides failed to control Helicoverpa armigera, Spodoptera litura and other cotton pests (Kranthi et al., 2002).
Around the world total biopesticides’ production is 3,000 tons per year and their use is enhancing steadily by 10% every year (Gupta and Dixit, 2010; Kumar and Singh, 2015). Marrone (2007) states that about 1,400 biopesticide products are prepared and sold worldwide. Over 200 biopesticides are sold in the US market as compared to only 60 similar products available in the European Union (EU) (EPA, 2012). In comparison, Asia lacks biopesticides and uses only 5% of biopesticides sold globally (Bailey et al., 2010; Hubbard et al., 2014).
Biopesticides are registered and regulated under the Insecticides Act, 1968. The India biopesticides market size was US$64.73 million in 2021. The market is expected to grow from US$69.62 million in 2022 to US$130.37 million by 2029, exhibiting a CAGR of 9.38% during the forecast period. Fortune Business Insights™ presents this information in its report titled “India Biopesticides Market, 2022-2029”. For sustainable farming, National Farmer Policy 2007 has promoted the utilization of biopesticides (Dar et al., 2019). In India, biopesticides have been registered under the Insecticide Act, 1968 India, which shows different biopesticides formulated in various industries (Table 1; Fig. 2). The trend of biopesticides consumption in India has shown a drastic increase in uses over time which stood at 8,647 and 8,898 tons in 2020-21 and 2021-22, respectively (Fig. 3).
In recent years, there has been a tremendous increase in the use of biopesticides, and the area of cultivation of biopesticides has been rapidly increasing. In India, West Bengal, Rajasthan, and Maharashtra used the maximum amount of biopesticides in 2021-22. Figs. 4, 5 and 6 represent the consumption and area under the use of chemical pesticides and bio-pesticides in various crops of India during 2017-18 to 2021-22. The rapid growth in the biopesticide market is based on the advantages such as inherently less harmful, reduced environmental load, affecting only one specific pest or a few pests in some cases, degradable therefore decrease exposure to the biota, thus avoiding the pollution problems, also effective in small quantities, and nontoxic to humans.
FORMULATIONS OF BIOPESTICIDES
Biopesticides are mainly plants or living organisms’ best extracts. So, during the formulation and storage process, these are to be maintained at the level of acceptance. In the process of biopesticide formulation, adjuvants and different carriers are mixed with phytochemicals to improve the storage stability, protect from the environmental conditions, and improve bioactivity. The major factors to be considered in the formulation process are: 1. stabilization and storage, 2. protection against adverse environmental conditions, 3. easy application, 4. improvement of bioactivity, and 5. maximum interaction with target pest.
Development of a bioformulation is necessary to commercialize biocontrol technologies by industries. The commercial use of bioagents requires inoculum that retains high cell viability and can easily be transported and applied to seed. It needs extensive studies for large-scale multiplication of a biocontrol agent, which include suitable and inexpensive medium, method of fermentation (solid or liquid), type of formulation (wettable powder, liquid, granular), nature of filler material, delivery systems, optimum shelf life, and storage conditions. Application guidelines are set by considering all these aspects of a bioformulation. Delivery of free cell form is usually impractical to achieve satisfactory bioremediative effect because microbes are encumbered by biotic and abiotic stresses from the environment (Ting et al., 2010). The aims of formulating viable cells are to ensure that adequate cell viability is sustained to increase the efficacy of the cells and to facilitate the delivery and handling processes (Filho et al., 2001). A bioformulation can improve product stability and shelf life and also protect microbial inoculants against different environmental conditions and provide initial food source (Jambhulkar and Sharma, 2014). Application of microbial inoculants either to increase crop health or to manage plant diseases depends on the development of commercial formulations with suitable carriers that support the survival of microorganism for considerable length of time (Aeron et al., 2011).
The formulations are broadly classified into solids and liquids; however, there are several subcategories of bioformulation that are presently accessible and utilized across India.
Solid formulation
The most prevalent type of solid bio formulations comprises of granules, micro granules, wettable powders (WPs), wettable granules (WGs), water-dispersible granules (WDGs), and dust. They are developed by mixing a binder, a dispersant, a wetting agent, etc. (Knowles, 2008). Granular formulations include dry particles with the active ingredient, carrier and binder. The concentration of active gradients in granule (GR) is 5%–20%. Apart from GR-based formulation, WPs have a prolonged shelf life and generally contain 50% – 80% technical powder, 15% – 45% filler, 1% – 10% dispersant and 3%–5% surfactant by weight (Brar et al., 2006). In contrast, dust-type formulated products have a finely ground mixture of the active ingredient (usually 10%) with particle sizes ranging from 50 to 100 µm. The other types of formulated products are the modification of the types and include WG/WDG that make WP more environmentally friendly, non-dusty and quickly soluble in water, but WG and WDG have a higher concentration of the dispersing agent. The WDG has an excellent shelf life and has been widely employed against the control of nematodes.
Liquid formulations
Liquid formulations are aqueous suspensions made in oil, water or oil and water (emulsion) (Schisler et al., 2004). A typical aqueous formulation contains 10% – 40% microorganisms, 35% – 65% carrier liquid (oil or water), 3% – 8% surfactant, 1% – 3% suspender and dispersant 1% – 5% (Brar et al., 2006). The liquid formulations are available in a variety of forms like suspension concentrates (SCs), oil miscible flowable concentrate (OF), ultralow volume (ULV) suspension (SU), and oil dispersion (OD). SCs are made by incorporating solid active ingredients among all these liquid formulations.
Liquid formulations have numerous merits like: (i) high cell count, (ii) prolonged shelf life of over a year due to the occurrence of microorganism in a dormant cyst state, which becomes active in the field after application, (iii) enhanced protection from environmental stresses and improved field efficacy, and (iv) enhancing the activity of the organism at the target site by increasing its contact with the target insect pest or pathogen (Vendan and Thangaraju, 2006).
Novel formulations
The newer formulations are a step up from traditional formulation technologies and these improved formulation types can provide products with a competitive edge, increased shelf life and better field efficacy. These sorts of formulations are often known as new-generation formulations that are environment-friendly in nature (Hazra, 2015). Some of these are illustrated below:
Encapsulation
In formulation technology, encapsulating microorganisms in a polymeric matrix is presently under experimentation. Encapsulation is creating a film or encasing microbial cells in a polymeric material to yield beads that are permeable to minerals, gases, and metabolites while permitting cellular activity to persist inside the beads (Bashan et al., 2014; John et al., 2011). Two approaches are employed depending on the mass of the beads that are produced - macro encapsulation (involving beads that are millimeters to centimeters in size) and microencapsulation (involving beads that are 1–1000 μm in size) (Nordstierna et al., 2010). Bio-encapsulation as a carrier provides a protective environment during field and storage and assists the slow release of microbes. With the usage of encapsulation, particularly suitable for soil applications, microorganisms may be prevented from biotic and abiotic stress factors like contaminants, antagonists or dryness and may proliferate at the site of application due to the beneficial microenvironment provided by the formulation (Vemmer and Patel, 2013).
Among the available polymers, alginate has been the most preferred material for most of the encapsulated formulations. According to Vassilev et al. (2005), there are at least 1,350 combinations of natural, semi-synthetic, and synthetic polymers for the entrapment of biomaterials, but the majority of techniques involved in situ entrapment by using natural polysaccharide gels which include alginates, agar, and carrageenan. The formulation provides a temporary shelter for the encapsulated strain from the soil environment and microbial competitors, both hostile to any change in the biological makeup of the soil. Any premature release of microbes from these encapsulated forms is detrimental because the main purpose of these formulations is to sustain the entrapped cells in an active phase for as long as feasible at high concentrations as nutrients and oxygen are easily accessible to a strain due to simple diffusion, the shelf life increases. The viability of microbes is higher in the case of encapsulation as controlled release to the external environment minimizes the wastage of microbial cells. Minimum storage space is required as it is immobilized in micro or macro beads.
Tablet formulation
Tablets are "preformed solids of uniform shape and dimensions, usually circular, with either flat or convex face, the distance between faces being less than the diameter". A tablet is a solid mass of uniform shape and size of an optimal blend of active ingredients (AIs) and adjuvants (Kala et al., 2020). These tablets offer numerous benefits for the industrial processes in terms of physical-chemical stability and diminished manufacturing costs, as well as beneficial to the consumer concerning easy handling and dosage precision.
The formulation is easy to handle and free from dust due to its compressed form. It offers a premeasured dose rate; thus, there is no need for weighing and making other measurements for the formulation preparation as it can be enumerated by counting the number of tablets. It is suitable in case a low dose is required (Sharma et al., 2005).
Microemulsions
Microemulsions (MEs) are homogeneous and isotropic dispersions with low viscosity, optical transparency, thermodynamic stability and an internal (dispersed) phase having typical sizes of 10-200 nm. Microemulsions are thermodynamically stable transparent dispersions of two immiscible liquids and are stable over a wide temperature range. They have a fine droplet size of fewer than 0.05 microns (50 nanometres). The total concentration of surfactants for a microemulsion can be as high as 10–30% or more, compared with about 5% for a typical o/w emulsion. Microemulsions have relatively low active ingredient concentrations, but the active ingredient's high surfactant content and solubilization may give rise to enhanced biological activity. The oil-in-water formulation was prepared by mixing the surfactant mixed oil phase with the spore suspension in the aqueous phase.
Microemulsions are easy to prepare and scalability with reduced external energy input. The shelf life of the active ingredient is also extended. There is a need to improve the solubilization of poorly water-soluble compounds as well as increase its absorption due to its smaller size.
Oil-water emulsion /oil dispersion
Oils can considerably boost the efficacy of entomopathogens against insects, hyperparasitic fungi and mycoherbicides. Over the last decade, oil dispersions (ODs) have confirmed an increasing relevance. Oil dispersions are suspensions of active ingredients in a water-insoluble solution or oil. In contrast to other liquid formulations, OD is a stable suspension of active ingredient(s) in water-immiscible solvent or oil. For the usage of fungus-containing ODs, specialized defence methods are required.
An experiment was conducted in which oil was used as a carrier to prepare liquid formulations of Trichoderma. Different oil-based formulations of Pseudomonas fluorescens were developed by employing oils like soybean oil, canola oil and groundnut oil to evaluate the viability of bacteria in the formulations (Dobhal et al., 2021). Oil-based formulations of Trichoderma viride using different oils, viz., paraffin oil, soybean oil, and mineral oil were prepared (Akshata et al., 2018). An oil-based formulation of Beauveria bassiana (Bb 112) was prepared and targeted against onion thrips tabaci (Murugasridevi et al., 2021). Several previous studies have emphasized the importance of oil in bio-preparations in contrast to water to enhance the fungal activity for insect pest infection, including the fungus B. bassiana (Prithiva et al., 2017; Sangamithra et al., 2015). The findings that fungal conidia packaged in oils have better infectivity than standard water-based suspensions have enhanced the possibility for mycopesticide development. Oil-based products are preferred for foliar spray and have improved entomopathogen efficacy. Oil evaporation is low; thus, the formulation retains its efficacy for a longer period and can be used as an emulsion. Oil traps water around the organism and slows water evaporation once applied. This is particularly beneficial for microorganisms sensitive to desiccation (Sridevi et al., 2018).
Nanoformulation
Nanotechnology has the potency to improve the delivery of microbial pesticides by utilizing nanosized preparations or formulations based on nanomaterial. Compared to conventional pesticides and associated formulations, nanomaterial-based formulations offer improved efficiency, increased solubility, induction of systemic action owing to smaller particle size and increased mobility, and reduced toxicity due to the removal of organic solvents. Pesticides developed from the nanoformulation technique are called nanopesticides. Nano-pesticides are prepared by very small particles of active pesticide ingredients or other nanostructured molecules with pesticide properties. Researchers have developed different types of nanopesticides like nanocapsule formulations, nanoemulsion, nanogel, nanospheres, and metal and metal oxide nanoparticles (Kah and Hofmann, 2014). They aid in increasing the apparent solubility of a poorly soluble active ingredient or releasing the active ingredient in a slow or targeted manner, thus protecting the active ingredient against premature degradation.
Nanoformulations may provide new strategies to improve the stability of bioagents. The controlled use of biologically active compounds (like agrochemicals) could be possible by developing a smart delivery system using biomaterials (Djiwanti and Kaushik, 2019). The potential of biodegradable and biocompatible nanomaterials like chitosan or clay as enzyme stabilizing and delivery agents is being investigated in a study. Nanoformulations stabilizing the Myrothecium enzyme complex were prepared using chitosan (250–350 nm) and montmorillonite clay nanoparticles (NPs) (100–200 nm) (Ghormade et al., 2011).
With the aid of nanotechnology, it can enhance the efficacy and accessibility of bioherbicide formulations for field application (Pallavi and Sharma, 2017). Nanoformulations are the outcome of a brand-new area of investigation; if used in weed biological control, it can lead to breakthroughs as nanoparticles with high surface areas need less bioherbicide. A nanoformulation method gaining much attention is nanoencapsulation, which is the coating of numerous components into another material at different sizes in the nano-range. Nanocapsule technology, which incorporates nanosized fungicidal or pesticide molecules, is one of the methods for developing pesticide formulations that kill pests more readily and rapidly (Guan et al., 2008).
METHODS OF APPLICATION OF BIOPESTICIDES
Biocontrol agents are delivered through several means based on the survival nature and mode of infection of the pathogen. It is generally delivered through seed treatment, root dip, soil application and irrigation water. An ideal formulation is expected to facilitate the delivery of the living biocontrol agents in its active state, at the right place, at the right time. While the formulated microbial products must be effective at the site of action and compatible with agronomic practices, they should be easy to apply to and adhere to plant parts such as seeds, tubers, cuttings, seedlings, transplants, and mature plants or be available in the soil medium. The method of application forms an important factor that decides the long term effect of any given bioagent (Hegde and Jahagirdar, 2020).
When living microorganisms are introduced, they may also augment natural beneficial populations to further reduce the damage caused by targeted pathogens (Heydari and Pessarakli, 2010). Biological control agents can be applied using different methods such as seed treatment, root inoculation, cutting and seedling root dip, soil treatment, plant inoculation, furrow application and wound application.
Seed treatment
Biological formulations applied to seeds greatly help to deliver the agents to the spermosphere of plants, where, in general, extremely conducive environments prevail. The BCAs are therefore provided an excellent opportunity to survive, multiply, persist, and exercise control of soilborne phytopathogens. Seed treatment has the potential to deliver microbial agents “in the right amount, at the right place and at the right time” (Prashant et al., 2016). At the time of planting seedlings, the formulated products can be used directly (powders, liquids) without stickers. Powders for seed treatment are formulated by mixing an active ingredient, inert carrier to facilitate product adherence to seeds by mixing seeds with formulated product Additives such as gum arabic and xanthan gum are used to prolong the survival of microbial agents applied to seeds. Alginate hydrogel, used as a seed encapsulation material, maintains the entity in a viable state and protects it from other stresses. Seed priming, in which seeds are mixed with an organic carrier and then moisture content, is brought to a level just below that required for seed treatment which has been used to deliver bioagents. In another process of seed treatment, an industrial film-coating process which was developed for the application of chemicals and biological crop protection agents is being utilized for the application of Trichoderma spp. on radish and cucumber seeds through a film coating.
Treating seeds with bacterial cultures will improve plant production and productivity by protecting plants against phythopathogens. Seed bacterization with peat-based formulation of P. fluorescens strain at the rate of 10 g/kg seed reduced rice blast and sheath blight disease (Vidhyasekaran et al., 1997). Meena et al. (2001) reported that seed treatment with powder formulation of P. fluorescens resulted in significant reduction in root rot incidence of groundnut under field conditions. Treatment of tomato seeds with powder formulation of PGPR (Bacillus subtilis, B. pumilus) reduced symptom severity of tomato mosaic virus and increased the fruit yield (Murphy et al., 2000).
Seedling root dip
This method is suitable for the vegetable crops and rice where transplanting is practiced (Singh and Zaidi, 2002). Nandakumar et al. (2001) reported that P. fluorescens strain mixtures by dipping the rice seedlings in bundles in water containing talc-based formulation of strain mixtures (20 g/l) for 2 h and later transplanting it to the main field suppressed sheath blight incidence. Application of P. fluorescens strain mixtures by dipping the seedling of rice in bucket of water containing talk based formulation containing mixture of 20 g/l for 2h and later transplanting it in the field helps to control sheath blight of rice (Nandakumar et al., 2001).
Seed bio-priming
Biopriming, a seed treatment system that integrates the biological and physiological aspects of disease control, involves coating the seed with fungal or bacterial biocontrol agents. It is a method of treating seeds with microbial inoculants and incubating under warm and moist conditions until just prior to radical emergence. Priming is one of the simple techniques which improve the vigour, seedling establishment, and plant efficiency in the field. Priming allows the early DNA transcription and RNA and protein synthase which repair the damaged parts of the seeds and reduce metabolic exudation. Biopriming may also reduce the amount of biocontrol agents that is applied to seeds (Ramanujam et al., 2010). Nayaka et al. (2008) bioprimed maize seeds with conidial suspension of Trichoderma harzianum for the control of Fusarium verticillioides and fumonisins in maize. It was found that the pure culture of T. harzianum was more effective in reducing the F. verticillioides and fumonisin incidence, followed by talc formulation.
Biopriming could also reduce the amount of biocontrol agents that is applied to the seed. Seed biopriming is successfully used in tomato, brinjal, soybean and chickpea in Tarai region of Uttaranchal (Mishra et al., 2013). Three rhizosphere competent microbial strains, viz., P. fluorescens OKC, Trichoderma asperellum T42 and Rhizobium sp. RH4, individually and in combination in bioprimed seeds of chickpea and rajma in pots and fields showed higher germination percentage and better plant growth in both the crops compared to non-bioprimed control plants. It was also observed that the combined application of the microbes enhanced seed germination and plant growth better than their individual application.
Seed encapsulation
It is a specialized seed-coating process which involves enveloping the seed, microbes, and possibly few other components such as pesticides or micronutrients, in a gelatinous or polymergel matrix, thereby prolonging the survival of microbial inoculants on seed. The gellike matrix allows the cell to remain viable with its catalytic ability for longer duration. This method of delivery system has a distinct advantage of being user friendly and environmentally safe, since the active ingredients are effectively sealed until they are released during germination. Major factors that need to be taken care of while adopting this technique are seed inoculum density, coating stability, both for microbes viability and coat integrity, in association with user feasibility and cost of production. Encapsulation of Bacillus megaterium was attempted by Sivakumar et al. (2014), with bacterial alginate by enriching the bead microenvironment with humic acid, and high viability of encapsulated bacteria with minimum cell loss after 5 months of storage was observed, thereby achieving successful plant-growth promotion of rice seedlings. This novel technique clearly demonstrates that inoculation of encapsulated microbial inoculants promotes plant growth and is feasible for application in agricultural industry.
Foliar spray application
Liquid formulations are being commonly applied on foliar parts of the plants for control of foliar pathogens. The efficacy of the foliar application mainly governs by the microclimate of the crop canopy. The crop canopy has varied concentration of nutrients like amino acids, organic acids, and sugars exuded through stomata, lenticels, hydathodes, and wounds. It affects the efficacy and survival of antagonists in phylloplane. Rice blast (Pyricularia oryzae) can be effectively controlled by foliar spray of talc-based powder formulation of P. fluorescens strain Pf1 (1 kg ha-1). The effectiveness of spraying persisted up to 2 weeks. When the bacterial product was sprayed on plants grown from treated seed, the effectiveness was higher than when spraying was carried out without any prior seed treatment (Vidhyasekaran et al., 1997). Trichoderma species can be applied as foliar spray to control diseases affecting above-ground parts. Biological control of foliar diseases is not so developed as biocontrol of soil-borne diseases. Today liquid bio formulations with high potency, cost-effective with good suspension properties, and good stability are available and being successfully adopted.
Application of Bacillus subtilis to bean leaves reduces the incidence of bean rust. Foliar application of P. fluorescent reduces the severity of bean rust and leaf spot under field conditions. Rice blast (P. oryzae) can be effectively controlled by foliar spray of talc based powder formulation of P. fluorescent strain Pf1 (1 kg/ha). The effectiveness of spraying persisted up to 15 days. When the bacterial product was sprayed on plants grown from treated seed, the effectiveness was higher than when spraying was carried out without any prior seed treatment. The dosage and frequency of application has to be standardized based on the crop value, which could be a reliable and practical approach. Selected strains from many genera of bacteria isolated from these suppressive soils have the potential to reduce plant diseases when applied to the plant root environment (Weller et al., 2002).
Soil application
Soil acts as repertoire of both beneficial and pathogenic microbes; delivering of microbial inoculants to soil will increase the population dynamics of augmented bacterial antagonists and thereby suppress the establishment of pathogenic microbes on to the infection court. Many species of Trichoderma have also been formulated extensively, using cellulosic carriers and binders and modern thin-film coating techniques, in an attempt to introduce them into the rhizosphere regions of seedlings to protect them from diseases such as Rhizoctonia solani and Pythium ultimum. Though drenching of soil with aqueous suspensions of bioagent propagules was carried out, there will not be any even distribution of bioagents in the soil. Soil drenching with T. harzianum has given good control of stem rot of groundnut caused by Sclerotium rolfsii (Kulkarni and Anahosur, 1994). An aqueous drench containing conidia of T. harzianum controlled wilt of chrysanthemum by preventing reinvasion by Fusarium oxysporum. Wilt disease of sunflower was found to be suppressed when P. cepacia strain N24 was applied to the seedbeds at the rate of 500 ml per m2 under greenhouse conditions (Hebber et al., 1991). A technique of enrichment of farmyard manure (FYM) with Trichoderma culture for soil and nursery bed application is widely accepted and appreciated by farmers for soil treatment against soil-borne pathogens. This technique involves less labour and time to multiply Trichoderma culture to manifold for soil application. Vidhyasekaran and Muthamilan (1995) stated that soil application of peat-based formulation of P. fluorescens (Pf1) at the rate of 2.5 Kg of formulation mixed with 25 Kg of well decomposed farm yard manure, in combination with seed treatment, increased rhizosphere colonization of Pf1 and suppressed chickpea wilt caused by Fusarium oxysporum f.sp. ciceris. Application of Trichoderma harzianum Th3-enriched farm yard manure in soil, along with seed treatment, before sowing of chickpea to ward off against root rot caused by Rhizoctonia solani exceptionally reduce the disease and increased yield (Jambhulkar et al., 2015).
Fluid drilling technology
Fluid drilling, also referred to as fluid sowing or gel seeding, is the technology of sowing seeds that have been germinated, using a gel to suspend and transfer them to the seedbed. This delivery system involves the incorporation of biocontrol agents into fluid drill gels. The major advantage of sowing germinated seed compared to dry seed is earlier and more uniform emergence. The gel protects the exposed radicle from mechanical damage and also provides the growing seedling with an initial water source. Unfortunately, the gel tends to attract microorganisms, including soilborne pathogens, which may result in an increased incidence of disease. Unfortunately, the gel tends to attract microorganisms, including soilborne pathogens, which may result in an increased incidence of disease (Prashant et al., 2016).
Microbigation
Applying microbial biocontrol agents to control weeds, soil pathogens, and soil insect through drip irrigation system is called “microbigation”. The conidial suspensions (106 conidia ml-1) can pass through the drippers without causing clogging, regardless of their size, and remained viable. A further advantage could be the limitation of the applied doses to the crop root zone and not the whole field, and therefore a reduction of the costs for treatment (Charudattan, 2001).
Coaggregation assay
Coaggregation is the use of multiple microbial consortia for multipronged attack against phytopathogens and to thrive together in unique ecological niches in ideal proportions, instead of using a single strain, for a single trait. It is a bacteria–bacteria, fungus–fungus, or fungus–bacteria interaction, and the interactions are highly specific that only few or certain species are consortial partners. Sivakumar and Joe (2008) attempted coaggregation of Azorhizobium caulinodans with A. brasilense, A. chroococcum, Bacillus megatherium, and Pseudomonas fluorescens to develop coaggregates with multiple benefits using seed powders of many plants, viz., Moringa oleifera, Strychnos potatorum and Sappindus emarginatus. There is wide scope of using coaggregates to deliver microbial inoculants for obtaining multiple benefits in different crops against various soil-borne pathogens. Studies on coaggregates open up the possibilities for further investigation of the genetic basis of effective coaggregation and also the nature of cellular mechanism.
Consortia application
To improve biocontrol efficacies achieved through the use of a single BCA, there has been increasing interest recently among researchers in using mixtures of BCAs to exploit potential synergistic effect among them. Biocontrol agents applied individually are not likely to perform consistently against all pathogens of the crop or under diverse crop conditions. A combination of biocontrol agents is more likely to have a greater variety of traits responsible for the suppression of one or more pathogens, and also it is likely to have these traits expressed over a wide range of environmental conditions. Accumulating evidence from literature has shown that compatible multiple strains appear to be an important prerequisite for the desired effectiveness of strains and more consistent disease suppression (Ganeshmoorthi et al., 2008). Compatible strains of P. fluorescens (Pf1, Py15 and Fp7) and Bacillus subtilis strains (EPCO 16 and EPC 5) were found to effectively inhibit the growth of Alternaria solani in tomato crop (Sundaramoorthy and Balabaskar, 2012). Similarly, experiments for the biological control of the bacterial blight pathogen revealed that different species of Bacillus applied to rice plants as a seed treatment before sowing, a root dip prior to transplantation, and two foliar sprays prior to inoculation could afford up to 59 % suppression of the disease. These treatments could also bring about a twofold increase in plant height and grain yield (Vasudevan and Gnanamanickam, 2000). Efforts are in progress, including formulation of synergy hypothesis in relation to biocontrol mechanism to exploit microbial mixture for uses in biocontrol of plant diseases.
COMMERCIALIZATION OF BIOPESTICIDES
Commercialization and growers’ acceptance of the biopesticides has been slow to develop, mainly due to the variation in efficacy under the range of the environmental conditions likely to occur in the field. This problem can only be overcome by better understanding the environmental parameters that limit biological control. In addition to this problem, there has been relatively little investment in the development commercially viable products for biological control, partly due to the cost of developing, testing efficacy, and risky, registering and marketing such a product. In recent years many small and large entrepreneurs have entered into the commercial production of biopesticides resulting into the entry of various biopesticides products into the Indian market. Commercialization of biocontrol products is a multi-step process involving a wide range of activities: (1) isolation of micro-organism from the natural ecosystem; (2) Evaluation of bio-agent both in vitro and under glass house conditions; (3) Testing of the best isolate under field conditions; (4) Mass production; (5) Formulation; (6) Delivery; (7) Compatibility; and (8) Registration and release (Prashant et al., 2016).
Most of these are sold commercially as one or more products. The technology of commercialization is still in its initial phase. Many technological problems were overcome and shifts in thinking occurred for these products to reach the shelves. Some of the commercially available biocontrol products available in the market are shown in Table 2.
Table 2: Some of the commercial formulations of biopesticides available in India (Prashant et al., 2016; Saxena et al., 2021)
Product
Bioagent(s)
Target organism
Delivery system/formulation
Developing agency
Antagon-TV
Trichoderma viride
Rhizoctonia solani and Macrophomina phaseolina
Seed treatment,
soil application
Green Tech Agro Products, Coimbatore
ARKA Krishi All Rounder and ARKA Plant Growth Booster
Pseudomonas fluorescens IIHR Pf-2 (NAIMCC-SB-0038/ITCC B0034) and Trichoderma harzianum IIHR Th-2 (NAIMCC-SF-0033/ITCC 6888)
Nematodes: Root knot nematode (Meloidogyne incognita) Bacterial pathogens–Ralstonia solanacearum and Erwinia carotovora Fungal pathogens –Fusarium oxysporum f. sp. Vasinfectum and Fusarium solani
Liquid formulation
ICAR-Indian Institute of Horticultural Research, Bengaluru
ARKA Krishi Kawach
Purpureocillium lilacinum (=Paecilomyces lilacinus) IIHR- Pl-2 (NAIMCC-SF-0034/ITCC 6887)
Root knot nematode (Meloidogyne incognita) Crops: Brinjal, tomato, carrot and okra
Wettable powder
ICAR-Indian Institute of Horticultural Research, Bengaluru
ARKA Krishi Rakshak
Pochonia chlamydosporia (=Verticillium chlamydosporium) IIHR- Vc-3(NAIMCC-SF-0035/ITCC 6898)
Root knot nematode (Meloidogyne incognita).
Crops: Brinjal, tomato, carrot and okra
Carrier based formulation;
2 × 106 cfu/g
ICAR-Indian Institute of Horticultural Research, Bengaluru
ARKA Krishi Veera
Trichoderma viride ICAR-IIHR Tv-5 (NAIMCC-SF-0032/ITCC 6889)
Root knot nematode (Meloidogyne incognita), Fungal pathogens–Fusarium oxysporum f.sp. vasinfectum, F. oxysporum f. sp. lycopersici, Sclerotium rolfsii and F. solani
Wettable powder
ICAR-Indian Institute of Horticultural Research, Bengaluru
Biocon
Pseudomonas fluorescens
Bacterial wilt and rot diseases
Spray
Tockalai Experimental Station, Tea Research Association, Jorhat, ASSAM
Bioguard
Trichoderma viride
Fusarium
Spray
Krishi Rasayan Export Pvt. Ltd. Solan (HP)
Bioshield
Pseudomonas fluorescens
Fusarium, Rhizoctonia,
Pythium, Colletotrichum and Phytophthora
Seed treatment, spray
POABS Biotech, Kuttoor, Kerala
Biotik
Metarhizium anisopliae
Termites, red ants, root
grubs, grasshoppers
Seed treatment, spray, soil application
SS Biotech Guwahati Assam
Bioderma
Trichoderma viride + Trichoderma harzianum
Pythium, Rhizoctonia,
Phytophthora and Fusarium
Seed treatment and spray
Biotech International Ltd., New Delhi, India
Bioguard Bt-L
Bacillus thuringiensis var. kurstaki DOR Bt-127 (MTCC 5976/NAIMCC-B-01463)
Spodoptera litura in soybean
Liquid suspension concentrate; 1 × 1011 cfu/mL
ICAR-Indian Institute of Oilseeds Research, Rajendranagar, Hyderabad
Bio-Pulse
Trichoderma harzianum (NAIMCC-SF-0036) and Bacillus amyloliquefaciens (NAIMCC-SB-0052
Wilt of chickpea, lentil, pea, pigeon pea; damping off/seedling mortality in papaya; Target fungi: Rhizoctonia, Sclerotium, Sclerotinia, Fusarium, Pythium, Ralstonia, Macrophomina, Bipolaris and Phoma
Talc formulation;
1 × 107 cfu/g each
ICAR-National Bureau of Agriculturally Important Microorganisms, Mau
Biowilt-X
Trichoderma harzianum
Fusarium oxysporum f.sp.
ciceris and F. udum,
Meloidogyne incognita,
and wilt disease complex (Fusarium + Meloidogyne)
Seed treatment
Dept. of Plant Pathology, AMU, Aligarh
Bionem-X
Pochonia chlamydosporia
Biocomp-X
Pseudomonas fluorescens
Ecoderma
Trichoderma viride
Fusarium, Rhizoctonia,
Pythium and Phytophthora
Seed treatment, drenching, soil application, seedling dip
Margo Biocontrol Pvt. Ltd., Bangalore
Ecofit
Trichoderma viride
R. solani and Macrophomina phaseolina
Seed treatment
Hoechast and Schering AgrEvo Ltd., Mumbai
Eco-Pesticide
Pseudomonas fluorescens
(NAIMCC-SB-0053)
Spot blotch of wheat, sheath blight of rice and wilt of tomato and chickpea
Talc based; 1 × 108 cfu/g
ICAR-National Bureau of Agriculturally Important Microorganisms, Mau
Funginil
Trichoderma harzianum
Botrytis, Pythium, Fusarium, Macrophomina,
Rhizoctonia and Sclerotinia
Seed treatment, soil application
Crop Health Bioproduct Research Centre, Gaziabad
Gliostar
Gliocladium. spp.
Fusarium, Rhizoctonia,
Sclerotium, and Pythium
Seed treatment, drenching
GBPUAT, Pantnagar
ICAR-Fusicont
Trichoderma reesei CSR-T-3 (NAIMCC-SF-0030)
Fusarium wilt of banana (Fusarium oxysporum f. sp. cubense Tropical race 4 and race 1)
Wettable powder
ICAR-Central Soil Salinity Research Institute, Karnal
Kalisena SD
Kalisena SL
Aspergillus niger AN-27
Pythium, Fusarium,
Macrophomina,
Rhizoctonia and Sclerotinia
Seed treatment, foliar spray, soil application
Cadila Pharmaceuticals
Limited, Ahmedabad
Manjari Rakshak
Trichoderma afroharzianum (NAIMCC-F-01938)
Powdery mildew of grapes
Liquid; 5 × 108 cfu/mL
ICAR-National Research Centre for Grapes, Pune
Manjari Vineguard
Trichoderma asperelloides 5R (NAIMCC-SF-0026)
Powdery mildew of grapes
Liquid formulation
ICAR-National Research Centre for Grapes, Pune
Maru sena 3
Bacillus firmus ICARCAZRI
AZ-1 (MCC 0122)
Macrophomina phaseolina in legumes and oil seed crops
Carrier based formulation; 1 × 108 cfu/g
ICAR- Central Arid Zone Research Institute, Jodhpur
Monitor
Trichoderma sp.
Fusarium, Rhizoctonia,
Sclerotium and Pythium
Seed treatment and spray
Agricultural and Biotech Pvt. Ltd. Gujarat
Mycoguard Bb-L
Beauveria bassiana (ITCC 4513)
Helicoverpa armigera in pigeon pea
Liquid suspension Concentrate
ICAR-Indian Institute of Oilseeds Research, Rajendranagar, Hyderabad
Myco-Jaal
Beauveria bassiana
Diamond black moth
Spray
Pest Control of India, Bangalore
NRRI-BBLF
Beauveria bassiana TF6
(MTCC 12233)
Rice leaf folder
(Cnaphalocrocis medinalis)
Talc formulation;
1 × 109 cfu/g
ICAR-National Rice Research Institute, Cuttack
NRRI-MALF
Metarhizium anisopliae
TF19 (MTCC 11644)
Rice leaf folder
(Cnaphalocrocis medinalis)
Talc formulation;
1 × 109 cfu/g
ICAR-National Rice Research Institute, Cuttack
Pant Biocontrol
Agent-1 (Biowilt-X)
Trichoderma harzianum
Pythium, Fusarium,
Macrophomina,
Rhizoctonia and Sclerotinia
Seed treatment,
soil application
G. B. Pant University of Agriculture Technology,
Pantnagar
Pant Biocontrol
Agent-2
Pseudomonas fluorescens
Fusarium
Seed treatment,
soil application
G. B. Pant University
of Agriculture Technology,
Pantnagar
Phule Trichokill
Trichoderma sp.
Fusarium, Rhizoctonia and Sclerotium
Seed treatment
Department of Plant
Pathology, MPKV, Rahuri
Pusa Th3
Trichoderma harzianum
Fusarium, Rhizoctonia and Sclerotium
Seed treatment,
soil application
Division of Plant Pathology, IARI, Pusa, New Delhi
Pusa 5SD
Trichoderma harzianum IARI P-4(MTCC 5371)
Fusarium oxysporum f. sp. ciceris, Sclerotium rolfsii and Sclerotinia sclerotiorum in chickpea;
Rhizoctonia solani and R. bataticola in chickpea and mungbean; Fusarium
oxysporum f. sp. lycopersici in tomato; Pythium ultimum and R. solani in French bean
Wettable powder;
108 cfu/g
Indian Council of Agricultural Sciences, New Delhi
Shatpada Aphid Kill
Beauveria bassiana ICAR-NBAIR Bb-5a (NAIMCC-F-00396)
Aphids in chilli and brinjal (Aphis gossypii); cabbage (Brevicoryne brassicae) and cowpea (Aphis craccivora)
Oil formulation
ICAR-National Bureau of Agricultural Insect Resources, Bengaluru
Shatpada All Rounder
Pseudomonas fluorescens
NBAIR-PFDWD
Thrips spp. in capsicum
And Fusarium wilt of red gram
Talc based; 1 × 108 cfu/g
ICAR-National Bureau of Agricultural Insect Resources, Bengaluru
Shatpada Armour
Bacillus thuringiensis var. kurstaki ICAR-NBAIR BT25
Armyworm (Spodoptera
frugiperda) in maize
Liquid; 1 × 108 cfu/mL
ICAR-National Bureau of Agricultural Insect Resources, Bengaluru
Shatpada Master Blaster
Bacillus albus NBAIR-BATP
Fall armyworm
(Spodoptera frugiperda) of maize, tomato pin worm (Tuta absoluta) and Fusarium wilt of cucumber (Fusarium oxysporum f. sp. cucumerinum)
Talc based; 1 × 108 cfu/g
Indian Council of Agricultural Sciences, New Delhi
Shatpada Sucking pest Hit
Lecanicillium lecanii ICAR-NBAIR Vl-8 (NAIMCC-F-01851)
Aphids in chilli (Aphis gossypii) and cowpea (Aphis craccivora)
Oil formulation
1 × 108 cfu/mL
ICAR-National Bureau of Agricultural Insect Resources, Bengaluru
Shatpada Rugose Whitefly Kill
Isaria fumosorosea ICAR-NBAIRP fu5 (NAIMCC-F-02139)
Rugose Spiralling Whitefly (Aleurodicus rugioperculatus) in coconut and oil palm
Talc; 1 × 108 cfu/g; Oil formulation; 1 × 108 cfu/mL
ICAR-National Bureau of Agricultural Insect Resources, Bengaluru
Shatpada Terminator
Bacillus thuringiensis var. kurstaki ICAR-NBAIR BTG4
Legume pod borer (Helicoverpa armigera,
Maruca sp.), diamondback moth (Plutellaxy lostella), spotted stem borer (Chilo partellus), rice leaf folder (Cnaphalocrocis medinalis), brinjal shoot borer (Leucinodes orbonalis) and redhairy caterpillar (Amsacta albistriga)
Liquid; 1 × 108 cfu/mL
ICAR-National Bureau of Agricultural Insect Resources, Bengaluru
Soil Guard
Trichoderma viride
Fusarium, Rhizoctonia,
Pythium, Colletotrichum and Phytophthora
Seed treatment,
soil application
POABS Biotech,
Kuttoor, Kerala
Sun Agro
Derma
Trichoderma viride
Fusarium, R. solani,
Macrophomina phaseolina and Colletotrichum
Seed treatment, seedling root dip, soil application
Sun Agro Chemicals,
Chennai
Sun Agro
Derma H
Trichoderma harzianum
Tricho-X
Trichoderma viride
Fusarium, Rhizoctonia,
Sclerotium, Pythium
Seed treatment, foliar spray, soil application
Excel Industries
Limited, Mumbai
Trichoderma
Trichoderma sp.
Fusarium, Rhizoctonia,
Sclerotium and Pythium
Seed treatment
Innovative Pest control Lab,
Bangalore
Trichostar
Trichoderma harzianum
Fusarium, Rhizoctonia,
Sclerotium and Pythium
Seed treatment
GBPUAT, Pantnagar
Triguard Th-L
Trichoderma harzianum Th4d (NAIMCC-F-02188)
Phytophthora seedling blight, Macrophomina root rot and Fusarium wilt of safflower and gray mold of castor, Alternaria aster leaf blight and powdery mildew of sunflower
Liquid suspension concentrates
ICAR-Indian Institute of Oilseed Research, Rajendranagar, Hyderabad
Triguard Th-P
Trichoderma harzianum Th4 (NAIMCC-F-02188)
Phytophthora seedling blight, Macrophomina root rot and Fusarium wilt of safflower and Fusarium wilt of castor and Aspergillus root rot in groundnut
Wettable powder
ICAR-Indian Institute of Oilseed Research, Rajendranagar, Hyderabad
Commercialization challenges of biopesticides in India
India is giving more emphasis on using biopesticides rather than chemical pesticides. The major factor which drives the use of biopesticides is the concern of sustainable agriculture. Various factors help in the growth of biopesticide markets like increasing adoption of organic products, concerned about the harmful effects of using synthetic pesticides, and the management of integrated pest management. In the biopesticides market, various companies are competing to hold the largest share in the market and focus on the quality of product and promotion.
Central and state agricultural universities and various ICAR institutes are major government agencies involved in commercial production of different biopesticides. Data derived from DPPQS shows that amongst the government biocontrol units, production is carried out only by a few of them. In the southern region, Tamil Nadu Agricultural University (TNAU), Coimbatore, Central Plantation Crops Research Institute (CPCRI), Indian Institute of Horticultural Research, Bangalore, Central Research Institute for Dryland Agriculture, Hyderabad, Directorate of Oilseed Research (ICAR), Hyderabad and Kerala Agricultural University (KAU), Kerala are known to have dedicated biopesticide production units. In the northeast, Assam Agriculture University and Central Agricultural University, Manipur are producing biopesticides against invasive pests. In north, Indian Agricultural Research Institute (IARI), New Delhi, Punjab Agricultural University (PAU), Punjab, G. B. Pant University of Agriculture & Technology (GBPUA & T), Uttarakhand are involved in the production of biopesticides. Whereas in Central Uttar Pradesh, Indian Institute of Sugarcane Research (IISR) and Central Institute for Subtropical Horticulture, and Directorate of Plant Protection Quarantine & Storage in Lucknow, which works under The Central Integrated Pest Management Centre are the major government agencies involved in manufacturing of biopesticides. Apart from these, with governmental support, several Krishi Vigyan Kendras (KVK) and State biocontrol labs have also been developed, which are producing biopesticides for managing the local demand. National Agricultural Cooperative Marketing Federation of India (NAFED) has also started to promote the use of biopesticides.
In India, most of the biopesticide production takes place in public sector units. It is estimated that the public sector contributes to almost 70% of the biopesticides production. Major companies like Biotech International Ltd. (New Delhi), International Panaacea Ltd., New Delhi, Ajay Biotech (India) Ltd., Pune, Bharat Biocon Pvt. Ltd. (Chhattisgarh), Microplex Biotech & Agrochem Pvt (Mumbai), Excel Crop Care Ltd., Mumbai and Govinda Agro Tech Ltd. (Nagpur), Jai Biotech Industries, Satpur, Nasik, Ganesh Biocontrol System, Rajkot, Gujarat Chemicals and Fertilizers Trading Company, Baroda, Gujarat Eco Microbial Technologies Pvt. Ltd., Vadodara, Chaitra Agri Organics, Mysore, Deep Farm Inputs (P) Ltd., Kan Biosys Pvt. Ltd., Pune Indore Biotech Inputs & Research Pvt. Ltd., Indore, Romvijay Biotech Pvt. Ltd., Pondichery Neyattinkara, Kerala, Devi Biotech (P) Ltd., Madurai, T. Stanes & Company Ltd., Coimbatore, Harit Bio Control Lab., Yavatmal and Hindustan Bioenergy Ltd., Lucknow. Some foreign companies have also entered in biopesticides production, but most of them are working in collaboration with Indian companies. For example, Lupin Agrochemicals, a Bombay based company, is now working with US-based Abbott Laboratories. Sugar and distillery companies such as KCP Sugar & Industries Corporation Ltd. (Andhra Pradesh), Rajshree Sugars & Chemicals Ltd. (Tamil Nadu), New Swadeshi Sugar Mills (Bihar), and Bannari Amman Sugars Ltd. (Tamil Nadu) are investing in developing the market of biopesticides. It indicates that the demand for biopesticides is gradually increasing (Kandar, 2021).
There are various challenges to commercialization of biopesticides in the Indian market, and the responsibility to address these challenges lies with the private sector but also with other important arms of the society like academia, state and central government agencies, public and private researchers and funding bodies, marketing professionals, etc. In fact, all the sectors involved in disease control and pest management must join hands and address these commercialization issues together (Fig. 7).
Various factors affecting the commercialization of biopesticides in the Indian agricultural market are as follows.
Awareness and information gap
The lack of awareness, knowledge, and confidence in farmers is one of the chief reasons behind the lagging of these eco-friendly pest control alternatives. Biopesticide packs carry detailed instructions and precautions for storage and usage. In spite of this, usage methods are often not clear to farmers. Sometimes the farmers lack the essential skills required for adopting the biopesticides in their agricultural farms.
Low reliability and inconsistent field performance
Lack of stability on the effect of biopesticides has been the primary concern for farmers. Since most of the microorganisms are living, several factors like temperatures, moisture, pH, exposure to ultraviolet radiation, and soil factors adversely affect their efficacy (Arora et al., 2010). Furthermore, the production of biopesticides is prone to contamination, which results in low count of active microorganisms, thus reducing the efficacy of microorganisms and leading to inconsistent field performance.
Poor quality and shelf life
Contamination and low cell count are the main concerns of the farmers and distributers (Alam, 2000). It is difficult to maintain the sterile conditions for a longer period. Contamination results in death of active microorganisms, and due to low microbial count, their performance is poorly deprived and uneven. This further leads to low shelf life and inconsistent performance of product in field conditions.
Huge investment and lack of profit
Biopesticides are high-technology products which are developed and maintained under completely sterile conditions. A huge capital investment has to be made not only in the initial development stages but also during its packaging, storage, and distribution. Screening of suitable strains and R&D issues add to the budget. Large scale screening of strains with biological activity is still required (Bashan, 1998). In general, firms with larger production facilities are expected to invest more on networks to understand and access the market. Besides, there is high risk and less profit associated with it. All these factors make biopesticide production a costly business, and companies will only develop these products if there is a long-term profit in doing so.
Health and ecological risks
Biopesticides may pose some adverse health effects if these are not used according to the guidelines mentioned on the labels of the commercial product. Biopesticides containing Bacillus thuringiensis as active ingredient are not reported to show any major adverse effects on human health, but in some cases, occupational exposure has confirmed health risks (Doekes et al., 2004). Studies on fungal biopesticides report that spore of entomopathogenic fungi such as Trichoderma, M. anisopliae, and B. bassiana may cause allergies in immune-compromised patients to farmers (Darbro and Thomas, 2009; Keswani et al., 2014).
FUTURE PROSPECTS
Despite several challenges, we believe that the microbial pesticide market in India has a bright future. Biopesticide research on the subcontinent is at a relatively early stage but evolving rapidly with increasing focus on identifying effective indigenous isolates, improving formulation and manufacturing technologies that reduce costs and enhance the shelf life of the products. Additional markets need to be explored. For example, there is little emphasis on the application of biopesticides for post-harvest use, such as grain silos and food storage systems where reside concerns and pest losses are an issue. Since rural famers in India receive little agricultural education or training, universities, federal and state agencies, and pesticide manufacturers and distributers need to work with farmers and other stakeholders to improve their knowledge and acceptance of biopesticides. Some existing initiatives may play a role in development, marketing, sale and use of microbial biopesticides. Examples include farmer field school programs, and outreach programs of pesticide suppliers who deal with farmers (input dealers). These provide opportunities to educate farmers about IPM and to differentiate between genuine and unregistered or fake biopesticides (Kaur and Kaur, 2018). In 2003, the National Institute of Agricultural Extension Management (MANAGE), an organization of the Ministry of Agriculture and Farmers Welfare, Government of India, initiated a Diploma in Agricultural Extension Services for Input Dealers (DAESI) program. The program disseminates crop production and protection technologies to farmers through input dealers, where most farmers obtain their pesticides. So far, over 4,000 candidates have been trained under this program. Demonstration of successful microbial biopesticide technologies among more progressive farmers may enhance local adoption. Consequently, most biopesticides in India are used by farmers in semi- and peri-urban areas. The current registration process for biopesticides needs to be reviewed. Current toxicological data requirements are out-dated for these products and dossier submission requirements should be streamlined for microbial biopesticides to reduce costs and accelerate the registration process. Similarly, legislation should address the patenting process for microbial products. Based on known product quality-control issues, and the proliferation of unregistered products, the effectiveness of current Central Insecticides Board and Registration Committee (CIBRC) policies to maintain the quality, accessibility and affordability of biopesticides may also require review. The adoption of future international registration standards for biopesticides may ultimately help facilitate this process (FAO and WHO, 2017).
CONCLUSION
The increasing concern of consumers on the one hand and government on the other hand about the problems associated with synthetic chemicals for pest control and on food safety has led growers to find new eco-friendly methods to replace the current chemical-based practices. The use of biopesticides as supplement has emerged as promising alternative to chemical pesticides and their demand is rising steadily in all parts of the India. Organic farming and agricultural produce free from pesticide residue would positively permit greater acceptance of biopesticides among the farmers. Biopesticide research is young and evolving and requires more attention and reliability. The challenges in broad usage of biopesticides in India are related to the efficacy, shelf-life, production methods, narrow range of host or target pathogens/pests, poor performance in the field, problems in the delivery system, economics, and regulations. In this context, discovery of new substances and research on formulation and delivery would boost commercialization and use of biopesticides. However, a commitment to the development of high quality products, a more responsive registration process, government support for research and extension and productive collaboration among researchers, industry, and farmers will help biopesticides to gain more mainstream acceptance in sustainable crop production practices.
REFERENCES
Aeron, A., R. C. Dubey, D. K. Maheshwari, P. Pandey, V. K. Bajpai, S. C. Kang. 2011. Multifarious activity of bioformulated Pseudomonas fluorescens PS1 and biocontrol of Sclerotinia sclerotiorum in Indian rapeseed (Brassica campestris L.). Eur. J. Plant Pathol. 131: 81–93.
Akshata, L. T., D. D. Guldekar, S. R. Potdukhe, S. S. Kale and A. Kumar. 2018. Shelf life study and antagonistic activity of Trichoderma viride in different oil formulations. Int. J. Curr. Microbiol. Appl. Sci. 7(11): 225–230.
Alam, G. 2000. A study of biopesticides and biofertilisers in Haryana, India.Gatekeeper series no. 93 IIED, London.http://pubs.iied.org/pdfs/6348IIED.pdf.
Aneja, K. R., S. A. Khan and A. Aneja. 2016. Biopesticides an eco-friendly pest management approach in agriculture: status and prospects. Kavaka. 47: 145–154.
Antonious, G. F. 2003.Impact of soil management and two botanical insecticides on urease and invertase activity. J. Environ. Sci. Health. 38(4): 479–488.
Arora, N. K., E. Khare and D. K. Maheshwari. 2010. Plant growth promoting rhizobacteria: constraints in bioformulation, commercialization and future strategies. In: Maheshwari, D. K. (ed) Plant growth and health promoting bacteria. Springer, Berlin, 97–116.
Bailey, K. L., S. M. Boyetchko and T. Längle. 2010. Social and economic drivers shaping the future of biological control: a Canadian perspective on the factors affecting the development and use of microbial biopesticides. Biological Control. 52(3): 221–229.
Bashan, Y. 1998. Inoculants of plant growth promoting bacteria use in agriculture. Biotech. Adv. 6: 729–770.
Bashan, Y., L. E. de-Bashan, S. R. Prabhu and J. P. Hernandez. 2014. Advances in plant growth-promoting bacterial inoculant technology: Formulations and practical perspectives (1998–2013). Plant Soil. 378(1): 1–33.
Brahmachari, G. 2004. Neem-An omnipotent. Chembiochem. 5: 408–421.
Brar, S. K., M. Verma, R. D. Tyagi and J. R. Valéro. 2006. Recent advances in downstream processing and formulations of Bacillus thuringiensis based biopesticides. Process Biochem. 41(2): 323–342.
Charudattan, R. 2001. Biological control of weeds by means of plant pathogens: significance for integrated weed management in modern agro-ecology. BioControl. 46: 229–260.
Dar, A. S., Z. H. Khan, A. A. Khan, S. B. Ahmad. 2019. Biopesticides-its prospects and limitations: An overview. Perspective in Animal Ecology and Reproduction.296-314, Astral International, New Delhi, India.
Darbro, J. M. and M. B. Thomas. 2009. Spore persistence and likelihood of aeroallergenicity of entomopathogenic fungi used for mosquito control. American J. Trop. Med. Hyg. 80: 992–997.
Djiwanti, S. R. and S. Kaushik. 2019. Nanopesticide: Future application of nanomaterials in plant protection. In: Prasad R (ed) Plant nanobionics, Nanotechnology in the Life Sciences. Springer, 255–298.
Dobhal, A., G. M. Hegde, S. Jahagirdar and K. Mirajkar. 2021. Exploring the potential of oil based formulation of Pseudomonas fluorescens against Bipolarissorokiniana causing spot blotch of wheat and its quality content. Paper presented at virtual national symposium on “Sustainable plant health management amidst covid pandemic: Challenges and strategies, ICAR-Central Plantation Crops Research Institute, Kasaragod, 1–3 December, 2021.
Doekes, G., P. Larsen, T. Sigsgaard and J. Baelum. 2004. IgE sensitization to bacterial and fungal biopesticides in a cohort of Danish greenhouse workers: the BIOGART study. American J. Ind. Med. 46: 404–407.
Environmental Protection Agency (EPA) USA. 2012. Regulating Biopesticides.
FAO and WHO. 2017. International Code of Conduct on Pesticide Management: Guidelines for the Registration of Microbial, Botanical and Semiochemical Pest Control Agents for Plant Protection and Public Health Uses. Food and Agriculture Organization of the United Nations World Health Organization, Rome, pp. 2017.
Filho, A. B., A. B. Alves, N. T. August, R. M. Pereira and L. F. A. Alves. 2001. Stability and persistence of two formulations containing Anticarsiagemmatalis Nuclear Polyhedrovirus (AgMNPV). J. Neotrop. Entomol. 30: 411–416.
Ganeshmoorthi, P., T. Anand, V. Prakasam, M. Bharani, N. Ragupathi, et al. 2008. Plant growth promoting rhizobacteria (PGPR) bioconsortia mediates induction of defense related proteins against infection of root rot pathogen in mulberry plants. J. Plant Interact. 3: 233–244.
Ghormade, V., M. V. Deshpande and K. M. Paknikar. 2011. Perspectives for nano-biotechnology enabled protection and nutrition of plants. Biotechnol. Adv. 29(6): 792–803.
Glare, T., J. Caradus, W. Gelernter, T. Jackson, N. Keyhani, J. Köhl, P. Marrone, L. Morin and A. Stewart. 2012. Have biopesticides come of age? Trends in Biotechnol. 30(5): 250–258.
Guan, G., B. Liu, Z. Wang and Z. Zhang. 2008. Imprinting of molecular recognition sites on nanostructures and its applications in chemosensors. Sensors. 8(12): 8291–8320.
Gupta, S. and A. K. Dikshit. 2010. Biopesticides: An ecofriendly approach for pest control. J. Biopest. 3: 186–188.
Hazra, D. K. 2015. Recent advancement in pesticide formulations for user and environment friendly pest management. Int. J. Res. Rev. 2: 35–40.
Hebber, P., O. Berge, T. S. Heulin and P. Singh. 1991. Bacterial antagonists of sunflower (Helianthusannuus L.) fungal pathogens. Plant Soil. 133:131–140.
Hegde, G. M. and S. Jahagirdar, 2020.Trichoderma: A potential millennium microbe for sustainable management of field and horticulture crop diseases: An overview. J. Farm Sci. 33(2): 159–172.
Heydari, A. and M. Pessarakli. 2010. A review on biology control of fungal plant pathogen using microbial antagonists. J. Biol. Sci. 10: 273–290.
Hubbard, M., R. K. Hynes, M. Erlandson, K. L. Bailey. 2014. The biochemistry behind biopesticide efficacy. Sustainable Chemical Processes. 2(1): 1–8.
Ingram, C. W., M. S. Coyne, D. W. Williams. 2005. Effects of commercial diazinon and imidacloprid on microbial urease activity in soil and sod. J. Environ. Quality. 34(5):1573–1580.
Isman, M. B. 1997. Neem and other botanical insecticides: barriers to commercialisation. Phytoparasitica. 25(4): 339-344.
Jambhulkar, P. P. and P. Sharma. 2014. Development of bioformulation and delivery system of Pseudomonas fl uorescens against bacterial leaf blight of rice (Xanthomonas oryzae pv. oryzae ). J. Environ. Biol. 35(5): 843–849.
Jambhulkar, P. P., P. Sharma and M. L. Meghwal. 2015. Additive effect of soil application with Trichoderma enriched FYM along with seed treatment and drenching with Trichoderma formulation for management of wet root rot caused by Rhizoctonia solani in chickpea. J. Pure Appl. Microbiol. 9(1): 405–412.
John, R. P., R. D. Tyagi, S. K. Brar, R. Y. Surampalli and D. Prévost. 2011. Bio-encapsulation of microbial cells for targeted agricultural delivery. Crit. Rev. Biotechnol. 31(3): 211–226.
Kah, M. and T. Hofmann. 2014. Nanopesticide research: Current trends and future priorities. Environ. Int. 63: 224–235.
Kala, S., N. Sogan and A. Agarwal, et al. 2020. Biopesticides: Formulations and delivery techniques. In: Natural remedies for pest, disease and weed control. Academic Press, Elsevier, 209–220.
Kandar, P. 2021. Phytochemicals and biopesticides: Development, current challenges and effects on human health and diseases. J. Biomed. Res. 2(1): 3–15.
Kaur, K. and P. Kaur. 2018. Agricultural extension approaches to enhance the knowledge of farmers. Int. J. Curr. Microbiol. Appl. Sci. 7: 2367–2376.
Keswani, C., H. Dilnashin, H. Birla and S. P. Singh. 2019. Regulatory barriers to Agricultural Research commercialization: A case study of biopesticides in India. Rhizosphere. 11:100155.
Keswani, C., S. Mishra, B. K. Sarma, S. P. Singh and H. B. Singh. 2014. Unraveling the efficient application of secondary metabolites of various Trichoderma. Appl Microbiol Biotechnol. 98: 533–544.
Knowles, A. 2008.Recent developments of safer formulations of agrochemicals. Environmentalist. 28: 35–44.
Kour. D., K. L. Rana, A. N. Yadav, N. Yadav, M. Kumar, V. Kumar, et al. 2020. Microbial biofertilizers: bioresources and eco- friendly technologies for agricultural and environmental sustainability. Biocatal. Agric. Biotechnol. 23: 101487. https://doi.org/10.1016/j.bcab.2019.101487.
Kranthi, K. R., D. Russell, R. Wanjari, M. Kherde, S. Munje, N. Lavhe and N. Armes. 2002. In-season changes in resistance to insecticides in Helicoverpaarmigera (Lepidoptera: Noctuidae) in India. J. Economic Entomol. 95(1): 134–142.
Kulkarni, S. A. and Anahosur, K. H. 1994.Effect of age of groundnut plant to infection of Sclerotium rolfsii Sacc.a causal agent of stem rot disease. Karnataka J. Agric. Sci. 7:367–368.
Kumar, A., A. K. Chaturvedi, K. Yadav, K. P. Arunkumar, S. K. Malyan, P. Raja and A. N. Yadav. 2019. Fungal phytoremediation of heavy metal-contaminated resources: current scenario and future prospects. In: Recent Advancement. In: White Biotechnology Through Fungi. 437-461, Springer.
Kumar, S. 2012. Biopesticides: a need for food and environmental safety. J. Biofert. Biopest. 3(4): 1–3.
Kumar, S. and A. Singh. 2015. Biopesticides: present status and the future prospects. J. Fertilizers and Pesticides, 6(2): 100–129.
Kumari, S., S. C. Kumar, M. N. Jha, R. Kant, U. Singh and P. Kumar. 2014. Microbial pesticide: A boom for sustainable agriculture. Int. J. Scientific Engg. Res. 5(6): 1394–1397.
Lengai, G. M. W. and J. W. Muthomi. 2018. Biopesticides and Their Role in Sustainable Agricultural Production. J. Biosci. Med. 6(6): 7–41.
Littlefield-Wyer, J. G., P. Brooks, M. Katouli. 2008. Application of biochemical fingerprinting and fatty acid methyl ester profiling to assess the effect of the pesticide Atradex on aquatic microbial communities. Environ. Pollution. 153(2): 393–400.
Marrone, P. G. 2007. Barriers to adoption of biological control agents and biological pesticides. CAB Reviews: Perspectives. In: Agriculture, Veterinary Science, Nutrition and Natural Resources 2(15). CABI Publishing, UK.
Mazid, S., J. C. Kalida and R. C. Rajkhowa. 2011. A review on the use of biopesticides in insect pest management. Int. J. Sci. Adv. Technol. 1(7): 169–178.
Mishra, D. S., A. Kumar, C. R. Prajapati, A. K. Singh and S. D. Sharma. 2013. Identification of compatible bacterial and fungal isolate and their effectiveness against plant disease. J. Environ. Biol. 34: 183–189.
Mishra, J., V. Dutta and N. K. Arora. 2020. Biopesticides in India: technology and sustainability linkages. Biotech. 10(5): 1–12.
Monkiedje, A. and M. Spiteller. 2002. Effects of the phenylamide fungicides, mefenoxam and metalaxyl on the microbiological properties of a sandy loam and a sandy clay soil. Biol. Fertility Soils. 35(6): 393–398
Murphy, J. F., G. W. Zehnder, D. J. Schuster, E. J. Sikora, J. E. Polston and J. W. Kloepper (2000). Plant growth-promoting rhizobacteria mediated protection in tomato against tomato mottle virus. Plant Dis. 84: 779–784.
Murugasridevi, K., S. Jeyarani, K. Ramaraju and S. M. Kumar. 2021. Oil based formulation of Beauveria bassiana (BB 112) against onion Thrips tabaci Lindeman. Indian J. Entomol. e20405. DOI:10.55446/IJE.2021.20.
Nandakumar, R., S. Babu, R. Viswanathan, J. Sheela and R. Samiyappan. 2001 Induction of systemic resistance in rice against sheath blight diseases by plant growth promoting rhizobacteria. Soil Biol. Biochem. 33: 603–612.
Nawaz, M., J. I. Mabubu and H. Hua. 2016. Current status and advancement of biopesticides: Microbial and botanical pesticides. J. Entomol. Zool. Stud. 4(2): 241–246.
Nayaka, S. R., A. C. Niranjana, S. Uday Shankar, M. S. Niranjanraj, H. S. Reddy, C. N. Prakash and Mortensen. 2008. Archives Phytopath. Plant Prot., 43(3): 264–282.
Organisation for Economic Co-operation and Development (OECD). 2009. Series on pesticides No. 448. Report of Workshop on the Regulation of Biopesticides: Registration and Communication Issues. http://www.oecd.org/dataoecd/3/Collego55/43056580.pdf.
Osman, G. 2015. Already R, Assaeedi A, Althubiani A. Bioinsecticide Bacillus thuringiensis a comprehensive review.Egyptian J. Biological Pest Control. 25: 271–288.
Pallavi, D. Chandra and A. K. Sharma. 2017. Commercial microbial products: Exploiting beneficial plant-microbe interaction. In: Singh D, Singh H, Prabha R (eds) Plant-microbe interactions in agro-ecological perspectives. Springer, Singapore. 643–699.
Pavela, R. 2014. Limitation of plant biopesticides. In: Advances in Plant Biopesticides. 347–359, Springer, New Delhi, India.
Peshin, R, R. S. Bandral, W. J. Zhang, et al. 2009. Integrated Pest Management: A Global Overview of History, Programs and Adoption. In: Integrated Pest Management: Innovation-Development Process (Vol. 1) (Peshin R, Dhawan AK, eds). 1-50, Springer, Netherlands .
Peshin, R. and W. J. Zhang. 2014. Integrated Pest Management and Pesticide Use. In: Integrated Pest Management: Pesticide Problems (Vol. 3) (eds, Pimentel D, Peshin R,). 1-46, Springer, Netherlands.
Prashant, P., Jambhulkar, P. Sharma and R. Yadav. 2016. Delivery Systems for Introduction of Microbial Inoculants in the Field, D.P. Singh et al. (eds.), Microbial Inoculants in Sustainable Agricultural Productivity, 199–218.
Prithiva, J. N., N. Ganapathy and S. Jeyarani. 2017. Efficacy of different formulations of Beauveria bassiana (Bb 112) against Bemisia tabaci on tomato. J. Entomol. Zool. Stud. 5(4): 1239–1243.
Ramanujam, B., R. D. Prasad, S. Sriram and R. Rangeswaran. 2010. Mass production, formulation, quality control and delivery of Trichoderma for plant disease management. J. Plant Protec. Sci. 2(2): 1–8.
Sangamithra, S., S. Jeyararani, K. Ramaraju. 2015. Compatibility of different oils with Beauveria bassiana, a potential entomopathogenic fungus. The Bios. 10(3): 1113–1117.
Satapathy, S. 2018. Regulatory norms and quality control of bio-pesticides in India. Int. J. Curr. Microbiol. Appl. Sci. 7(11): 3118–3122.
Saxena, A. K., Chakdar H, M. Kumar. et al. .2021. ICAR technologies: Biopesticides for eco-friendly pest management. Indian Council of Agricultural Research, New Delhi.
Schisler, D. A., P. J. Slininger, R. W. Behle and M. A. Jackson. 2004. Formulation of Bacillus sp. for biological control of plant diseases. Phytopathol. 94: 1267–1271.
Schmutterer, H. 1985. The Neem Tree Azadirachtia indica A. Juss and Other Meliaceous Plants: Sources of Unique Natural Products For Integrated Pest Management, Medicine, Industry And Other Purposes. VCH Weinheim, Germany.
Sharma, S. K., A. K. Upadhyay, M. A. Haque, et al. 2005. Village-Scale evaluation of mosquitoe nets treated with a tablet formulation of deltamethrin against malaria vector. Med. Vet. Entomol. 19(3): 286–292.
Singh, U. S. and N. W. Zaidi. 2002. Current Status of formulation and delivery of fungal and bacterial antagonists for disease management in India, In Microbial Biopesticide Formulations and Application (EdsRabindra, R. J. S. S. Hussaini and B. Ramanujam) Project Directorate of Biological Control, Bangalore pp. 168–179.
Sivakumar, P. K. and M. M. Joe. 2008. Development of coaggregated cells as bioinoculants using plant seed powders-A novel delivery system for rice grown under lowland condition. Agric. Conspec. Sci. 73(4): 253–257.
Sivakumar, P. K., R. Parthasarthi and V. P. Lakshmipriya, 2014. Encapsulation of plant growth promoting inoculant in bacterial alginate beads enriched with humic acid. Int. J. Curr. Microbiol. App. Sci. 3(6): 415–422.
Smith, R. F. and R. Van den Bosch. 1967. Integrated control. In: Pest control: Biological, Physical and Selected Chemical Methods (eds, Kilgore W. W. and R. L. Doutt). 295–340, Academic Press, New York, USA.
Sridevi, K. M., S. Jeyarani and K. Ramaraju. 2018. Evaluation of oil-based formulation of Beauveriabassiana (Bb 112) (Bals.) Vuill.and delivery methods for the management of chilli thrips, Scirtothripsdorsalis Hood. J. Biol. Control. 32(1): 62–67.
Sundaramoorthy, S. and P. Balabaskar. 2012. Consortial effect of endophytic and plant growth promoting rhizobacteria for the management of early blight of tomato Incited by Alternaria Solani . J. Plant Pathol. Microbiol. 3: 145.
Tijjani, A., K. A. Bashir, I. Mohammed, A. Muhammad, A. Gambo and H. Musa. 2016. Biopesticides for pest's control: A review. J. Biopest. Agri. 3(1): 6–13.
Ting, A. S. Y., S. W. Mah and C. S. Tee. 2010. Identification of volatile metabolites from fungal endophytes with biocontrol potential towards Fusarium oxysporum f. sp. cubense race 4. American J. Agr. Biol. Sci. 5: 177–182. 10.3844/ajabssp.2010.177.182.
Vassilev, N., I. Nikolaeva and M. Vassileva. 2005. Polymer-based preparation of soil inoculants: Applications to arbuscular mycorrhizal fungi. Rev. Environ. Sci. Biotechnol. 4: 235–243.
Vasudevan, P. and S. S. Gnanamanickam. 2000. Progress and prospects for biological suppression of rice diseases with bacterial antagonists. Biological control and plant growth promoting rhizobacteria (PGPR) for sustainable agriculture, Hyderabad, India, April 3–4.
Vemmer, M. and A. V. Patel. 2013. Review of encapsulation methods suitable for microbial biological control agents. Biol. Control. 67(3): 380–38.
Vendan, R. T. and M. Thangaraju. 2006. Development and standardization of liquid formulation for Azospiriliumbioinoculant. Indian J. Microbiol. 46(4): 379.
Vendan, S. E. 2016. Current scenario of biopesticides and eco-friendly insect pest management in India. South Indian J. Biol. Sci. 2(2): 268–271.
Vidhyasekaran, P. and Muthamilan, M. 1995. Development of formulations of Pseudomonas fluorescens for control of chickpea wilt. Plant Dis. 79: 782–786.
Vidhyasekaran, P., R. Rabindran, M. Muthamilan, K. Nayar, K. Rajappan, N. Subramanian and K. Vasumathi. 1997. Development of powder formulation of Pseudomonas fluorescens for control of rice blast. Plant Pathol. 46: 291–297.
Wang, M. C., M. Gong, H. B. Zang, X. M. Hua, J. Yao, Y. J. Pang and Y. H. Yang. 2006. Effect of methamidophos and urea application on microbial communities in soils as determined by microbial biomass and community level physiological profiles. J. Environ. Sci. Health. 41(4): 399–413.
Weller, D. M., J. M. Raaijmakers, B. B. McSpadden Gardner and L. S. Thomashow. 2002. Microbial populations responsible for specific soil suppressiveness to plant pathogens. Ann. Rev. Phytopathol. 40: 308–348.
Yadav, A. N., R. Kumar, S. Kumar, V. Kumar, T. Sugitha, B. Singh, et al. 2017. Beneficial microbiomes: biodiversity and potential biotechnological applications for sustainable agriculture and human health. J. Appl. Biol. Biotechnol. 5: 45–5.
Yadav, N. and A. N. Yadav. 2019. Biodegradation of biphenyl compounds by soil microbiomes. Biodiversity Int. J. 3: 37–40.
Yadav, R., S. Singh and A. N. Singh. 2022. Biopesticides: Current status and future prospects. Proc. Int. Acad. Ecol. Environ. Sci. 12(3): 211–233.
Zhang, W. J. 2018. Global pesticide use: Profile, trend, cost / benefit and more. Proceedings of the International Academy of Ecology and Environmental Sciences, 8(1): 1–27.
Zhang, W. J. and G. H. Liu. 2022. A Mathematical Model to Simulate the Development of Insecticide Resistance: Assessment of IPM Technologies for Reducing Insecticide Resistance. In: Advances in Integrated Pest Management Technology: Innovative and Applied Aspects (Tanda, A. S., ed). 121-143, Springer Nature, Switzerland.
Zhang, W. J., F. B. Jiang and J. F. Ou. 2011. Global pesticide consumption and pollution: with China as a focus. Proc. Int. Acad. Ecol. Environ. Sci. 1(2): 125–144.