DOI: https://doi.org/10.56669/VOZV3838
ABSTRACT
The trend of bio-pesticide consumption in India has shown a drastic increase in use over time, which stood at 8,647 and 8,898 metric tons in 2020-21 and 2021-22, respectively. Although the value of eco-friendly pest management in sustainable agriculture has been well recognized, very little is being adapted at the field level. But making it reach the field with a suitable delivery method and maintaining its consistent performance is the next most important challenge. In this review, we examined the different types of disease management practices especially organic but more importantly sustainable approaches to improve the acceptability and commercial usage for plant nutrition, crop production/ protection, plant health promotion, and their possible incorporation into the integrated pest management system.
Keywords: Fungal pathogens; Integrated Disease Management; Biocontrol; Soil Solarization
INTRODUCTION
Plant diseases are regarded as a major biotic constraint, resulting in significant crop losses worldwide. Plant pathogenic fungi stand forefront in causing devastating crop plant epidemics, as well as causing persistent and significant crop yield losses each year (El-Baky, 2021). The tormenting cases of late blight of potato caused by Phytophthora infestans in the 1980s, the Great Bengal Famine of 1943 and the southern corn leaf blight epidemic of 1970–1971 in the United States (Large, 1940; Ullstrup, 1972; Padmanabhan, 1973; Strange, 2003) emphasizes the far-reaching impacts of fungal diseases in transforming the course of social and political history. On a global scale, 16% of yield losses are caused by plant diseases, among which 70–80% of these losses are caused by fungal pathogens (Moore et al., 2020). Every year, fungal pathogens are estimated to cost the global economy hundreds of billions of dollars. Soilborne plant pathogens such as Rhizoctonia sp., Fusarium sp., Verticillium sp., Sclerotinia sp., Pythium sp., and Phytophthora sp. can cause 50–75% yield loss for many crops such as wheat, cotton, maize, vegetables, fruit, and ornamentals as reported to date (Baysal-Gurel and Kabir, 2018). In cereal crops, fungal diseases are a significant yield-limiting factor, with estimated yield losses of 15–20%; when widespread, losses can reach up to 50%. Blumeria graminis, Puccina recondita, Puccinia graminis, Puccina striiformis, Septoria tritici and Septoria nodorum, and Fusarium are all economically important cereal diseases (Rozewicz et al 2021). In legume crops, 144 fungal species are reported, among which Botrytis, Ascochyta, Stemphylium, and Septoria species are important foliar pathogens (Pande et al., 2009).
A number of methods have been used to manage phytopathogenic fungus. Among them, chemical fungicides have been used for a long time, but they have recently become the subject of serious public concern because they are harmful to our environment and non-target organisms (Zhang et al., 2007; Tozlu et al., 2018). Due to excessive and repeated application of chemical fungicides, pathogens have developed resistance. Furthermore, they have demonstrated resistance to these formulations even at higher doses, and sometimes repeated applications are required. Thus, the greatest threat to farmers now comes from these resistant pathogens (Vallieres and Avery, 2017). Hence, the development of sustainable disease management practices is required to eradicate the harmful impact of these chemicals on human health and food safety and to achieve sustainable agriculture. In this paper, we reviewed all green management approaches against plant fungal diseases.
INTEGRATED DISEASE MANAGEMENT
Soil solarization: model for sustainable crop protection
Soil solarization is one of the natural ways to manage soil-borne plant diseases, which involves the process of trapping solar energy by moist soil covered with transparent polyethylene films. This technique has been used in over 50 countries so far, mostly in hot and humid climates. It is a safe, easy-to-use, efficient, and environmentally friendly technique for fields because it doesn't emit any hazardous chemical residue into the ground (Kapoor, 2013). Because of its suitability as an model for sustainable crop protection, soil solarization may play an important role in the next years, particularly for nations such as India shown to manage diseases like damping-off, root rot, and wilt caused by various fungal pathogens in different crops, including Rhizoctonia solani, Fusarium spp., Pythium spp., Phytophthora spp., Verticillium spp., Bipolaris sorokiniana, Plasmodiophora brassicae, Sclerotium rolfsii, and others (Katan et al.,1983; Puri, 2016; Gelsomino et al., 2006; Chellemi and Mirusso, 2006).
This pre-plant method involves capturing solar radiations and heating the soil for 4-6 weeks during the summer when the soil receives the most sunlight. Soil absorbs solar energy, causing its temperature to rise to levels lethal to many soil-borne pathogens. The plant pathogen mortality is caused by elevated soil temperature (often between 45 and 55o C) at a soil depth of 5 cm beneath transparent polyethylene sheets (Katan, 1981). Apart from thermal killing of pathogens, disease suppression is also attributed to microbial, chemical and physical processes that occur in the soil both during and after the solarization treatment. Particularly, changes in microbial populations, alterations in the chemical and physical makeup and structure of the soil, high moisture levels maintained by the mulch, and alterations in the gas composition of the soil should also be taken into account (Katan, 1981; Horiuchi, 1984). A beneficial microbial shift is frequently created in solarized soil, resulting in soil suppressiveness. The performance of soil solarization can be enhanced by integrating it with additional management techniques (Katan and Gamliel, 2014).
Soil solarization also works well in conjunction with other techniques such as mulching and soil amendments to improve overall effectiveness against pests and crop yield (D'Addabbo et al., 2010). According to Dominguez et al., (2014) and Mauromicale et al., (2010), the integration of soil biofumigation with plasticulture (biosolarization) provides a sustainable solution for crop protection. Mawar and Lodha (2002) proved that integration of Indian mustard residues with single irrigation and without plasticulture resulted in a 70-80% reduction of the population of F. oxysporum f.sp. cumini due to the effect of hot summers by reaching more than 400C in the topsoil layer (Mawar and Lodha, 2002). In another experiment, Mawar and Lodha (2009) found that combining soil solarization (42-430C at 15 cm depth) with Brassica amendments reduced pathogen propagules of M. phaseolina and F. oxysporum f.sp. cumini than their alone incorporation. Besides, the study done in southern Italy is evident that the combination of soil solarization and organic amendments protects soil enzymatic properties and microbiota against the deleterious heating effects (Scopa and Dumontet, 2007).
BIOFUMIGATION: ALTERNATIVE TO CHEMICAL FUMIGATION
Biofumigation is yet another sustainable agronomic technique that exploits natural plant compounds to control soil borne plant pathogens and insect pests. Certain biofumigation approaches include cover crops, whole plant integration, and the use of separated plant products (for example, commercially produced defatted seed meal), concentrated plant essential oils, and distilled plant essences (Lazzeri et al., 2009). As an alternative to chemical soil fumigants, Brassicaceae plants are either used for biofumigantor catch crop green manures for soil disinfection and fertility management (Lazzeri et al., 2004). They are rich source of sulphur-containing secondary plant metabolites called as glucosinolates (GSLs) and hydrolyze to volatile compounds like isothiocyanates (ITCs), nitriles, epithionitriles, oxazolidines and thiocyanates catalyzed by myrosinase (MYR, β-thioglucosideglucohydrolase) isoenzymes (Abdallah et al., 2020). Particularly, ITCs are liberated from glucosinolates during tissue damage are responsible for suppression of soil borne plant pests. For instance, mustard cover crops can be grown in field prior to the crop and then tilled into the soil to achieve biofumigation supplemented with soil health benefits of cover crops (McGuire, 2003). Besides derived materials such as Defatted Seed Meal (DSM) based biofumigant pellets of different Brassica can also be used (Lazzeri et al., 2009). Major target pathogens include Fusarium, Rhizoctonia, Pythium, Phytophthora, Aphanomyces, Gaumannomyces, Sclerotinia and Verticillium and plant parasitic nematodes such as Meloidogne, Globodera, Pratylenchus and Tylenchus. Recently, laboratory and greenhouse study conducted in Egypt has used fresh and dried plants of Brassica juncea, methanol extract, or seed powder and meal of Indian mustard (Brassica juncea) as a biofumigant to suppress Rhizoctonia solani infection of common beans (Abdallah et al., 2020). Other non brasicaceous crops such as Sorghum (Dhurrin), Cassava (Linamarin), Marigold (α-terthienyl), are reported to be excellent nematotoxic biofumigants (Dutta et al., 2019). Intriguingly, another approach known as biosolarization, which involves supplementing soil with organic wastes prior to solarization, has accelerated pest and disease suppression by producing organic acids such as acetic, iso-butyric, and butyric acid (Simmons et al., 2016).
SOIL AMENDMENTS: SUSTAINABLE MICROBIAL AND NUTRIENT ENRICHMENT STRATEGY
Organic soil amendments are typically employed to enhance crop yield and soil quality, but they can also help to reduce soil borne infections. Although the application of these soil supplements is uncommon, composts and liquids enhanced with essential oils, phenols, organic acids, and many other biocidal chemicals from herbs may be beneficial against soilborne fungal diseases. To manage soil borne diseases and pests, organic manures comprised of organic wastes, composts, and peat have been suggested. The use of organic amendments is found to be an effective management method for many soil borne pathogens (Bonanomi et al., 2007). Application of heavy doses of farmyard manure or decomposed green manure at 50 kg/palm/year along with 5 kg of neem cake effectively checks the Ganoderma disease in coconut (Prakasam et al., 1997). Despite the fact that the studies have yet to confirm the effect of frequency of organic amendments to the soil on disease suppression, the recurrent addition of the organic amendments increased respiration and chemical properties of the soil (Bonanomi et al., 2018). The study conducted by El-Sharouny (2015) suggested that organic soil amendment with B. juncea seed meal could be used as biocontrol agents as they could suppress R. solani by increasing the total microbial count in soil.
A new developing technique called biochar, a pyrolyzed biomass waste, can be used to suppress plant disease by incorporating it as an organic amendment to the soil (Jaiswal et al., 2018). It is very critical to consider the concentration of biochar used. At relatively lower concentrations (<1%) enhanced plant growth performance and suppressed soil-borne diseases on the other hand, was ineffective at greater concentrations (>3%) and even exacerbated disease incidence and severity when compared to the control (Jaiswal et al., 2014). Mechanisms of biochar that could influence the development of soil-borne pathogens include: i) alterations in nutrient supplement; ii) changes in soil physiochemical characteristics; iii) activation of systemic plant defenses; iv) changes in soil microbial abundance; v) changes in pathogen growth; its survival and virulence; and vi) immobilization and deactivation of pathogenic enzymes and/or toxins (Jaiswal et al., 2018)
One of the main mechanisms that proved to be essential in biochar suppression of soilborne diseases was the population buildup of beneficial microorganisms and increased microbial activity.
IMPROVING PLANT RESISTANCE
Improving crop resistance to pathogens through plant breeding or genetic engineering is an environmentally benign method of disease management and yield loss reduction Plant breeding provides an efficient and sustainable alternate solution to chemicals that can be coupled with other management techniques in integrated methods Disease-resistant crops, for example, perform better with timely planting and harvesting, as well as crop diversification These cultivars have physical, morphological, or biochemical characteristics that reduce the plant's attractiveness or suitability for pathogen invasion, colonization, and reproduction. Under challenging scenario of fast emerging races and climate change, it is an arduous task to breed varieties with effective, stable, and broad-spectrum resistance. As the single R- genes (qualitative resistance) are mostly non-durable, they can be combined with multiple R- genes (quantitative resistance) Although the development of durable complex resistance is difficult, it is possible to assemble polygenic resistance by phenotypic and/or genotypic selection
The following approaches or methods are used for the development or improvement of varieties against plant diseases Marker-assisted breeding methods include QTL-mapping, marker-assisted selection, meta-analysis, R gene pyramiding, association mapping, genomic selection, haplotype analysis, and speed breeding. They have been used since the 1990's. Cisgenesis, intragenesis, and genome editing are examples of new breeding technologies that have been used from 2009 onwards. Next generation sequencing (NGS) based technologies like quantitative trait loci sequencing (QTL-seq), bulk segregant analysis-sequencing (BSA-seq), mutant sequencing (mut-seq), and R-gene enrichment sequencing (Ren-seq) have been used from 2013 onwards.
Marker-assisted selection (MAS) is used to implement pyramiding, which has been successful for a different crops (Wang et al., 2001, 2017; Pietrusinska et al., 2011; Bai et al., 2012; Jiang et al., 2012; Singh et al., 2017). Finding the relevant markers, validating the effect in various genetic backgrounds, and eventually deploying the relevant major genes are all necessary for successful implementation of major genes (Bernardo, 2008). Major gene implementation is further complicated when it comes to selecting multiple major genes simultaneously for gene pyramiding. A large population is needed to screen and select the lines with more than one gene in early generations while still maintaining enough lines to select for other traits in later generations (Poland and Rutkoski, 2016). Unfavorable linkage and numerous significant gene sources are additional factors contributing to the problem (Bernardo, 2008).
Genomic-assisted breeding is gaining popularity as an important tool in breeding programs due to the necessity to hasten the development of superior varieties. A new breeding method known as genomic selection or genome wide selection aims specifically at improving quantitative traits by predicting breeding values of selection candidates using genome-wide marker coverage Because genomic selection is based on genotypic data rather than phenotypic data, it reduces the frequency of phenotyping. Merrick et al., (2021) conducted a study to optimize genomic selection (GS) models for use in breeding programs in order to select both major and minor genes for resistance in wheat crop against stripe rust (Puccinia striiformis) diseases.
In view of breakdown of resistance, exploration of newer resistant genes becomes an urgent and difficult task both in practice and theory. Study of host pathogen interaction will aid in understanding pathogenesis mechanism and identifying defense related genes and their associated pathways as well as susceptibility targets. Tremendous progress achieved next-generation sequencing technologies omics tool opens avenues for exploring more R genes in a high-throughput and quantitative manner especially for non-model organisms with genomic sequences that are yet to be measured. Identified candidate genes may be exploited for genetic manipulation or gene editing or even serve as expression quantitative loci (eQTL or e-Marker) for breeding programmes (Malathi et al., 2022).
BIOCONTROL AGENTS: A GREEN TOOL IN AGRO-ENVIRONMENTAL SUSTAINABILITY
Biological control approaches to plant diseases include any reduction in the amount or their impact (disease-producing activity) that is accomplished through the introduction of biological mechanisms or the activity of naturally occurring or introduced antagonists, which occurs by modifying the microenvironment to favor the activity of antagonists (Baker, 1987; Stirling and Stirling, 1997). In general, fungi or bacteria isolated from the rhizosphere, endosphere, or phyllosphere that fight against plant-pathogenic organisms are used as biocontrol agents (BCAs) against plant diseases. The most frequently utilized bacteria for biological control and promoting plant development are Fluorescent pseudomonas, while species of Bacillus and Streptomyces have also been used commonly. The three fungi that are utilized as biocontrol agents most frequently are Trichoderma, Gliocladium and Coniothyrium (Haggag, 2002).
To achieve the best possible disease control, it is important to know the BCAs' mode of action. Biocontrol agents or microbial antagonists prevent infection of the host plant by the pathogen, or establishment of the pathogen in the host plant. The principal mechanisms for the control have been assumed to be those that act primarily upon the pathogens. The antagonists can exhibit several direct or indirect mechanisms of action involved in biological disease control. Direct interference with the pathogen is being caused by antagonists acting through hyperparasitism and antibiosis. These interactions are highly regulated metabolic cascades that often combine multiple modes of action. The compounds viz., signalling molecules, enzymes, and other interfering metabolites are produced during interaction. Without direct interaction with the targeted pathogen, some BCAs interact with plants via causing resistance or priming them (El-Baky et al., 2021).
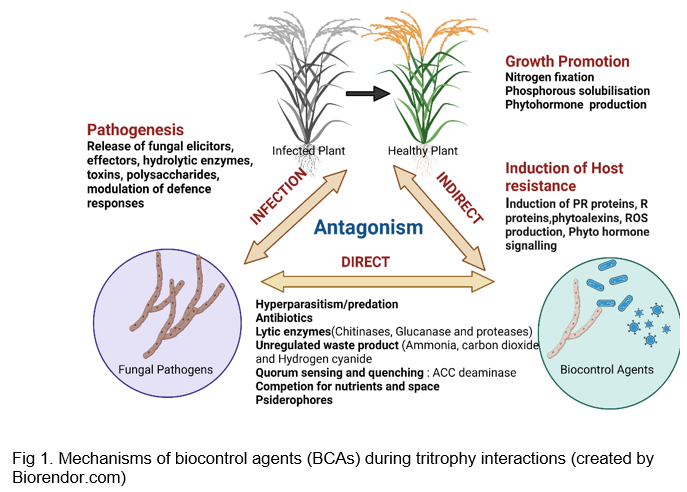
Microbial antagonists used for biocontrol of plant fungal diseases, in general, have multiple mechanisms of action. Trichoderma species, for example, act through parasitism, by production of antibiotics and enzymes that degrade the fungal cell wall, competition for nitrogen or carbon, and the production of auxin-like compounds that promote plant growth (Harman, 2006). Several microbial antagonists have been developed and commercialized for use against soil-borne phytopathogenic fungi that cause diseases in plant parts above ground. Trichoderma species, such as Trichoderma harzianum, are among the most widely used microbial antagonists for the biocontrol of plant fungal diseases caused by Fusarium, Rhizoctonia, and a variety of phytopathogenic fungi.
Formulations of microbial fungicides include liquid suspensions, granules, or dusts, which are applied in the soil just before cultivation or directly to plant roots. They can also be formulated as conventional sprays and applied on harvested fruits, plant stems, or leaves. Furthermore, novel application method, such as honeybee’s delivery during pollination, have been developed. Isolates of Trichoderma spp. can effectively control B. cinerea on strawberries, but frequent application is required. In this, bees were used in the spread of biological control agents to the target crop (Shafir et al., 2006).
By adding suppressive soil that contains antagonistic microorganisms to naturally pathogen-conducive soil, phytopathogenic fungi can be managed. Fungal pathogens and plant fungal diseases are suppressed as a result of the additional suppressive soil (Cha et al., 2016). In some cases, plant fungal disease can sometimes only be reduced by monocultures of the same crop in a pathogen-conducive soil because the antagonistic microflora to the fungal pathogen will develop over time (Nega, 2014). Monoculture of cucumber or wheat, for example, reduces Rhizoctonia infections caused by cucumber damping-off and wheat take all, respectively. Other studies contend that intercropping, or the concurrent growing of different crop species, is more effective than monoculture in preventing disease.
BOTANICALS
Secondary metabolites such as phenols, phenolic acids, quinones, flavones, flavonoids, flavonols, tannins, and coumarins can be produced by plants (Cowan, 1999). Carvacrol, eugenol, and thymol are examples of substances with phenolic compounds that are very effective against plant pathogens. These chemical compounds have antimicrobial properties and act as defence mechanisms for plants against pathogenic microbes. These substances are known as botanical pesticides or botanicals when they are taken from the plant and applied to disease-infected crops. It is highly attractive to use plant products, especially essential oils, to manage post-harvest infections (Gurjar et al., 2012).
In plant pathology research, using plant extracts and oils in their raw form to manage pathogens like fungi, bacteria, viruses, and nematodes is the most common method (Table 3). Extracts and essential oils from various plant species have shown efficacy in inhibiting plant pathogens under controlled conditions (Isman, 2000; Zaker, 2016). However, with few exceptions, the promising outcomes shown in the lab or greenhouse are typically not seen in the field (Jing et al., 2018). Under field conditions, plant bioactive compounds can degrade and volatilize quickly. Medicine, pharmaceuticals, food technology, and cosmetology use controlled-release liquid and solid formulations with plant compounds as active ingredients to avoid their degradation. However, there is less research on the application of formulations using plant compounds in agriculture (Borges et al., 2018). The following are the principal methods of encapsulation of plant active compounds:
Lyophilization (freeze-drying)
The plant product (extract or oil) is quickly frozen during the freeze-drying process, maintaining its chemical properties and shown to have fungicidal properties. The mycelial growth of the phytopathogenic fungi Fusarium solani, Pyrenochaeta lycopersici, Thielaviopsis basicola, Verticillium dahliae, and Penicilum sp. was inhibited by freeze-dried extracts of Ruta graveolens (Oliva et al., 1999). In another study, Phakopsora pachyrhizi spore germination was reduced by up to 85% by extracts from Pelargonium sp., Salvia officinalis, Lavandula officinalis, Mentha pulegium, and Mentha arvensis (Borges et al., 2013).
Emulsions
Recently emulsions of essential oils and plant extracts are used for managing plant diseases. Ribes et al. (2016) revealed that the nano encapsulated essential oils of cinnamon, lemon, and bergamot reduced the mycelial growth and spore germination of Aspergillus niger. In another study, Ali et al. (2017) showed the inhibition of Rhizoctonia solani and Sclerotium rolfsii by nano emulsions of oils of Azadirachta indica and Cymbopogon nardus.
Coacervation
The most suggested method for microencapsulating oily substances and essential oils is coacervation since it is inexpensive (Garcia-Saldana et al., 2016). Girardi et al. (2016) treated the peanut seeds with microcapsules containing essential oil of Peumusboldus, which was produced by complex coacervation. They provided the best protection for 114 days of storage against Penicillium sp. and Aspergillus sp. They suggested that complex coacervation is a suitable technique for entrapping essential oils with high encapsulation efficiency and controlled release of active compounds.
Spray drying/ Atomization
This is a low-cost industrial process, particularly for essential oil microencapsulation. Spray-drying formulations have not been extensively studied for plant pathogen control (Correa et al., 2016; Cortesi et al., 2017). Gallic acid or coffee leaf extract formulations have rarely been utilized as plant resistance inducers.
Molecular inclusion
Molecular inclusion complexes are special compounds in which the molecules of one component, known as the guest molecule, are completely or partially contained within the cavity of the other component, known as the host molecule. In recent years, against fungal pathogens, cyclodextrin molecular inclusion has been used to encapsulate active compounds. Estrada-Cano et al., (2017) found that the essential oils of clove and oregano microencapsulated with β-cyclodextrin effectively controlled Fusarium oxysporum.
GREEN NANO TECHNOLOGY: REVOLUTIONARY PLAYER IN MODERN ERA
Nanotechnology is the study of structures, technologies, and systems known as nanoparticles (NPs) with dimensions less than 100 nm and physicochemical features such as a high surface-to-volume ratio, which improves their reactivity and biological activity. It is one of the novel technologies for the management of plant diseases (Tabrez et al., 2016). Green nanotechnology does not arise de novo; rather, it is based on the concepts of green chemistry and green engineering. Here nanoparticles are synthesized using biological organisms such as bacteria, fungi, actinobacteria, algae and plant/ plant products (Azizi et al., 2013; Agarwal et al., 2017; Singh et al., 2018). The molecules like enzymes, proteins, phenolic compounds, alkaloids, amines, and pigments are used as reducing agents in nanoparticle synthesis. Therefore, the green method of nanoparticle synthesis, which offers an eco-friendly way to synthesize nanoparticles, is promoted as an alternative to chemical and physical methods (Abdul et al., 2014). Furthermore, its synthesis does not necessitate the use of costly, hazardous, and toxic chemicals. Biological techniques, which have been increased in application in recent years, can be utilized to produce metallic nanoparticles with various shapes, sizes, compositions, and physicochemical characteristics. The following are some examples of green nanotechnology nanoparticles used in fungal disease management, along with their targeted pathogens.
USE OF MYCOVIRUSES : A DOUBLE EDGED SWORD AGAINST FUNGAL PATHOGENS
Mycoviruses are viruses infecting fungi, therefore mycoviruses associated with hypovirulence may be used to control fungal diseases. However, how mycovirus strains survive in the field is unknown, and no mycovirus is used for crop protection in the field. Zhang et al., (2020) transformed Sclerotinia sclerotiorum from a pathogen to a beneficial endophytic fungus using a previously identified mycovirus. Their findings show that mycovirus reduces the expression of key pathogenicity factor genes in S. sclerotiorum during infection. They speculate that mycoviruses may have an impact on the origin of endophytism. They proposed an innovative disease control strategy that uses mycovirus-infected strains to improve crop health and release mycoviruses into the field. Mycovirus-infected strains were discovered to regulate plant gene expression responsible for defense, circadian rhythm, and hormone signaling pathways, promoting plant growth and disease resistance.
CONCLUSIONS
An extensive review of the scientific literature in this chapter reveals that soil solarization, bio fumigation, soil amendments, improving plant resistance, biocontrol agents, endophytes, green nanotechnology, and the use of mycoviruses as a safe, effective, and alternate control measure to chemical fungicides against fungal diseases of crops. Though some of these technologies, like green nanotechnology, use of endophytes, and mycoviruses, are still developing, they represent promising control means that deserve proper introduction to field applications. They can help to protect human and animal health and also the soil health by maintaining its biodiversity and thus improving the agriculture field to eliminate or reduce the damaging effects of chemical fungicides.
REFERENCES
Abdallah I, Yehia R and Kandil M A. 2020. Biofumigation potential of Indian mustard (Brassica juncea) to manage Rhizoctoniasolani. Egypt J Biol Pest Control 30: 99.
Abdul H, Sivaraj R and Venckatesh R. 2014. Green synthesis and characterization of zinc oxide nanoparticles from Ocimum basilicum L. var. purpurascens Benth.-lamiaceae leaf extract Mater. Lett, 131, 16–18.
Agarwal H, Venkat Kumar S and Rajeshkumar S. 2017. A review on green synthesis of zinc oxide nanoparticles: An eco-friendly approach. Resour.-EfficTechnol 3: 406–413.
Ali E O M, Shakil N A, Rana V S, Sarkar D J, Majumder S, Kaushik P, Singh B B and Kumar J. 2017. Antifungal activity of nano emulsions of neem and citronella oils against phytopathogenic fungi, Rhizoctoniasolani and Sclerotium rolfsii. Ind Crop Prod 108: 379-387.
Archana H R, Darshan K, Lakshmi M A, Ghoshal T, Bashayal B M and Aggarwal R. 2022. Biopesticides: A key player in agro-environmental sustainability. In Trends of Applied Microbiology for Sustainable Economy, Academic Press, 613-653pp.
Azizi S, Namvar F, Mahdavi M, Ahmad M and Mohamad R. 2013. Biosynthesis of Silver Nanoparticles Using Brown Marine Macroalga, Sargassum muticum Aqueous Extract. Materials 6: 5942–5950.
Bai B, He Z H, Asad M A, Lan C X, Zhang Y and Xia X C. 2012. Pyramiding adult-plant powdery mildew resistance QTLs in bread wheat, Crop Pasture Sci 63: 606–611.
Baker K F. 1987. Evolving concepts of biological control of plant pathogens. Annu. Rev. Phytopathol. 25(1): 67–85.
Baysal-Gurel F and Kabir N. 2018. Comparative performance of fungicides and biocontrol products in suppression of Rhizoctonia root rot in viburnum. J Plant Pathol Microbiol 9: 451.
Bernardo R. 2008. Molecular markers and selection for complex traits in plants: learning from the last 20 years. Crop Sci 48: 1649.
Bonanomi G, Antignani V, Pane C and Scala F. 2007. Suppression of soilborne fungal diseases with organicamendments. J Plant Pathol 89: 311–324.
Bonanomi G, Lorito M, Vinale F and Woo S L. 2018. Organic amendments, beneficial microbes, and soil microbiota:Toward a unified framework for disease suppression. Annu Rev Phytopathol 56: 1–20.
Borges D F, Lopes E A, Fialho Moraes A R, Soares M S, Visotto L E, Oliveira C R and Moreira Valente V M. 2018. Formulation of botanicals for the control of plant-pathogens: A review. Crop Prot 110: 135–140.
Borges D I, Alves E, Moraes M B and Oliveira D F. 2013. Efeito de extratos e óleosessenciais de plantasnagerminação de urediniósporos de Phakopsorapachyrhizi. Rev Bras Plantas Med 15: 325–331.
Cha J Y, Han S, Hong H J, Cho H, Kim D, Kwon Y, Kwon S K, Crusemann M, Lee Y B and Kim J F. 2016. Microbial and biochemical basis of a Fusarium wilt-suppressive soil. ISME J 10: 119–129.
Chellemi D O and Mirusso J. Optimizing soil disinfection producers for fresh market tomato and pepper production. Plant Dis 90: 668–674.
Correa J L G, Mendonça K S, Rodrigues L R, Resende M L V and Alves G E. 2016. Spray drying of coffee leaf extract. Coffee Sci 11: 359–367.
Cortesi R, Quattrucci A, Esposito E, Mazzaglia A and Balestra G M. 2017. Natural antimicrobials in spray-dried microparticles based on cellulose derivatives as potential eco-compatible agrochemicals. J Plant Di. Prot 124: 269–278.
Cowan M M. 1999. Plant products as antimicrobial agents. Clinical Microbiol Rev 12: 564-582.
D’Addabbo T, Miccolis V, Basile M and Candido V. 2010. Soil solarization and sustainable agriculture. In Sociology, Organic Farming, Climate Change, and Soil Science, ed. E. Lichtfocus (Dordrecht: Springer), 217–274.
Dominguez P, Miranda L, Soria C, de los Santos B, Chamorro M, Romero F, Daugovish O, Lopez-Aranda J and Medina J. 2014. Soil biosolarization for sustainable strawberry production. Agron Sustain Dev 34: 821–829.
Dutta TK, Khan MR and Phani V. 2019. Plant-parasitic nematode management via biofumigation using brassica and non-brassica plants: Current status and future prospects. Curr Plant biol 17:17-32.
El-Baky NA and Amara AAAF. 2021. Recent Approaches towards Control of Fungal Diseases in Plants: An Updated Review. J Fungi 7: 900.
El-Sharouny EE. 2015. Effect of different soil amendments on the microbial count correlated with resistance of apple plants towards pathogenic Rhizoctonia solani AG-5, Biotechnol Biotechnol Equip 29(3): 463-469.
Estrada-Cano C, Castro MAA, Munoz-Castellanos LNAOA, Garcia-Triana NAOA and Hernandez-Ochoa L. 2017. Antifungal activity of microcapsulated clove (Eugenia caryophyllata) and Mexican oregano (Lippiaberlandieri) essential oils against Fusarium oxysporum. J Microb Biochem Technol 9: 567–571.
Flood J and Hasan Y. 2004. Basal stem rot-taxonomy, biology, epidemiology, economic status and control in South East Asia and Pacific Islands. In Proceedings of the International Conference on Pest and Diseases of Importance to The Oil Palm Industry. Malaysian Palm Oil Board.
Garcia-Saldana JS, Campas-Baypoli ON, Lopez-Cervantes J, Sanchez-Machado DI, Cantu-Soto EU and Rodriguez-Ramirez R. 2016. Microencapsulation of sulforaphane from broccoli seed extracts by gelatin/gum Arabic and gelatin/pectin complexes. Food Chem 201: 94–100.
Gelsomino A, Badalucco L, Landi L and Cacco G. 2006. Soil carbon, nitrogen and phosphorus dynamics as affected by solarization alone and combined with organic amendment. Plant Soil 279: 307–325.
Girardi NS, Garcia D, Robledo SN, Passone MA, Nesci A and Etcheverry M. 2016. Microencapsulation of Peumusboldus oil by complex coacervation to provide peanut seeds protection against fungal pathogens. Ind Crop Prod 92: 93–101.
Gliessman SR. 1990. Understanding the basis of sustainability for agriculture in the tropics: Experiences in Latin America. In: C.A. Edwards, R. Lal, P. Madden, R. H. Miller and G. House (eds.), Sustainable agricultural systems. SWCS, Ankeny, USA, PP:378-390.
Gurjar M, Ali S, Akhtar M and Singh K. 2012. Efficacy of plant extracts in plant disease management. Agric Sci 3, 425-433.
Haggag WM. 2002. Sustainable Agriculture Management of Plant Diseases. J Biol Sci 2: 280-284.
Narayanasamy Harman GE. 2006. Overview of Mechanisms and Uses of Trichoderma spp”. Phytopathol, 96: 190–194.
HassanM, Zayton MA and El-Feky S A. 2019. Role of Green Synthesized Zno Nanoparticles as Antifungal against Post-Harvest Gray and Black Mold of Sweet Bell Pepper. J Biotechnol Bioeng 3(4): 8-15.
Horiuchi S. 1984. Soil solarization for suppressing soil-borne diseases in Japan, in The Ecology and Treatment of Soil-Borne diseases in Asia. Technical Bulletin No.78, (Taiwan: Food and Fertilizer Technology Center), 11–23pp.
Ilias GNM. 2009. Trichoderma and its efficacy as a bio control agents of Basal Stem Rot of oil palm (Elaeis guinensis, Jacq.), Ph.D thesis, University Putra Malaysia,
Isman MB. 2000. Plant essential oils for pest and disease management, Crop Protect 19: 603–608.
Jaiswal AK, Elad Y, Graber ER and Frenkel O. 2014. Rhizoctonia solani suppression and plant growth promotion in cucumber as affected by biochar pyrolysis temperature, feedstock and concentration. Soil Biol Biochem 69: 110–118.
Jaiswal AK, Frenkel O, Tsechansky L, Elad Y and Graber ER. 2018. Immobilization and deactivation of pathogenic enzymes and toxic metabolites by biochar: a possible mechanism involved in soilborne disease suppression. Soil Biol Biochem 121: 59–66.
Jiang M, Chunyu Z, Khalid H, Nan L, Quan S, Qing M, Suwen W and Feng L. 2012. Pyramiding Resistance Genes to Northern Leaf Blight and Head Smut in Maize. Int J Agric Biol 14: 430–434.
Jing C, Zhao J, Han X, Huang R, Cai D and Zhang C. 2018. Essential oil of Syringa oblata Lindl. as a potential biocontrol agent against tobacco brown spot caused by Alternaria alternata. Crop Protect 104:41–46.
Kapoor RT. 2013. Soil solarization: eco-friendly technology for farmers in agriculture for pest management, in Proceedings of 2nd International Conference on Advances in Biological and Pharmaceutical Sciences (ICABPS 2013), Hong Kong.
Katan J and Gamliel A. 2014. Plant Health Management: Soil Solarization”, Editor(s): Neal K. Van Alfen, Encyclopedia of Agriculture and Food Systems, Academic Press, 460-471.
Katan J, Fishler G and Grinstein A. 1983. Short and long-term effects of soil solarization and crop sequence on Fusarium wilt and yield of cotton in Israel. Phytopathol 73:1215-1219.
Katan J. 1981. Solar heating (solarization) of the soil for control of soil borne pests. Annu Rev Phytopathol 19:211–236.
Large EC. 1940. The advance of the fungi. Jonathan Cape Ltd., London.
Lazzeri L, Curto G, Dallavalle E, D’avino L, Malaguti L, Santi R and Patalano G. 2009. Nematicidal efficacy of biofumigation by defatted Brassicaceae meal for control of Meloidogyne incognita (Kofoid et White) Chitw. on a fullfield zucchini crop. J Sustain Agric 33: 349-58.
Lazzeri L, Curto G, Dallavalle E, D'Avino L, Malaguti L, Santi R and Patalano G .2009. Nematicidal Efficacy of Biofumigation by Defatted Brassicaceae Meal for Control of Meloidogyne incognita (KofoidetWhite) Chitw. on a Full Field Zucchini Crop. J Sustain Agric 33(3): 349–358.
Lazzeri L, Leoni O and Manici LM. 2004. Biocidal plant dried pellets for biofumigation Indus. Crops Prod 20: 59- 65.
Malathi VM, Lakshmi MA and Charles S. 2022. The Applications of Genomics and Transcriptomics Approaches for Biotic Stress Tolerance in Crops”. In Principles and Practices of OMICS and Genome Editing for Crop Improvement. Springer Cham 93-122.
Marcianò D, Mizzotti C, Maddalena G and Toffolatti S. 2021. The Dark Side of Fungi: How They Cause Diseases in Plants. Front Young Minds 9:560315.
Mauromicale G, Lo Monaco A and Longo AMG. 2010. Improved efficiencyof soil solarization for growth and yield of greenhouse tomatoes. Agron Sustain Dev 30:753–761.
Mawar R and Lodha S. 2002. Brassica amendments and summer irrigation for the control of Macrophomina phaseolina and Fusarium oxysporum f.sp. cumini in hot arid regions. Phytopathol Mediterr 41:45–54.
Mawar R and Lodha S. 2009. Prior weakening of Macrophomina phaseolina and Fusarium propagules for enhancing efficiency of Brassica amendments. Crop Protect 28:812–817.
McGuire A. 2003. Mustard — Cover Crops for the Columbia Basin. Bulletin EB1952E. Grant-Adams: Washington State University Cooperative Extension.
Merrick LF and Carter AH. 2017. Comparison of genomic selection models for exploring predictive ability of complex traits in breeding programs. bioRxiv 15:440015.
Merrick LF, Burke AB, Chen X and Carter AH. 2021. Breeding With Major and Minor Genes: Genomic Selection for Quantitative Disease Resistance. Front Plant Sci 12: 713667.
Mihajlovic M, Rekanovic E, Hrustic J and Tanovic B. 2017. Methods for management of soilborne plant pathogens, Pestic Fitomedicina 32: 9–24.
Moore D, Robson GD and Trinci APJ. 2020. 21st Century Guidebook to Fungi – Second Edition - Reviews and Contents, Cambridge University Press, 143.
Mores A, Borrelli GM, Laido G, Petruzzino G, Pecchioni N, Amoroso LGM, Desiderio F, Mazzucotelli E, Mastrangelo AM and Marone D. 2021. Genomic Approaches to Identify Molecular Bases of Crop Resistance to Diseases and to Develop Future Breeding Strategies. Int J Mol Sci 22(11):5423.
Mundt CC, Cowger CC and Garrett KA. 2002. Relevance of integrated disease management to resistance durability.Euphytica 124: 245–252.
Mundt CC. 2015. Durable resistance: a key to sustainable management of pathogens and pests. Genet Evol 27: 446–455.
Nega A. 2014. Review on concepts in biological control of plant pathogens. J Biol Agric Health 4: 33–35.
Nelson R, Wiesner-Hanks T, Wisser R and Balint-Kurti P. 2018. Navigating complexity to breed disease-resistant crops. Nature Rev Genet 19(1):21-33.
Oerke EC and Dehne HW. 2004. Safeguarding production losses in major crops and the role of crop protection. Crop Prot 23: 275–285.
Oliva A, Lahoz E, Contillo R and Aliott G. 1999. Fungistatic activity of Rutagraveolens extract and its allelochemicals. J Chem Ecol 25: 519–526.
Padmanabhan SY. 1973 The great Bengal famine. Ann Rev Phytopathol 11(1): 11-24.
Pal KK and McSpadden GB. 2006. Biological Control of Plant Pathogens. The Plant Health Instructor.
Palloix A, Ayme V and Moury B. 2009. Durability of plant major resistance genes to pathogens depends on the genetic background, experimental evidence and consequences for breeding strategies. New Phytol 183:190–199.
Pande SM, Sharmal S, Kumari PM, Gaur W, Chen L, Kaur W, MacLeod A, Basandrai, D, Basandrai A, Bakr JS, Sandhu HS. Tripathi and Gowda CLL. 2009. Integrated foliar diseases management of legumes”, International Conference on Grain Legumes: Quality Improvement, Value Addition and Trade, February 14-16, Indian Society of Pulses Research and Development, Indian Institute of Pulses Research, Kanpur, India, 143-161.
Pietrusinska A, Czembor JH and Czembor PC. 2011. Pyramiding two genes for leaf rust and powdery mildew resistance in common wheat. Cereal Res Commun 39:577–588.
Poland J and Rutkoski J. 2016. Advances and challenges in genomic selection for disease resistance. Annu Rev Phytopathol 54:79–98.
Prakasam V, Valluvaparidasan V, Raguchander T, Prabakar K, Thiruvudainambi S. 1997. Field crop disease. Coimbatore (India): A. E. Publications 111–112.
Puri S. 2016. Eco-friendly management strategies for soil borne plant pathogens. Int J Adv Res Biol Sci 3(1):69-75.
Ribes S, Fuentes A, Talens P, Barat JM, Ferrari G and Donsi F. 2016. Influence of emulsifier type on the antifungal activity of cinnamon leaf, lemon and bergamot oil nanoemulsions against Aspergillus Niger. Food Contr 73: 784–795.
Rozewicz M, Wyzinska M and Grabinski J. 2021.The Most Important Fungal Diseases of Cereals— Problems and Possible Solutions, Agronomy 11:714.
Rutkoski JE, Heffner EL and Sorrells ME. 2011. Genomic selection for durable stem rust resistance in wheat. Euphytica, 179:161–173.
Santillan Martínez MI, Bracuto V, Koseoglou E, Appiano M, Jacobsen E, Visser RG, F, Wolters AMA and Bai Y. 2020. CRISPR/Cas9-targeted mutagenesis of the tomato susceptibility gene PMR4 for resistance against powdery mildew. BMC Plant Biol 20:284.
Scopa A and Dumontet S. 2007. Soil solarization: effects on soil microbiological parameters J. Plant Nutr 30:537–547.
Shafir S, Dag A, Bilu A, Abu-Toamy M and Elad Y. 2006. Honey bee dispersal of the biocontrol agent Trichoderma harzianum T39: effectiveness in suppressing Botrytis cinerea on strawberry under field conditions. Eur J Plant Pathol 116:119–128.
Simmons CW, Higgins B. Staley, S, Joh LD, Simmons BA, Singer SW, Stapleton JJ. and Vander Gheynst JS. 2016. The role of organic matter amendment level on soil heating, organic acid accumulation, and development of bacterial communities in solarized soil. Appl Soil Ecol 106:37-46.
Singh J, Dutta T, Kim KH, Rawat M, Samddar P and Kumar P. 2018. Green synthesis of metals and their oxide nanoparticles: Applications for environmental remediation. J Nanobiotechnol 16:84.
Singh M, Mallick N, Chand S, Kumari P, Sharma JB, Sivasamy M, Jayaprakash P, Prabhu KV and Jha SK. 2017.Marker-assisted pyramiding of Thinopyrum-derived leaf rust resistance genes Lr19 and Lr24 in bread wheat variety HD2733. J genet 96(6):951-7.
Stirling M and Stirling G. 1997. Disease Management: Biological Control in Plant Pathogens and Plant Diseases. Eds. J. Brown and H. Ogle, 427–439.
Strange RN. 2003. Introduction to Plant Pathology. Chichester, UK: Wiley. 464.
Sundram S, Meon S, Seman IA and Othman R. 2011. Symbiotic interaction of endophytic bacteria with arbuscular mycorrhizal fungi and its antagonistic effect on Ganoderma boninense. J Microbiol 49(4):551-557.
Susanto A, Sudharto PS and Purba RY. 2005. Enhancing biological control of basal stem rot disease (Ganoderma boninense) in oil palm plantations. Mycopathologia, 159(1):153-157.
Tabrez S, Musarrat J, Al-khedhairy AA. 2016. Colloids and surfaces B: biointerfaces countering drug resistance, infectious diseases, and sepsis using metal and metal oxides nanoparticles: current status, Colloids Surf. B Biointerfaces 146:70–83.
Tozlu E, Tekiner N, Kotan R and Serkan O. 2018. Investigation on the biological control of Alternaria alternata. Indian J Agric Sci 88: 1241–1247.
Ullstrup AJ. 1972. The impacts of the southern corn leaf blight epidemics of 1970-1971. Ann review Phytopathol 10(1): 37-50.
Vallieres C and Avery S V. 2017. Metal-based combinations that target protein synthesis by fungi. Adv Microb Physiol 70: 105–121.
Vanblaere T, Flachowsky H, Gessler C and Broggini GA. 2014. Molecular Characterization of Cisgenic Lines of Apple ‘Gala’ Carrying the Rvi6 Scab Resistance Gene. Plant Biotechnol J 12:2–9.
Wang D, Lin Z, Kai LI, Ying MA, Wang L, Yang YQ, Yang YH and Zhi HJ. 2017 Marker-assisted pyramiding of soybean resistance genes RSC4, RSC8, and RSC14Q to soybean mosaic virus. J Integr Agric 16: 2413–2420.
Wu J, Zeng Q, Wang Q, Liu S, Yu S, Mu J, Huang S, Sela H, Distelfeld A, Huang L and Han D. 2018. SNP-based pool genotyping and haplotype analysis accelerate fine-mapping of the wheat genomic region containing stripe rust resistance gene Yr26. Theor Appl Genet 131(7):1481-96.
Zaker M. 2016. Natural plant products as eco-friendly fungicides for plant diseases control -a review. Agric For 14:134–141.
Zhang H, Xie J, Fu Y, Cheng J, Qu Z, Zhao Z, Cheng S, Chen T, Li B, Wang Q and Liu X. 2020. A 2-kb Mycovirus Converts a Pathogenic Fungus into a Beneficial Endophyte for Brassica Protection and Yield Enhancement. Mol Plant 13: 1420–1433.
Zhang J. 2007. The potential of a new fungicide fludioxonil for stem-end rot and green mold control on Florida citrus fruit. Postharvest Biol Technol 46: 262–270.
Green Management of Pathogens for Plant Health Improvement by Utilizing Sustainable Practices
DOI: https://doi.org/10.56669/VOZV3838
ABSTRACT
The trend of bio-pesticide consumption in India has shown a drastic increase in use over time, which stood at 8,647 and 8,898 metric tons in 2020-21 and 2021-22, respectively. Although the value of eco-friendly pest management in sustainable agriculture has been well recognized, very little is being adapted at the field level. But making it reach the field with a suitable delivery method and maintaining its consistent performance is the next most important challenge. In this review, we examined the different types of disease management practices especially organic but more importantly sustainable approaches to improve the acceptability and commercial usage for plant nutrition, crop production/ protection, plant health promotion, and their possible incorporation into the integrated pest management system.
Keywords: Fungal pathogens; Integrated Disease Management; Biocontrol; Soil Solarization
INTRODUCTION
Plant diseases are regarded as a major biotic constraint, resulting in significant crop losses worldwide. Plant pathogenic fungi stand forefront in causing devastating crop plant epidemics, as well as causing persistent and significant crop yield losses each year (El-Baky, 2021). The tormenting cases of late blight of potato caused by Phytophthora infestans in the 1980s, the Great Bengal Famine of 1943 and the southern corn leaf blight epidemic of 1970–1971 in the United States (Large, 1940; Ullstrup, 1972; Padmanabhan, 1973; Strange, 2003) emphasizes the far-reaching impacts of fungal diseases in transforming the course of social and political history. On a global scale, 16% of yield losses are caused by plant diseases, among which 70–80% of these losses are caused by fungal pathogens (Moore et al., 2020). Every year, fungal pathogens are estimated to cost the global economy hundreds of billions of dollars. Soilborne plant pathogens such as Rhizoctonia sp., Fusarium sp., Verticillium sp., Sclerotinia sp., Pythium sp., and Phytophthora sp. can cause 50–75% yield loss for many crops such as wheat, cotton, maize, vegetables, fruit, and ornamentals as reported to date (Baysal-Gurel and Kabir, 2018). In cereal crops, fungal diseases are a significant yield-limiting factor, with estimated yield losses of 15–20%; when widespread, losses can reach up to 50%. Blumeria graminis, Puccina recondita, Puccinia graminis, Puccina striiformis, Septoria tritici and Septoria nodorum, and Fusarium are all economically important cereal diseases (Rozewicz et al 2021). In legume crops, 144 fungal species are reported, among which Botrytis, Ascochyta, Stemphylium, and Septoria species are important foliar pathogens (Pande et al., 2009).
A number of methods have been used to manage phytopathogenic fungus. Among them, chemical fungicides have been used for a long time, but they have recently become the subject of serious public concern because they are harmful to our environment and non-target organisms (Zhang et al., 2007; Tozlu et al., 2018). Due to excessive and repeated application of chemical fungicides, pathogens have developed resistance. Furthermore, they have demonstrated resistance to these formulations even at higher doses, and sometimes repeated applications are required. Thus, the greatest threat to farmers now comes from these resistant pathogens (Vallieres and Avery, 2017). Hence, the development of sustainable disease management practices is required to eradicate the harmful impact of these chemicals on human health and food safety and to achieve sustainable agriculture. In this paper, we reviewed all green management approaches against plant fungal diseases.
INTEGRATED DISEASE MANAGEMENT
Soil solarization: model for sustainable crop protection
Soil solarization is one of the natural ways to manage soil-borne plant diseases, which involves the process of trapping solar energy by moist soil covered with transparent polyethylene films. This technique has been used in over 50 countries so far, mostly in hot and humid climates. It is a safe, easy-to-use, efficient, and environmentally friendly technique for fields because it doesn't emit any hazardous chemical residue into the ground (Kapoor, 2013). Because of its suitability as an model for sustainable crop protection, soil solarization may play an important role in the next years, particularly for nations such as India shown to manage diseases like damping-off, root rot, and wilt caused by various fungal pathogens in different crops, including Rhizoctonia solani, Fusarium spp., Pythium spp., Phytophthora spp., Verticillium spp., Bipolaris sorokiniana, Plasmodiophora brassicae, Sclerotium rolfsii, and others (Katan et al.,1983; Puri, 2016; Gelsomino et al., 2006; Chellemi and Mirusso, 2006).
This pre-plant method involves capturing solar radiations and heating the soil for 4-6 weeks during the summer when the soil receives the most sunlight. Soil absorbs solar energy, causing its temperature to rise to levels lethal to many soil-borne pathogens. The plant pathogen mortality is caused by elevated soil temperature (often between 45 and 55o C) at a soil depth of 5 cm beneath transparent polyethylene sheets (Katan, 1981). Apart from thermal killing of pathogens, disease suppression is also attributed to microbial, chemical and physical processes that occur in the soil both during and after the solarization treatment. Particularly, changes in microbial populations, alterations in the chemical and physical makeup and structure of the soil, high moisture levels maintained by the mulch, and alterations in the gas composition of the soil should also be taken into account (Katan, 1981; Horiuchi, 1984). A beneficial microbial shift is frequently created in solarized soil, resulting in soil suppressiveness. The performance of soil solarization can be enhanced by integrating it with additional management techniques (Katan and Gamliel, 2014).
Soil solarization also works well in conjunction with other techniques such as mulching and soil amendments to improve overall effectiveness against pests and crop yield (D'Addabbo et al., 2010). According to Dominguez et al., (2014) and Mauromicale et al., (2010), the integration of soil biofumigation with plasticulture (biosolarization) provides a sustainable solution for crop protection. Mawar and Lodha (2002) proved that integration of Indian mustard residues with single irrigation and without plasticulture resulted in a 70-80% reduction of the population of F. oxysporum f.sp. cumini due to the effect of hot summers by reaching more than 400C in the topsoil layer (Mawar and Lodha, 2002). In another experiment, Mawar and Lodha (2009) found that combining soil solarization (42-430C at 15 cm depth) with Brassica amendments reduced pathogen propagules of M. phaseolina and F. oxysporum f.sp. cumini than their alone incorporation. Besides, the study done in southern Italy is evident that the combination of soil solarization and organic amendments protects soil enzymatic properties and microbiota against the deleterious heating effects (Scopa and Dumontet, 2007).
BIOFUMIGATION: ALTERNATIVE TO CHEMICAL FUMIGATION
Biofumigation is yet another sustainable agronomic technique that exploits natural plant compounds to control soil borne plant pathogens and insect pests. Certain biofumigation approaches include cover crops, whole plant integration, and the use of separated plant products (for example, commercially produced defatted seed meal), concentrated plant essential oils, and distilled plant essences (Lazzeri et al., 2009). As an alternative to chemical soil fumigants, Brassicaceae plants are either used for biofumigantor catch crop green manures for soil disinfection and fertility management (Lazzeri et al., 2004). They are rich source of sulphur-containing secondary plant metabolites called as glucosinolates (GSLs) and hydrolyze to volatile compounds like isothiocyanates (ITCs), nitriles, epithionitriles, oxazolidines and thiocyanates catalyzed by myrosinase (MYR, β-thioglucosideglucohydrolase) isoenzymes (Abdallah et al., 2020). Particularly, ITCs are liberated from glucosinolates during tissue damage are responsible for suppression of soil borne plant pests. For instance, mustard cover crops can be grown in field prior to the crop and then tilled into the soil to achieve biofumigation supplemented with soil health benefits of cover crops (McGuire, 2003). Besides derived materials such as Defatted Seed Meal (DSM) based biofumigant pellets of different Brassica can also be used (Lazzeri et al., 2009). Major target pathogens include Fusarium, Rhizoctonia, Pythium, Phytophthora, Aphanomyces, Gaumannomyces, Sclerotinia and Verticillium and plant parasitic nematodes such as Meloidogne, Globodera, Pratylenchus and Tylenchus. Recently, laboratory and greenhouse study conducted in Egypt has used fresh and dried plants of Brassica juncea, methanol extract, or seed powder and meal of Indian mustard (Brassica juncea) as a biofumigant to suppress Rhizoctonia solani infection of common beans (Abdallah et al., 2020). Other non brasicaceous crops such as Sorghum (Dhurrin), Cassava (Linamarin), Marigold (α-terthienyl), are reported to be excellent nematotoxic biofumigants (Dutta et al., 2019). Intriguingly, another approach known as biosolarization, which involves supplementing soil with organic wastes prior to solarization, has accelerated pest and disease suppression by producing organic acids such as acetic, iso-butyric, and butyric acid (Simmons et al., 2016).
SOIL AMENDMENTS: SUSTAINABLE MICROBIAL AND NUTRIENT ENRICHMENT STRATEGY
Organic soil amendments are typically employed to enhance crop yield and soil quality, but they can also help to reduce soil borne infections. Although the application of these soil supplements is uncommon, composts and liquids enhanced with essential oils, phenols, organic acids, and many other biocidal chemicals from herbs may be beneficial against soilborne fungal diseases. To manage soil borne diseases and pests, organic manures comprised of organic wastes, composts, and peat have been suggested. The use of organic amendments is found to be an effective management method for many soil borne pathogens (Bonanomi et al., 2007). Application of heavy doses of farmyard manure or decomposed green manure at 50 kg/palm/year along with 5 kg of neem cake effectively checks the Ganoderma disease in coconut (Prakasam et al., 1997). Despite the fact that the studies have yet to confirm the effect of frequency of organic amendments to the soil on disease suppression, the recurrent addition of the organic amendments increased respiration and chemical properties of the soil (Bonanomi et al., 2018). The study conducted by El-Sharouny (2015) suggested that organic soil amendment with B. juncea seed meal could be used as biocontrol agents as they could suppress R. solani by increasing the total microbial count in soil.
A new developing technique called biochar, a pyrolyzed biomass waste, can be used to suppress plant disease by incorporating it as an organic amendment to the soil (Jaiswal et al., 2018). It is very critical to consider the concentration of biochar used. At relatively lower concentrations (<1%) enhanced plant growth performance and suppressed soil-borne diseases on the other hand, was ineffective at greater concentrations (>3%) and even exacerbated disease incidence and severity when compared to the control (Jaiswal et al., 2014). Mechanisms of biochar that could influence the development of soil-borne pathogens include: i) alterations in nutrient supplement; ii) changes in soil physiochemical characteristics; iii) activation of systemic plant defenses; iv) changes in soil microbial abundance; v) changes in pathogen growth; its survival and virulence; and vi) immobilization and deactivation of pathogenic enzymes and/or toxins (Jaiswal et al., 2018)
One of the main mechanisms that proved to be essential in biochar suppression of soilborne diseases was the population buildup of beneficial microorganisms and increased microbial activity.
IMPROVING PLANT RESISTANCE
Improving crop resistance to pathogens through plant breeding or genetic engineering is an environmentally benign method of disease management and yield loss reduction Plant breeding provides an efficient and sustainable alternate solution to chemicals that can be coupled with other management techniques in integrated methods Disease-resistant crops, for example, perform better with timely planting and harvesting, as well as crop diversification These cultivars have physical, morphological, or biochemical characteristics that reduce the plant's attractiveness or suitability for pathogen invasion, colonization, and reproduction. Under challenging scenario of fast emerging races and climate change, it is an arduous task to breed varieties with effective, stable, and broad-spectrum resistance. As the single R- genes (qualitative resistance) are mostly non-durable, they can be combined with multiple R- genes (quantitative resistance) Although the development of durable complex resistance is difficult, it is possible to assemble polygenic resistance by phenotypic and/or genotypic selection
The following approaches or methods are used for the development or improvement of varieties against plant diseases Marker-assisted breeding methods include QTL-mapping, marker-assisted selection, meta-analysis, R gene pyramiding, association mapping, genomic selection, haplotype analysis, and speed breeding. They have been used since the 1990's. Cisgenesis, intragenesis, and genome editing are examples of new breeding technologies that have been used from 2009 onwards. Next generation sequencing (NGS) based technologies like quantitative trait loci sequencing (QTL-seq), bulk segregant analysis-sequencing (BSA-seq), mutant sequencing (mut-seq), and R-gene enrichment sequencing (Ren-seq) have been used from 2013 onwards.
Marker-assisted selection (MAS) is used to implement pyramiding, which has been successful for a different crops (Wang et al., 2001, 2017; Pietrusinska et al., 2011; Bai et al., 2012; Jiang et al., 2012; Singh et al., 2017). Finding the relevant markers, validating the effect in various genetic backgrounds, and eventually deploying the relevant major genes are all necessary for successful implementation of major genes (Bernardo, 2008). Major gene implementation is further complicated when it comes to selecting multiple major genes simultaneously for gene pyramiding. A large population is needed to screen and select the lines with more than one gene in early generations while still maintaining enough lines to select for other traits in later generations (Poland and Rutkoski, 2016). Unfavorable linkage and numerous significant gene sources are additional factors contributing to the problem (Bernardo, 2008).
Genomic-assisted breeding is gaining popularity as an important tool in breeding programs due to the necessity to hasten the development of superior varieties. A new breeding method known as genomic selection or genome wide selection aims specifically at improving quantitative traits by predicting breeding values of selection candidates using genome-wide marker coverage Because genomic selection is based on genotypic data rather than phenotypic data, it reduces the frequency of phenotyping. Merrick et al., (2021) conducted a study to optimize genomic selection (GS) models for use in breeding programs in order to select both major and minor genes for resistance in wheat crop against stripe rust (Puccinia striiformis) diseases.
In view of breakdown of resistance, exploration of newer resistant genes becomes an urgent and difficult task both in practice and theory. Study of host pathogen interaction will aid in understanding pathogenesis mechanism and identifying defense related genes and their associated pathways as well as susceptibility targets. Tremendous progress achieved next-generation sequencing technologies omics tool opens avenues for exploring more R genes in a high-throughput and quantitative manner especially for non-model organisms with genomic sequences that are yet to be measured. Identified candidate genes may be exploited for genetic manipulation or gene editing or even serve as expression quantitative loci (eQTL or e-Marker) for breeding programmes (Malathi et al., 2022).
BIOCONTROL AGENTS: A GREEN TOOL IN AGRO-ENVIRONMENTAL SUSTAINABILITY
Biological control approaches to plant diseases include any reduction in the amount or their impact (disease-producing activity) that is accomplished through the introduction of biological mechanisms or the activity of naturally occurring or introduced antagonists, which occurs by modifying the microenvironment to favor the activity of antagonists (Baker, 1987; Stirling and Stirling, 1997). In general, fungi or bacteria isolated from the rhizosphere, endosphere, or phyllosphere that fight against plant-pathogenic organisms are used as biocontrol agents (BCAs) against plant diseases. The most frequently utilized bacteria for biological control and promoting plant development are Fluorescent pseudomonas, while species of Bacillus and Streptomyces have also been used commonly. The three fungi that are utilized as biocontrol agents most frequently are Trichoderma, Gliocladium and Coniothyrium (Haggag, 2002).
To achieve the best possible disease control, it is important to know the BCAs' mode of action. Biocontrol agents or microbial antagonists prevent infection of the host plant by the pathogen, or establishment of the pathogen in the host plant. The principal mechanisms for the control have been assumed to be those that act primarily upon the pathogens. The antagonists can exhibit several direct or indirect mechanisms of action involved in biological disease control. Direct interference with the pathogen is being caused by antagonists acting through hyperparasitism and antibiosis. These interactions are highly regulated metabolic cascades that often combine multiple modes of action. The compounds viz., signalling molecules, enzymes, and other interfering metabolites are produced during interaction. Without direct interaction with the targeted pathogen, some BCAs interact with plants via causing resistance or priming them (El-Baky et al., 2021).
Microbial antagonists used for biocontrol of plant fungal diseases, in general, have multiple mechanisms of action. Trichoderma species, for example, act through parasitism, by production of antibiotics and enzymes that degrade the fungal cell wall, competition for nitrogen or carbon, and the production of auxin-like compounds that promote plant growth (Harman, 2006). Several microbial antagonists have been developed and commercialized for use against soil-borne phytopathogenic fungi that cause diseases in plant parts above ground. Trichoderma species, such as Trichoderma harzianum, are among the most widely used microbial antagonists for the biocontrol of plant fungal diseases caused by Fusarium, Rhizoctonia, and a variety of phytopathogenic fungi.
Formulations of microbial fungicides include liquid suspensions, granules, or dusts, which are applied in the soil just before cultivation or directly to plant roots. They can also be formulated as conventional sprays and applied on harvested fruits, plant stems, or leaves. Furthermore, novel application method, such as honeybee’s delivery during pollination, have been developed. Isolates of Trichoderma spp. can effectively control B. cinerea on strawberries, but frequent application is required. In this, bees were used in the spread of biological control agents to the target crop (Shafir et al., 2006).
By adding suppressive soil that contains antagonistic microorganisms to naturally pathogen-conducive soil, phytopathogenic fungi can be managed. Fungal pathogens and plant fungal diseases are suppressed as a result of the additional suppressive soil (Cha et al., 2016). In some cases, plant fungal disease can sometimes only be reduced by monocultures of the same crop in a pathogen-conducive soil because the antagonistic microflora to the fungal pathogen will develop over time (Nega, 2014). Monoculture of cucumber or wheat, for example, reduces Rhizoctonia infections caused by cucumber damping-off and wheat take all, respectively. Other studies contend that intercropping, or the concurrent growing of different crop species, is more effective than monoculture in preventing disease.
BOTANICALS
Secondary metabolites such as phenols, phenolic acids, quinones, flavones, flavonoids, flavonols, tannins, and coumarins can be produced by plants (Cowan, 1999). Carvacrol, eugenol, and thymol are examples of substances with phenolic compounds that are very effective against plant pathogens. These chemical compounds have antimicrobial properties and act as defence mechanisms for plants against pathogenic microbes. These substances are known as botanical pesticides or botanicals when they are taken from the plant and applied to disease-infected crops. It is highly attractive to use plant products, especially essential oils, to manage post-harvest infections (Gurjar et al., 2012).
In plant pathology research, using plant extracts and oils in their raw form to manage pathogens like fungi, bacteria, viruses, and nematodes is the most common method (Table 3). Extracts and essential oils from various plant species have shown efficacy in inhibiting plant pathogens under controlled conditions (Isman, 2000; Zaker, 2016). However, with few exceptions, the promising outcomes shown in the lab or greenhouse are typically not seen in the field (Jing et al., 2018). Under field conditions, plant bioactive compounds can degrade and volatilize quickly. Medicine, pharmaceuticals, food technology, and cosmetology use controlled-release liquid and solid formulations with plant compounds as active ingredients to avoid their degradation. However, there is less research on the application of formulations using plant compounds in agriculture (Borges et al., 2018). The following are the principal methods of encapsulation of plant active compounds:
Lyophilization (freeze-drying)
The plant product (extract or oil) is quickly frozen during the freeze-drying process, maintaining its chemical properties and shown to have fungicidal properties. The mycelial growth of the phytopathogenic fungi Fusarium solani, Pyrenochaeta lycopersici, Thielaviopsis basicola, Verticillium dahliae, and Penicilum sp. was inhibited by freeze-dried extracts of Ruta graveolens (Oliva et al., 1999). In another study, Phakopsora pachyrhizi spore germination was reduced by up to 85% by extracts from Pelargonium sp., Salvia officinalis, Lavandula officinalis, Mentha pulegium, and Mentha arvensis (Borges et al., 2013).
Emulsions
Recently emulsions of essential oils and plant extracts are used for managing plant diseases. Ribes et al. (2016) revealed that the nano encapsulated essential oils of cinnamon, lemon, and bergamot reduced the mycelial growth and spore germination of Aspergillus niger. In another study, Ali et al. (2017) showed the inhibition of Rhizoctonia solani and Sclerotium rolfsii by nano emulsions of oils of Azadirachta indica and Cymbopogon nardus.
Coacervation
The most suggested method for microencapsulating oily substances and essential oils is coacervation since it is inexpensive (Garcia-Saldana et al., 2016). Girardi et al. (2016) treated the peanut seeds with microcapsules containing essential oil of Peumusboldus, which was produced by complex coacervation. They provided the best protection for 114 days of storage against Penicillium sp. and Aspergillus sp. They suggested that complex coacervation is a suitable technique for entrapping essential oils with high encapsulation efficiency and controlled release of active compounds.
Spray drying/ Atomization
This is a low-cost industrial process, particularly for essential oil microencapsulation. Spray-drying formulations have not been extensively studied for plant pathogen control (Correa et al., 2016; Cortesi et al., 2017). Gallic acid or coffee leaf extract formulations have rarely been utilized as plant resistance inducers.
Molecular inclusion
Molecular inclusion complexes are special compounds in which the molecules of one component, known as the guest molecule, are completely or partially contained within the cavity of the other component, known as the host molecule. In recent years, against fungal pathogens, cyclodextrin molecular inclusion has been used to encapsulate active compounds. Estrada-Cano et al., (2017) found that the essential oils of clove and oregano microencapsulated with β-cyclodextrin effectively controlled Fusarium oxysporum.
GREEN NANO TECHNOLOGY: REVOLUTIONARY PLAYER IN MODERN ERA
Nanotechnology is the study of structures, technologies, and systems known as nanoparticles (NPs) with dimensions less than 100 nm and physicochemical features such as a high surface-to-volume ratio, which improves their reactivity and biological activity. It is one of the novel technologies for the management of plant diseases (Tabrez et al., 2016). Green nanotechnology does not arise de novo; rather, it is based on the concepts of green chemistry and green engineering. Here nanoparticles are synthesized using biological organisms such as bacteria, fungi, actinobacteria, algae and plant/ plant products (Azizi et al., 2013; Agarwal et al., 2017; Singh et al., 2018). The molecules like enzymes, proteins, phenolic compounds, alkaloids, amines, and pigments are used as reducing agents in nanoparticle synthesis. Therefore, the green method of nanoparticle synthesis, which offers an eco-friendly way to synthesize nanoparticles, is promoted as an alternative to chemical and physical methods (Abdul et al., 2014). Furthermore, its synthesis does not necessitate the use of costly, hazardous, and toxic chemicals. Biological techniques, which have been increased in application in recent years, can be utilized to produce metallic nanoparticles with various shapes, sizes, compositions, and physicochemical characteristics. The following are some examples of green nanotechnology nanoparticles used in fungal disease management, along with their targeted pathogens.
USE OF MYCOVIRUSES : A DOUBLE EDGED SWORD AGAINST FUNGAL PATHOGENS
Mycoviruses are viruses infecting fungi, therefore mycoviruses associated with hypovirulence may be used to control fungal diseases. However, how mycovirus strains survive in the field is unknown, and no mycovirus is used for crop protection in the field. Zhang et al., (2020) transformed Sclerotinia sclerotiorum from a pathogen to a beneficial endophytic fungus using a previously identified mycovirus. Their findings show that mycovirus reduces the expression of key pathogenicity factor genes in S. sclerotiorum during infection. They speculate that mycoviruses may have an impact on the origin of endophytism. They proposed an innovative disease control strategy that uses mycovirus-infected strains to improve crop health and release mycoviruses into the field. Mycovirus-infected strains were discovered to regulate plant gene expression responsible for defense, circadian rhythm, and hormone signaling pathways, promoting plant growth and disease resistance.
CONCLUSIONS
An extensive review of the scientific literature in this chapter reveals that soil solarization, bio fumigation, soil amendments, improving plant resistance, biocontrol agents, endophytes, green nanotechnology, and the use of mycoviruses as a safe, effective, and alternate control measure to chemical fungicides against fungal diseases of crops. Though some of these technologies, like green nanotechnology, use of endophytes, and mycoviruses, are still developing, they represent promising control means that deserve proper introduction to field applications. They can help to protect human and animal health and also the soil health by maintaining its biodiversity and thus improving the agriculture field to eliminate or reduce the damaging effects of chemical fungicides.
REFERENCES
Abdallah I, Yehia R and Kandil M A. 2020. Biofumigation potential of Indian mustard (Brassica juncea) to manage Rhizoctoniasolani. Egypt J Biol Pest Control 30: 99.
Abdul H, Sivaraj R and Venckatesh R. 2014. Green synthesis and characterization of zinc oxide nanoparticles from Ocimum basilicum L. var. purpurascens Benth.-lamiaceae leaf extract Mater. Lett, 131, 16–18.
Agarwal H, Venkat Kumar S and Rajeshkumar S. 2017. A review on green synthesis of zinc oxide nanoparticles: An eco-friendly approach. Resour.-EfficTechnol 3: 406–413.
Ali E O M, Shakil N A, Rana V S, Sarkar D J, Majumder S, Kaushik P, Singh B B and Kumar J. 2017. Antifungal activity of nano emulsions of neem and citronella oils against phytopathogenic fungi, Rhizoctoniasolani and Sclerotium rolfsii. Ind Crop Prod 108: 379-387.
Archana H R, Darshan K, Lakshmi M A, Ghoshal T, Bashayal B M and Aggarwal R. 2022. Biopesticides: A key player in agro-environmental sustainability. In Trends of Applied Microbiology for Sustainable Economy, Academic Press, 613-653pp.
Azizi S, Namvar F, Mahdavi M, Ahmad M and Mohamad R. 2013. Biosynthesis of Silver Nanoparticles Using Brown Marine Macroalga, Sargassum muticum Aqueous Extract. Materials 6: 5942–5950.
Bai B, He Z H, Asad M A, Lan C X, Zhang Y and Xia X C. 2012. Pyramiding adult-plant powdery mildew resistance QTLs in bread wheat, Crop Pasture Sci 63: 606–611.
Baker K F. 1987. Evolving concepts of biological control of plant pathogens. Annu. Rev. Phytopathol. 25(1): 67–85.
Baysal-Gurel F and Kabir N. 2018. Comparative performance of fungicides and biocontrol products in suppression of Rhizoctonia root rot in viburnum. J Plant Pathol Microbiol 9: 451.
Bernardo R. 2008. Molecular markers and selection for complex traits in plants: learning from the last 20 years. Crop Sci 48: 1649.
Bonanomi G, Antignani V, Pane C and Scala F. 2007. Suppression of soilborne fungal diseases with organicamendments. J Plant Pathol 89: 311–324.
Bonanomi G, Lorito M, Vinale F and Woo S L. 2018. Organic amendments, beneficial microbes, and soil microbiota:Toward a unified framework for disease suppression. Annu Rev Phytopathol 56: 1–20.
Borges D F, Lopes E A, Fialho Moraes A R, Soares M S, Visotto L E, Oliveira C R and Moreira Valente V M. 2018. Formulation of botanicals for the control of plant-pathogens: A review. Crop Prot 110: 135–140.
Borges D I, Alves E, Moraes M B and Oliveira D F. 2013. Efeito de extratos e óleosessenciais de plantasnagerminação de urediniósporos de Phakopsorapachyrhizi. Rev Bras Plantas Med 15: 325–331.
Cha J Y, Han S, Hong H J, Cho H, Kim D, Kwon Y, Kwon S K, Crusemann M, Lee Y B and Kim J F. 2016. Microbial and biochemical basis of a Fusarium wilt-suppressive soil. ISME J 10: 119–129.
Chellemi D O and Mirusso J. Optimizing soil disinfection producers for fresh market tomato and pepper production. Plant Dis 90: 668–674.
Correa J L G, Mendonça K S, Rodrigues L R, Resende M L V and Alves G E. 2016. Spray drying of coffee leaf extract. Coffee Sci 11: 359–367.
Cortesi R, Quattrucci A, Esposito E, Mazzaglia A and Balestra G M. 2017. Natural antimicrobials in spray-dried microparticles based on cellulose derivatives as potential eco-compatible agrochemicals. J Plant Di. Prot 124: 269–278.
Cowan M M. 1999. Plant products as antimicrobial agents. Clinical Microbiol Rev 12: 564-582.
D’Addabbo T, Miccolis V, Basile M and Candido V. 2010. Soil solarization and sustainable agriculture. In Sociology, Organic Farming, Climate Change, and Soil Science, ed. E. Lichtfocus (Dordrecht: Springer), 217–274.
Dominguez P, Miranda L, Soria C, de los Santos B, Chamorro M, Romero F, Daugovish O, Lopez-Aranda J and Medina J. 2014. Soil biosolarization for sustainable strawberry production. Agron Sustain Dev 34: 821–829.
Dutta TK, Khan MR and Phani V. 2019. Plant-parasitic nematode management via biofumigation using brassica and non-brassica plants: Current status and future prospects. Curr Plant biol 17:17-32.
El-Baky NA and Amara AAAF. 2021. Recent Approaches towards Control of Fungal Diseases in Plants: An Updated Review. J Fungi 7: 900.
El-Sharouny EE. 2015. Effect of different soil amendments on the microbial count correlated with resistance of apple plants towards pathogenic Rhizoctonia solani AG-5, Biotechnol Biotechnol Equip 29(3): 463-469.
Estrada-Cano C, Castro MAA, Munoz-Castellanos LNAOA, Garcia-Triana NAOA and Hernandez-Ochoa L. 2017. Antifungal activity of microcapsulated clove (Eugenia caryophyllata) and Mexican oregano (Lippiaberlandieri) essential oils against Fusarium oxysporum. J Microb Biochem Technol 9: 567–571.
Flood J and Hasan Y. 2004. Basal stem rot-taxonomy, biology, epidemiology, economic status and control in South East Asia and Pacific Islands. In Proceedings of the International Conference on Pest and Diseases of Importance to The Oil Palm Industry. Malaysian Palm Oil Board.
Garcia-Saldana JS, Campas-Baypoli ON, Lopez-Cervantes J, Sanchez-Machado DI, Cantu-Soto EU and Rodriguez-Ramirez R. 2016. Microencapsulation of sulforaphane from broccoli seed extracts by gelatin/gum Arabic and gelatin/pectin complexes. Food Chem 201: 94–100.
Gelsomino A, Badalucco L, Landi L and Cacco G. 2006. Soil carbon, nitrogen and phosphorus dynamics as affected by solarization alone and combined with organic amendment. Plant Soil 279: 307–325.
Girardi NS, Garcia D, Robledo SN, Passone MA, Nesci A and Etcheverry M. 2016. Microencapsulation of Peumusboldus oil by complex coacervation to provide peanut seeds protection against fungal pathogens. Ind Crop Prod 92: 93–101.
Gliessman SR. 1990. Understanding the basis of sustainability for agriculture in the tropics: Experiences in Latin America. In: C.A. Edwards, R. Lal, P. Madden, R. H. Miller and G. House (eds.), Sustainable agricultural systems. SWCS, Ankeny, USA, PP:378-390.
Gurjar M, Ali S, Akhtar M and Singh K. 2012. Efficacy of plant extracts in plant disease management. Agric Sci 3, 425-433.
Haggag WM. 2002. Sustainable Agriculture Management of Plant Diseases. J Biol Sci 2: 280-284.
Narayanasamy Harman GE. 2006. Overview of Mechanisms and Uses of Trichoderma spp”. Phytopathol, 96: 190–194.
HassanM, Zayton MA and El-Feky S A. 2019. Role of Green Synthesized Zno Nanoparticles as Antifungal against Post-Harvest Gray and Black Mold of Sweet Bell Pepper. J Biotechnol Bioeng 3(4): 8-15.
Horiuchi S. 1984. Soil solarization for suppressing soil-borne diseases in Japan, in The Ecology and Treatment of Soil-Borne diseases in Asia. Technical Bulletin No.78, (Taiwan: Food and Fertilizer Technology Center), 11–23pp.
Ilias GNM. 2009. Trichoderma and its efficacy as a bio control agents of Basal Stem Rot of oil palm (Elaeis guinensis, Jacq.), Ph.D thesis, University Putra Malaysia,
Isman MB. 2000. Plant essential oils for pest and disease management, Crop Protect 19: 603–608.
Jaiswal AK, Elad Y, Graber ER and Frenkel O. 2014. Rhizoctonia solani suppression and plant growth promotion in cucumber as affected by biochar pyrolysis temperature, feedstock and concentration. Soil Biol Biochem 69: 110–118.
Jaiswal AK, Frenkel O, Tsechansky L, Elad Y and Graber ER. 2018. Immobilization and deactivation of pathogenic enzymes and toxic metabolites by biochar: a possible mechanism involved in soilborne disease suppression. Soil Biol Biochem 121: 59–66.
Jiang M, Chunyu Z, Khalid H, Nan L, Quan S, Qing M, Suwen W and Feng L. 2012. Pyramiding Resistance Genes to Northern Leaf Blight and Head Smut in Maize. Int J Agric Biol 14: 430–434.
Jing C, Zhao J, Han X, Huang R, Cai D and Zhang C. 2018. Essential oil of Syringa oblata Lindl. as a potential biocontrol agent against tobacco brown spot caused by Alternaria alternata. Crop Protect 104:41–46.
Kapoor RT. 2013. Soil solarization: eco-friendly technology for farmers in agriculture for pest management, in Proceedings of 2nd International Conference on Advances in Biological and Pharmaceutical Sciences (ICABPS 2013), Hong Kong.
Katan J and Gamliel A. 2014. Plant Health Management: Soil Solarization”, Editor(s): Neal K. Van Alfen, Encyclopedia of Agriculture and Food Systems, Academic Press, 460-471.
Katan J, Fishler G and Grinstein A. 1983. Short and long-term effects of soil solarization and crop sequence on Fusarium wilt and yield of cotton in Israel. Phytopathol 73:1215-1219.
Katan J. 1981. Solar heating (solarization) of the soil for control of soil borne pests. Annu Rev Phytopathol 19:211–236.
Large EC. 1940. The advance of the fungi. Jonathan Cape Ltd., London.
Lazzeri L, Curto G, Dallavalle E, D’avino L, Malaguti L, Santi R and Patalano G. 2009. Nematicidal efficacy of biofumigation by defatted Brassicaceae meal for control of Meloidogyne incognita (Kofoid et White) Chitw. on a fullfield zucchini crop. J Sustain Agric 33: 349-58.
Lazzeri L, Curto G, Dallavalle E, D'Avino L, Malaguti L, Santi R and Patalano G .2009. Nematicidal Efficacy of Biofumigation by Defatted Brassicaceae Meal for Control of Meloidogyne incognita (KofoidetWhite) Chitw. on a Full Field Zucchini Crop. J Sustain Agric 33(3): 349–358.
Lazzeri L, Leoni O and Manici LM. 2004. Biocidal plant dried pellets for biofumigation Indus. Crops Prod 20: 59- 65.
Malathi VM, Lakshmi MA and Charles S. 2022. The Applications of Genomics and Transcriptomics Approaches for Biotic Stress Tolerance in Crops”. In Principles and Practices of OMICS and Genome Editing for Crop Improvement. Springer Cham 93-122.
Marcianò D, Mizzotti C, Maddalena G and Toffolatti S. 2021. The Dark Side of Fungi: How They Cause Diseases in Plants. Front Young Minds 9:560315.
Mauromicale G, Lo Monaco A and Longo AMG. 2010. Improved efficiencyof soil solarization for growth and yield of greenhouse tomatoes. Agron Sustain Dev 30:753–761.
Mawar R and Lodha S. 2002. Brassica amendments and summer irrigation for the control of Macrophomina phaseolina and Fusarium oxysporum f.sp. cumini in hot arid regions. Phytopathol Mediterr 41:45–54.
Mawar R and Lodha S. 2009. Prior weakening of Macrophomina phaseolina and Fusarium propagules for enhancing efficiency of Brassica amendments. Crop Protect 28:812–817.
McGuire A. 2003. Mustard — Cover Crops for the Columbia Basin. Bulletin EB1952E. Grant-Adams: Washington State University Cooperative Extension.
Merrick LF and Carter AH. 2017. Comparison of genomic selection models for exploring predictive ability of complex traits in breeding programs. bioRxiv 15:440015.
Merrick LF, Burke AB, Chen X and Carter AH. 2021. Breeding With Major and Minor Genes: Genomic Selection for Quantitative Disease Resistance. Front Plant Sci 12: 713667.
Mihajlovic M, Rekanovic E, Hrustic J and Tanovic B. 2017. Methods for management of soilborne plant pathogens, Pestic Fitomedicina 32: 9–24.
Moore D, Robson GD and Trinci APJ. 2020. 21st Century Guidebook to Fungi – Second Edition - Reviews and Contents, Cambridge University Press, 143.
Mores A, Borrelli GM, Laido G, Petruzzino G, Pecchioni N, Amoroso LGM, Desiderio F, Mazzucotelli E, Mastrangelo AM and Marone D. 2021. Genomic Approaches to Identify Molecular Bases of Crop Resistance to Diseases and to Develop Future Breeding Strategies. Int J Mol Sci 22(11):5423.
Mundt CC, Cowger CC and Garrett KA. 2002. Relevance of integrated disease management to resistance durability.Euphytica 124: 245–252.
Mundt CC. 2015. Durable resistance: a key to sustainable management of pathogens and pests. Genet Evol 27: 446–455.
Nega A. 2014. Review on concepts in biological control of plant pathogens. J Biol Agric Health 4: 33–35.
Nelson R, Wiesner-Hanks T, Wisser R and Balint-Kurti P. 2018. Navigating complexity to breed disease-resistant crops. Nature Rev Genet 19(1):21-33.
Oerke EC and Dehne HW. 2004. Safeguarding production losses in major crops and the role of crop protection. Crop Prot 23: 275–285.
Oliva A, Lahoz E, Contillo R and Aliott G. 1999. Fungistatic activity of Rutagraveolens extract and its allelochemicals. J Chem Ecol 25: 519–526.
Padmanabhan SY. 1973 The great Bengal famine. Ann Rev Phytopathol 11(1): 11-24.
Pal KK and McSpadden GB. 2006. Biological Control of Plant Pathogens. The Plant Health Instructor.
Palloix A, Ayme V and Moury B. 2009. Durability of plant major resistance genes to pathogens depends on the genetic background, experimental evidence and consequences for breeding strategies. New Phytol 183:190–199.
Pande SM, Sharmal S, Kumari PM, Gaur W, Chen L, Kaur W, MacLeod A, Basandrai, D, Basandrai A, Bakr JS, Sandhu HS. Tripathi and Gowda CLL. 2009. Integrated foliar diseases management of legumes”, International Conference on Grain Legumes: Quality Improvement, Value Addition and Trade, February 14-16, Indian Society of Pulses Research and Development, Indian Institute of Pulses Research, Kanpur, India, 143-161.
Pietrusinska A, Czembor JH and Czembor PC. 2011. Pyramiding two genes for leaf rust and powdery mildew resistance in common wheat. Cereal Res Commun 39:577–588.
Poland J and Rutkoski J. 2016. Advances and challenges in genomic selection for disease resistance. Annu Rev Phytopathol 54:79–98.
Prakasam V, Valluvaparidasan V, Raguchander T, Prabakar K, Thiruvudainambi S. 1997. Field crop disease. Coimbatore (India): A. E. Publications 111–112.
Puri S. 2016. Eco-friendly management strategies for soil borne plant pathogens. Int J Adv Res Biol Sci 3(1):69-75.
Ribes S, Fuentes A, Talens P, Barat JM, Ferrari G and Donsi F. 2016. Influence of emulsifier type on the antifungal activity of cinnamon leaf, lemon and bergamot oil nanoemulsions against Aspergillus Niger. Food Contr 73: 784–795.
Rozewicz M, Wyzinska M and Grabinski J. 2021.The Most Important Fungal Diseases of Cereals— Problems and Possible Solutions, Agronomy 11:714.
Rutkoski JE, Heffner EL and Sorrells ME. 2011. Genomic selection for durable stem rust resistance in wheat. Euphytica, 179:161–173.
Santillan Martínez MI, Bracuto V, Koseoglou E, Appiano M, Jacobsen E, Visser RG, F, Wolters AMA and Bai Y. 2020. CRISPR/Cas9-targeted mutagenesis of the tomato susceptibility gene PMR4 for resistance against powdery mildew. BMC Plant Biol 20:284.
Scopa A and Dumontet S. 2007. Soil solarization: effects on soil microbiological parameters J. Plant Nutr 30:537–547.
Shafir S, Dag A, Bilu A, Abu-Toamy M and Elad Y. 2006. Honey bee dispersal of the biocontrol agent Trichoderma harzianum T39: effectiveness in suppressing Botrytis cinerea on strawberry under field conditions. Eur J Plant Pathol 116:119–128.
Simmons CW, Higgins B. Staley, S, Joh LD, Simmons BA, Singer SW, Stapleton JJ. and Vander Gheynst JS. 2016. The role of organic matter amendment level on soil heating, organic acid accumulation, and development of bacterial communities in solarized soil. Appl Soil Ecol 106:37-46.
Singh J, Dutta T, Kim KH, Rawat M, Samddar P and Kumar P. 2018. Green synthesis of metals and their oxide nanoparticles: Applications for environmental remediation. J Nanobiotechnol 16:84.
Singh M, Mallick N, Chand S, Kumari P, Sharma JB, Sivasamy M, Jayaprakash P, Prabhu KV and Jha SK. 2017.Marker-assisted pyramiding of Thinopyrum-derived leaf rust resistance genes Lr19 and Lr24 in bread wheat variety HD2733. J genet 96(6):951-7.
Stirling M and Stirling G. 1997. Disease Management: Biological Control in Plant Pathogens and Plant Diseases. Eds. J. Brown and H. Ogle, 427–439.
Strange RN. 2003. Introduction to Plant Pathology. Chichester, UK: Wiley. 464.
Sundram S, Meon S, Seman IA and Othman R. 2011. Symbiotic interaction of endophytic bacteria with arbuscular mycorrhizal fungi and its antagonistic effect on Ganoderma boninense. J Microbiol 49(4):551-557.
Susanto A, Sudharto PS and Purba RY. 2005. Enhancing biological control of basal stem rot disease (Ganoderma boninense) in oil palm plantations. Mycopathologia, 159(1):153-157.
Tabrez S, Musarrat J, Al-khedhairy AA. 2016. Colloids and surfaces B: biointerfaces countering drug resistance, infectious diseases, and sepsis using metal and metal oxides nanoparticles: current status, Colloids Surf. B Biointerfaces 146:70–83.
Tozlu E, Tekiner N, Kotan R and Serkan O. 2018. Investigation on the biological control of Alternaria alternata. Indian J Agric Sci 88: 1241–1247.
Ullstrup AJ. 1972. The impacts of the southern corn leaf blight epidemics of 1970-1971. Ann review Phytopathol 10(1): 37-50.
Vallieres C and Avery S V. 2017. Metal-based combinations that target protein synthesis by fungi. Adv Microb Physiol 70: 105–121.
Vanblaere T, Flachowsky H, Gessler C and Broggini GA. 2014. Molecular Characterization of Cisgenic Lines of Apple ‘Gala’ Carrying the Rvi6 Scab Resistance Gene. Plant Biotechnol J 12:2–9.
Wang D, Lin Z, Kai LI, Ying MA, Wang L, Yang YQ, Yang YH and Zhi HJ. 2017 Marker-assisted pyramiding of soybean resistance genes RSC4, RSC8, and RSC14Q to soybean mosaic virus. J Integr Agric 16: 2413–2420.
Wu J, Zeng Q, Wang Q, Liu S, Yu S, Mu J, Huang S, Sela H, Distelfeld A, Huang L and Han D. 2018. SNP-based pool genotyping and haplotype analysis accelerate fine-mapping of the wheat genomic region containing stripe rust resistance gene Yr26. Theor Appl Genet 131(7):1481-96.
Zaker M. 2016. Natural plant products as eco-friendly fungicides for plant diseases control -a review. Agric For 14:134–141.
Zhang H, Xie J, Fu Y, Cheng J, Qu Z, Zhao Z, Cheng S, Chen T, Li B, Wang Q and Liu X. 2020. A 2-kb Mycovirus Converts a Pathogenic Fungus into a Beneficial Endophyte for Brassica Protection and Yield Enhancement. Mol Plant 13: 1420–1433.
Zhang J. 2007. The potential of a new fungicide fludioxonil for stem-end rot and green mold control on Florida citrus fruit. Postharvest Biol Technol 46: 262–270.