DOI: https://doi.org/10.56669/NCBE8246
ABSTRACT
Agriculture generates large quantities of wastes, most of which are not used productively and poses environmental and health risks. One sensible way to reduce the environmental and health risks caused by agricultural wastes (agro-wastes) is to convert these wastes into biofertilizer. Since agricultural wastes usually contain sugars, amino acids, organic acids, fats, oils, waxes, resins, pigments, proteins and minerals that can serve as a source of nutrients for microbial growth, it can be used as a carrier in the production of biofertilizers. Biofertilizers derived from agricultural wastes are economically viable and are considered an important component of sustainable agriculture as they utilize modern recycling methods, reduce greenhouse gas emissions, avoid landfill costs and improve soil health and crop yields. In this sense, this review aims to address (i) an overview of agro-wastes biofertilizer production, (ii) selection, characterization, and pre-treatment of agro-wastes as carrier material, (iii) isolation, characterization, and selection of desired microbial inoculants, (iv) inoculums preparation and inoculating the carrier and curing, (v) quality control and optimization, (vi) preservation and storage, and (vii) mass production of agro-waste biofertilizers. The potential of using agro-waste biofertilizers to improve soil health and crop yields while reducing environmental hazards is discussed.
Keywords: Agricultural waste, greenhouse gas emissions, soil carbon sequestration, soil health, and anaerobic digestion
INTRODUCTION
The demand for food production for a growing population and the necessity for sustainable agriculture have sparked interest in converting agricultural wastes into biofertilizers. Intensive agriculture produces a large number of agricultural wastes (agro-wastes), which mostly consist of crop residues, animal manure, and organic by-products (Sadh et al., 2018). Crop residues can be categorized into field residues and process residues. Field residues are what remain on the field after crops are harvested. Field residues include straw, stalks, stems, leaves and seed pods, while processing residues – husks, roots, seeds, bagasse and molasses – are what is left after processing the crop into another important resource. Even while agro-wastes are typically used for making organic fertilizers, animal feed, and other processes like making biofuel, biofertilizer, bioenzymes, and biopolymers, most of it is wasted and disposed (Sadh et al., 2018; Puglia et al., 2021). Indiscriminate dumping and improper management of agro-wastes are major environmental issues that pose a threat to the ecosystem and human health. For example, burning agro-wastes causes air pollution through the emission of particulate matter and toxic gases such as carbon monoxide (CO), sulfur dioxide (SO2), nitrogen oxides (NOx), gaseous hydrochloric acid (HCl), volatile organic compounds (VOC), carcinogenic polycyclic aromatic hydrocarbons (PAH), dioxins and furans, etc. (Shyamsundar et al., 2019). The burning of straw also contributes significantly to the formation of suspended particles [fine particles (PM2.5) and coarse dust particles (PM10)] that deteriorate the air quality and cause global warming by emitting greenhouse gases such as methane (CH4) and nitrous oxide (N2O). Significant losses of plant nutrients, such as nitrogen (~40%), potassium (~33%), and sulfur (~45%), are a worry for the agro-ecosystem in south-east Asia, particularly in India, due to the prevalent practice of burning straw (Shyamsundar et al., 2019). Likewise, dumping agro-wastes releases greenhouse gases such as CH4 and other harmful gases, mainly hydrogen sulfide (H2S). During the dumping and decomposition process, agricultural waste contaminated by pesticides releases toxic elements into groundwater, polluting the groundwater and discharging it into rivers, lakes, and ponds, causing eutrophication of water bodies (Puglia et al., 2021). Dumping of agro-wastes can also contaminate the surrounding environment, cause disease in humans and animals, and serve as a vector for subsequent fungal diseases of crops (Puglia et al., 2021). Prudent reuse and recycling of agro-wastes can mitigate the negative effects on the environment, health and safety risks, and aesthetic concerns resulting from improper handling of agro-wastes.
Chemical fertilizers have long been used in agriculture to provide essential nutrients to plants and increase crop yields. The overuse of chemical fertilizers has, however, resulted in environmental pollution, including greenhouse gas emissions and global warming, soil acidification, water eutrophication, loss of biodiversity, pest outbreaks, nutrient loss and its biomagnification in food chains, all of which pose challenges to our health (Good and Beatty, 2011). Biofertilizers, on the other hand, use beneficial microorganisms to enhance nutrient availability and promote plant growth (Kumar et al., 2022). Furthermore, biofertilizers can not only enhance plant growth and yield but also mitigate the adverse effects of chemical fertilizers due to the potential combination of organic wastes and microorganisms (Kumar et al., 2022). Agro wastes consist of various components, including soluble ones like sugars, amino acids, and organic acids, insoluble ones like cellulose and lignin, as well as other components like fats, oils, waxes, resins, pigments, proteins, and minerals that can serve as a source of nutrients for microbial growth (Bala et al., 2023). Agricultural wastes, such as decaying plants, is a major source of organic matter (Riseh et al., 2024). Agro-wastes are the most economical resource in the production of biofertilizers for sustainable agriculture.
Agro-wastes contain diverse biological, chemical, and physical characteristics since it originates from a variety of sources, including animal dung and organic byproducts (Bala et al., 2023). Plant growth promoting microorganisms (PGPM) have been identified in agro-wastes. These include phosphate-solubilizing microbes, N-fixers, siderophores producers, phytohormone producers, and K solubilizing microbes (Puglia et al., 2021). Microorganisms that may produce the enzymes amylase, cellulase, chitinase, pectinase, protease, and lipase are also present in agro-wastes (Bala et al., 2023). These have a major role in the degradation of agro-wastes. These harmonious mixture of microorganisms in agro-wastes establish symbiotic or associative relationships with plants, which facilitates the conversion of complex organic compounds in agro-wastes into forms readily assimilated by plants, thereby improving crop growth and yield. Furthermore, the biodegradable waste components in agro-wastes serve as a carrier for microorganisms, extend the life of active microorganisms, and directly benefit crops (Sadh et al., 2018). Since agro-wastes serve as a suitable niche for PGPM, it can be used for the production of a specific biofertilizer (phosphate-solubilizer, N-fixer, K-solubilizer, siderophores and phytohormone producers) or a cocktail of biofertilizers (Sadh et al., 2018). However, due to the fact that naturally occurring microorganisms often do not perform as efficiently as one would anticipate, the introduction of artificially replicated cultures of efficient microorganisms in the form of biofertilizer is essential for accelerating soil microbial processes. Biofertilizers made from agro-wastes can be used on a range of crops due to their significant variances in characteristics and microbial enrichment (Lim and Matu, 2015). Table 1 shows the example of agro-waste biofertilizers in improving fertilizer efficiency and crop performance.
Table 1. Carriers (agro-wastes), inoculants, and performance of agro-waste biofertilizers
Carriers (agro-wastes)
|
Microbial inoculants
|
Targeted plants
|
Performance
|
References
|
Sawdust, rice bran, and condensed molasses
|
Bacillus licheniformis, Gordonia terrae, and Virgibacillus halophilus (thermophilic)
|
Chinese cabbage
|
The germination percentage of Chinese cabbage markedly increased.
|
Chen et al., 2012
|
Vegetable Wastes
|
Bacillus sp. and Pseudomonas sp. (PGPB)
|
Green gram
|
In composting, the isolates decompose waste and enhance the growth of green gram plants.
|
Indumati, 2017
|
Vegetable wastes
|
Clostridium, Bacillus, Pseudomonas, Actinobacteria, and Micrococcus (PGPB)
|
-
|
Biofertilizer potential is conferred on the digestate by these N-fixers and P solubilizers.
|
Raymond et al., 2020
|
Dairy manure
|
Bacillus subtilis, Pseudomonas putida (Mesophilic)
|
Wheat
|
All isolates significantly promoted wheat growth, and anaerobic digestion (AD) was a potential source of PGPB.
|
Qi et al., 2017
|
Organic wastes
|
Bacilli, Cytophaga, Flavobacteria
|
Radish and Chinese cabbage
|
By using organic formulations with this bacteria, radish and Chinese cabbage growth was significantly improved.
|
Anandham et al., 2015
|
Agricultural waste press mud
|
Bacillus wiedmannii, B. altitudinis, B. velezensis, Pseudomonas aeruginosa, Aspergillus niger, (PGPB)
|
-
|
Compared to a non-inoculated substrate, these microbes accelerate the maturation of lignocellulosic waste and increase nutrient content.
|
Naeem et al., 2022
|
Soluble fermented solution (solid state fermentation) of watermelon, guava, papaya, custard apple and pineapple
|
Bacillus sp. and Aspergillus sp.
|
Watermelon guava, papaya, custard apple and pineapple
|
Improved germination, plant growth, and prevent root disease in the plants.
|
Devi and Sumathy, 2017
|
Lignocellulosic substrates in sugarcane, rice, cotton and fruit waste
|
Staphylococcus pseudintermedius, Granulicatella elegans, Kocuria varians (Cellulose and lignin degrading bacterial consortium)
|
Moong
|
Improved plant growth and yield.
|
Gaikwad et al., 2023
|
Cattle manure, poultry litter, pig slurry and onion wastes
|
-
|
Lettuce plant
|
Improved nutrient availability for lettuce plants and increased soil carbon storage.
|
Iocoli et al., 2019
|
Mixed dairy manure and food wastes
|
-
|
Tomato
|
Dairy manure and FW digestate improved tomato yield with better physicochemical properties than synthetic fertilizer.
|
Barzee et al., 2019
|
Rice straw
|
Aspergillus flavus, and Trichoderma citrinoviride
|
-
|
Improved fertilizer efficiency.
|
Saini et al., 2023
|
Organic wastes (crop residue) and food waste (rotton)
|
-
|
Chili
|
Improved plant growth and yield.
|
|
Solid state fermentation product from watermelon, papaya, pineapple, citrus orange, and banana waste
|
-
|
Mustard
|
Increased crop growth and yield.
|
Lim and Matu, 2015
|
Agro-wastes
(Sesame straw) plus cow dung
|
Earthworm species: Eisenia fetida and Perionyx sansibaricus
|
-
|
Improved fertilizer efficiency.
|
Panwar and Tripathi, 2021
|
While there are many formulations for making biofertilizers, agro-waste-based formulations have attracted a lot of attention not only because of their potential to improve soil fertility but also to reduce the environmental impact associated with waste disposal. Biofertilizers derived from the recycling of wastes are now considered a cornerstone of sustainable agriculture as they utilize modern recycling techniques, reduce negative carbon footprints and greenhouse gases, and circumvent the cost of landfilling (Mandal et al., 2024). However, with a share of only 1–2% of the global market, the use of agro-waste biofertilizers is still quite low compared to synthetic products or biofertilizers from other sources (Bala et al., 2023). The aim of this review is to provide an overview of the production of biofertilizers from agro-wastes and to highlight their importance in sustainable agriculture. By exploring the production process and its potential benefits, this study aims to contribute to the understanding and adoption of agro-waste-based biofertilizers as an effective strategy for sustainability and productivity in agriculture.
Production of biofertilizer from agro-wastes
Composting agro-wastes is an effective way to produce biofertilizers for personal use, but at the production scale, it may be less effective, requiring a scalable method (Noor et al., 2024). Important considerations for biofertilizer production include selection of appropriate microbial strains, formulation type, carrier materials, and field uses. Biofertilizers are typically prepared as carrier-based inoculants, which makes biofertilizers easy to handle, store for a long time and have high efficiency. The basic process for producing biofertilizers (including agro-waste-based biofertilizers) on a commercial scale consists of the following steps: (i) selection, characterization, and pre-treatment of the carrier material (in this case, agro-waste); (ii) isolation, characterization, and selection of desired microbial inoculants, such as PGPM, N2 fixer, phosphate solubilizer; (iii) inoculums preparation and inoculating the carrier, and curing; (iv) quality control and optimization; and (v) preservation and storage (Puglia et al., 2021). Through these steps, particularly the nutrient content, microbial population, and overall quality of the biofertilizer are optimized, guaranteeing its safety and effectiveness for use in agriculture. Below is a detailed description of these steps:
Selection, characterization, and pre-treatment of the carrier material (agro-waste)
The proper selection of carrier materials is essential for maintaining the viability and proper number of microorganisms in liquid formulations or carrier-based materials like granular, powder, or slurry (Sahu and Brahmaprakash, 2016). The properties of a good carrier material for the seed inoculation are: (1) non-toxic to the inoculating bacterial strain; (2) non-toxic to the plant; (3) good moisture absorption capacity; (4) easy to process and free of lump-forming materials; (5) easy to sterilize by autoclaving or gamma irradiation; (6) good adhesion to the seed; (7) good pH buffering capacity; (8) available in sufficient quantities; (9) inexpensive; and (10) easy handling and transportability (Mandal et al., 2024). As carriers for the production of agro-waste-based biofertilizers, various types of agro-wastes are used, including crop residues (plant remains like stalks, leaves, husks, crushed corn cobs, and sawdust), vegetative waste, animal manure, compost, biogas slurry, biochar, and organic by-products. The carrier material should be processed (e.g., dried, coarsely ground to obtain particles of 0.1–1.5 mm in size) and sterilized before use (Lim and Matu, 2015). Particles coarser than 0.1–1.5 mm in size can cause ‘balling-up’ when wetted and adhere poorly to the seed coat at the time of inoculation. The sterilization can be performed by autoclaving or Gamma-irradiation. It is important to note that during the autoclave process, some carrier materials change their properties and become toxic to certain bacterial strains (Lim and Matu, 2015). Because gamma-irradiation virtually completely preserves the material's chemical and physical characteristics, it is considered as the best method of carrier sterilization (Sahu and Brahmaprakash, 2016). It may be necessary to pre-treat carrier materials with sulfuric acid, nitric acid, hydrochloric acid, zinc metal, cupric sulphate, boric acid, calcium carbonate, and distilled water, depending on their properties (Mandal et al., 2024). Generally, carriers are mixed with calcium carbonate to neutralize the pH (6.5-7.0) and mixed with 10% water before sterilization (Lim and Matu, 2015). In order to create the ideal environmental conditions necessary for the inoculant organism's growth and survival during the curing and storage of biofertilizers, it is crucial to analyze the properties (mainly moisture content, bulk density, porosity and water absorption capacity) of the carrier materials (Lim and Matu, 2015).
Isolation, characterization, and selection of desired microbial inoculants
Microorganisms used for biofertilizer production are usually isolated from their natural habitats (Kumar et al., 2022), so the ideal source for isolating microorganisms for production of agro-waste biofertilizers is the carrier materials or the soil/rhizosphere of the crop to be inoculated. Isolating, identifying and functionally characterizing potentially active and non-toxic microorganisms that can aid in plant growth is the key to producing biofertilizers from agro-wastes (Puglia et al., 2021). Using standard laboratory methods like qualitative testing or differential culture media, the functional characterization of the microbial strains can be accomplished. The selection of a pure culture of the target strain(s) should be done based on the desired functionalities of biofertilizers in the field, such as nutrient solubilization and mobilization, nitrogen fixation, phosphorus solubilization, siderophore formation, phytohormone synthesis, or a combination of these functions (Sartori et al., 2021). Additional in vitro testing, such as growth on selective media and quantitative testing to ascertain potency, can be performed to confirm the appropriateness of the selected strain or strains. Before strains can be tested in the field, they must first be rigorously tested in greenhouse experiments to determine their efficacy.
Inoculums preparation and inoculating the carrier and curing
Preparation of an efficient inoculant involves a number of steps, including attaching one or more microbial strains to a specific carrier along with adhesive or additives that ensure the cells' protection during transportation and storage (Mandal et al., 2024). The inoculants must have a long shelf-life, as they are often stored under unfavorable conditions (e.g., high temperatures and exposure to light). This means that the microorganisms must be resilient or have a higher capacity to live in large quantities under unfavorable conditions. To ensure that host plants derive the greatest benefit from inoculation, a well-formulated mixture must effectively introduce the microorganisms into the soil and increase their activity (Kumar et al., 2022). For an inoculant to be readily embraced by farmers, it must be affordable, easy to handle, and ensure that the microorganisms are delivered to the target plant in the most appropriate way. Better inoculant formulations are urgently needed in order to create and market agro-waste biofertilizers that will meet agricultural needs and be more successful, stable over time, and of higher quality.
During the production of biofertilizers, there are a few crucial processes that need to be carefully examined. The decisions taken at these points can determine whether the inoculation is successful or not. The choice of which microorganisms to inoculate is very important. The inoculant strain's genetic stability, capacity to benefit target crops, ability to compete with native populations, ability to migrate from the inoculation site to the hosts, and capacity to survive in unfavorable soil in the absence of the host are some of its most important and desirable traits (Sahu and Brahmaprakash, 2016). Other crucial characteristics that are looked for during biofertilizer production include the strain's ability to grow in laboratory settings (with the exception of AMF, which cannot grow without a host plant), grow or survive in carriers (during curing or storage), on seeds, and in soil, as well as its compatibility with agrochemical products that may be used on seeds (Herrmann and Lesueur, 2013). Along with maintaining its functional properties, the live inoculant must be able to withstand a variety of technological challenges during the production processes. Bacterial inoculants are typically cultured in liquid media to achieve high biomass yields (Allouzi et al., 2022). Growing bacteria on a large scale in pure cultures in bioreactors is a well-accepted standard procedure. The physiological and biochemical characteristics of the specific strain or the type of inoculant to be produced are intimately correlated with the media composition and growth conditions (temperature, pH, agitation, aeration, etc.) (Herrmann and Lesueur, 2013). The bacterial cultures obtained are used to inoculate different carriers (encapsulation or impregnation of particles) or, after adding various additives, liquid formulations can be produced (Allouzi et al., 2022). The addition of carbon sources or cell protectants to biofertilizers could improve their shelf-life and efficiency. Some supplementary nutrients and/or cell protectants commonly used with carrier materials for maximizing cell viability and extending shelf-life include sucrose, maltose, trehalose, molasses, glucose, and glycerol (Agarwal et al., 2021). The addition of carriers, stabilizers, wetting agents, and dispersing agents to formulated goods also improves their shelf life, viability, and efficiency. The standard procedure for blending (mixing broth with the carrier) and curing is as follows: (1) grow the culture in a fermenter until the population reaches 109 cells/ml; (2) mix the inoculum broth with the finely ground and sterilized carrier; (3) add the broth at a ratio of 1/3 of the water holding of the carrier; (4) mix the broth culture with the sterilized carrier thoroughly and aseptically; and (5) allow the blended carrier for curing for 24 hours to ensure acclimatization of the strain with the carrier (Herrmann and Lesueur, 2013; Lim and Matu, 2015).
Quality control and optimization
For biofertilizers, including agro-waste biofertilizers to be successful and be accepted by farmers, their quality must be guaranteed. Maintaining the efficacy and integrity of biofertilizers is largely dependent on quality control procedures. By closely monitoring key quality criteria, regulatory requirements for biofertilizers can be met and the expected yield benefits for crops achieved. Quality control in the production of biofertilizers is crucial for several reasons: (1) It ensures that the active microorganisms are present in the biofertilizer in the desired concentration per gram or milliliter. This is important because the concentration and viability of the beneficial microorganisms contained in the biofertilizers determine how effective they are. (2) The efficacy and efficiency of biofertilizers might be adversely affected by contaminants present in it. Therefore, strict quality control procedures are necessary to reduce the impurities and to ensure the purity of the final product. (3) The production of biofertilizers relies heavily on quality control to ensure consistency and reliability. Producing biofertilizers that meet certain quality parameters can provide farmers with reliable results. This promotes confidence and trust in the product and helps it succeed in the market as a whole (Agarwal et al., 2021).
The production of biofertilizers requires careful monitoring of several key quality parameters. The parameters can vary depending on what type of biofertilizer is produced and the standards set by regulatory agencies. The following are some of the most important quality parameters to consider (Sahu and Brahmaprakash, 2016; Agarwal et al., 2021; Saif et al., 2021; Mandal et al., 2024):
Microbial Density: Among the most critical quality parameters in biofertilizers is the concentration or density of beneficial microorganisms. Having a sufficient number of active microorganisms in a biofertilizer will ensure its effectiveness. Measurement of the microbial density at the time of manufacture as well as following expiration is a good way to guarantee the product's viability. At 25–30°C, the inoculant should contain a minimum of 108 viable cells per gram of carrier.
Contamination Levels: Biofertilizer production is susceptible to contamination by non-target microorganisms. To keep the biofertilizer pure and effective, contamination levels must be monitored and controlled. It is important to set and follow guidelines for acceptable levels of contamination during production.
pH and Moisture: Two critical variables that can impact microbial activity and viability in biofertilizers are their pH level and moisture content. The microorganisms in the biofertilizer benefit from optimal conditions, which are maintained by monitoring and regulating these parameters. Inoculants should have a pH between 6.0 and 7.5.
Microbial Strain and Carrier: The precise labeling and conformity of the biofertilizer's microbial strains to the intended formulation are essential. Furthermore, the carrier material must be of the highest caliber and devoid of any impurities that can impair the effectiveness of the biofertilizer.
Expiry Period: In order to guarantee the product's safety and effectiveness, biofertilizers must have an expiration date. Based on how long the microorganisms in the biofertilizer are viable and stable, the expiration date of biofertilizer should be determined. The inoculants should have at least 6 months shelf-life from the manufacturing date in case of carrier based and 9 months in case of liquid based (Saif et al., 2021).
Biofertilizer manufacturers can make sure that their products fulfill the required criteria and provide farmers with the benefits they want by putting in place strong quality control procedures and regularly monitoring these important metrics. By giving farmers reliable and efficient biofertilizer options, this not only helps the biofertilizer industry succeed but also advances sustainable agricultural practices.
Preservation and storage
Agro-waste biofertilizers, as well as other biofertilizers, are usually stored in liquid form or dry form (Sakpirom et al., 2021). The loss of liquid due to evaporation and leakage must be prevented in liquid storage. It is necessary to agitate the contents of the vessel before applying it to soils with a spreader or watering system. One of the main advantages of liquid storage is the small loss of nutrients (especially nitrogen) (Allouzi et al., 2022). In a dry storage, the biofertilizer is dried in dry and hot weather. The main advantage of this storage method is the reduction of fertilizer volume and weight, while the disadvantage is that significant losses of nutrients, especially nitrogen, from biofertilizers are usually observed. The inoculant should be packed in low-density polythene bags with size of 50–75 micron for storage. Every product should have a clearly visible label that includes the following details: product name, microbial inoculant name, bioinoculant activity, intended crop, manufacturer's name and address, type of carrier, batch and code numbers, date of manufacture, expiration date, net quantity intended for 0.4 hectares, and storage guidelines (Sakpirom et al., 2021). It must be devoid of any impurities or contamination from other microbes.
Mass-scale production of agro-waste biofertilizer
Agro-waste-based fertilizers could be a more cost-effective source of nutrients than synthetic inorganic fertilizer sources like urea and diammonium phosphate because of their vast amounts and the possibility for mass-scale, low-cost manufacture (Sartori et al., 2021). Microbial fermentation (bio-based fermenter) in bioreactors produced value-added products for a number of industries, including agro-industrial waste transformation, biofuel production, food modification, and pharmaceuticals (Bala et al., 2023). There are two types of fermentation processes: submerged fermentation (SmF) and solid-state fermentation (SSF) (Sadh et al., 2018). While SSF uses solid substrates and requires little to no water, SmF refers to the microbial catabolism of components in the presence of water or a water-based nutritional medium, primarily in bioreactors where aeration and pH are regulated. To choose the best mode of fermentation, several variables must be optimized, including the type of biomass (e.g., lignocellulosic), the type of microorganisms (e.g., N2 fixers, phosphate solubilizers, plant growth-promoting bacteria, arbuscular mycorrhizal fungi), the type of bioformulation (solid and liquid), and the method of scaling up (Sartori et al., 2021). Anaerobic digestion (AD) and composting (aerobic digestion) are conversion processes generally used for the production of biofertilizers from agricultural waste in bio-based digesters (Ibanez et al., 2023; Mandal et al., 2024). The additional soil-improving properties of digestate show that it is a better soil nutrient conditioner due to its PGPB, which releases various nutrients, and the digestates have lower leaching and improved water retention capacity.
During AD, microorganisms break down agricultural waste in the absence of oxygen, producing biogas and nutrient-rich substances (Mandal et al., 2024). AD has been used to remove sludge from sewers for over a century and a half. Unlike chemical fertilizers, AD bio-fertilizers can be produced anywhere, at a cheap cost, using a greater range of source materials, such as agricultural, commercial, and residential wastes. Over the past few decades, the number of industrial-scale AD plants has increased worldwide (Mandal et al., 2024). The primary goals of AD facilities are the production of biogas and the treatment of wastewater (Noor et al., 2024). As an additional source of revenue, the solid waste from AD plants is handled to create compost, biofertilizer, and soil amendment (Sharma et al., 2023). Liquid products following AD can be used as a biofertilizer in many places (Mandal et al., 2024). Digestate bio-fertilizer demand will be contingent upon quality standards being met. Nevertheless, because of contaminants and pathogen count, agro-waste quality must be maintained throughout the synthesis of AD-based biofertilizers (Sharma et al., 2023). Physical impurities (glass and plastics), chemical pollutants (pesticides and heavy metals), organic contaminants (dioxin-like polychlorinated biphenyls, dibenzo-p-furans, and dioxins), and biological impurities (plant and animal pathogens) can all be fixed by applying various pretreatment techniques (Sharma et al., 2023). Dewatering, sanitizing, and controlling ammonia levels are required prior to employing AD as a soil amendment, solid compost, and biofertilizer. Enhanced AD efficiency would aid in the production of bio-fertilizers as well, since they are a byproduct of the AD process.
The four main processes that AD uses to break down an organic substrate of agro-waste are hydrolysis, acetogenesis, acidogenesis, and methanogenesis (Mandal et al., 2024; Noor et al., 2024). Larger molecules such as lipids, proteins, nucleotides, and carbohydrates are broken down into smaller molecules such as fatty acids, amino acids, and glucose by the hydrolysis process carried out by a fermentative bacterial community. During acidogenesis, the smaller molecules are then broken down into volatile fatty acid-based compounds (such as butyrate, propionate, and acetate) and various by-products (CO2, NH3, and H2S). Following acidogenesis, the volatile fatty acid components are converted into H2, acetate, and CO2 by the acetogenesis mechanism. Ultimately, acetate is mostly converted to CH4 by methanogens. AD-based biofertilizers have been shown to improve soil quality and increase crop yields (Sharma et al., 2023). In addition, digestate can be used as a soil amendment to improve dissolved organic matter, resulting in rapid and delayed nutrient release and increasing the soil’s ability to retain water (Sharma et al., 2023).
Agro-wastes can be managed by using composting or aerobic digestion techniques, in which microorganisms break down organic materials in an oxygen-rich environment (Noor et al., 2024). The final product is commonly used as a soil amendment. Various methods of composting exist, from backyard compost piles to large-scale operations that decompose thousands of tons of organic waste every year. In composting, three main stages are involved: the mesophilic phase (55 °C for 5-7 days), the thermophilic phase (75 °C for 2-3 days), and the maturation/cooling phase, where a variety of mesophilic and thermophilic microorganisms converge to stabilize and convert organic matter into humus (Noor et al., 2024). As composting progresses, different microorganisms become active and persist in different stages of composting. There are a variety of microbiomes involved in composting, such as fungi, bacteria, actinomycetes, and protozoa, which change depending on the temperature, moisture content, C/N ratio, and organic matter composition (Mandal et al., 2024). Because there are readily available C sources at the beginning, mesophiles carry out the first phase's breakdown of organic substances. During the oxidation of organic material, mesophilic microbes generate heat, which decomposes the bulk of the biodegradable components and enhances the integrity of the organic residue. The rise in temperature renders pathogens and weed seeds inactive. The mesophilic phase is succeeded by the thermophilic phase. During the thermophilic stage, the growth of mesophilic microorganisms will be replaced and regulated by heat-resistant thermophilic microorganisms. Just before compost reaches maturity, the temperature gradually drops and returns to the mesophilic stage because rising temperatures hasten compound breakdown and, consequently, nutrient exhaustion. During the cooling process, the mesophilic microorganisms in the compost become active and break down the remaining organic matter. The organic matter continues to decompose until stable humic compounds are produced (Noor et al., 2024). In order to optimize its fertilizing potential, composting necessitates continuous monitoring of certain parameters such as oxygen content, carbon to nitrogen ratio, pH, particle size, moisture content, curing time, and temperature (Sharma et al., 2023; Mandal et al., 2024).
Figure 1 shows a simple schematic diagram of agro-waste biofertilizer production.
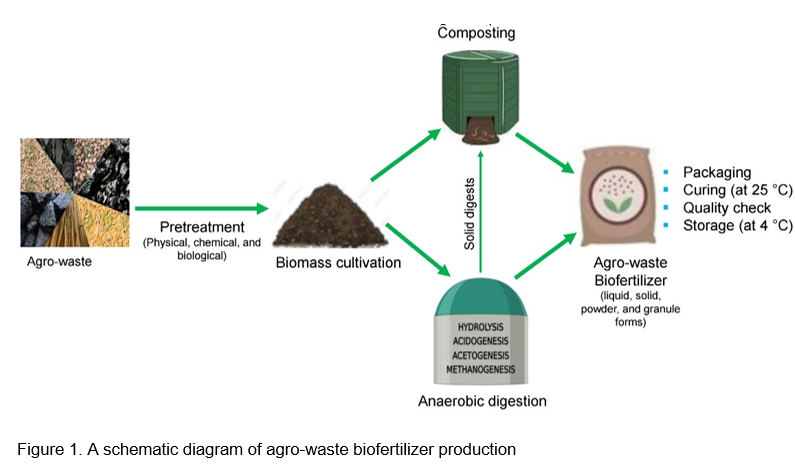
CONCLUSIONS
Agro-wastes are converted into biofertilizers through a series of processes that include collection, pre-treatment, microorganism inoculation, fermentation, maturation, and quality testing. Through these processes, nutrients, microbial populations, and overall quality of the biofertilizer are optimized, ensuring its efficacy and safety. Biofertilizer production with agro wastes not only addresses the problem of waste management, it also promotes sustainable agriculture and economic benefits to farmers. By converting agro-wastes to biofertilizers can reduce the environmental impact associated with waste disposal, minimize the need for chemical fertilizers, and improve soil health in the long run.
Even while the benefits of agro-waste biofertilizer for sustainable agriculture have been acknowledged, it is clear that their application in agronomic practice and industrial production is still far behind expectations. To enable large-scale adoption, mass production, and global marketing, a more clearly defined legal framework is essential. Furthermore, the screening, characterization, and application of innovative agro-waste biofertilizers with the goal of reaching wide adaptation and industrial-scale production are being spurred by developing technologies (e.g., metaomics, encapsulation). However, research and interaction among laboratories, industry, policymakers, and farmers are needed to promote agro-waste biofertilizers as greener alternatives. The culmination of these various contributions ought to be distilled into highly efficient and economically viable bioformulations as a feasible substitute for the chemical fertilizer.
REFERENCES
Agarwal, S., Kumari, S. and Khan, S., 2021. Quality control of biofertilizers. Biofertilizers: Study and Impact, 413-428.
Allouzi, M.M.A., Allouzi, S.M.A., Keng, Z.X., Supramaniam, C.V., Singh, A. and Chong, S., 2022. Liquid biofertilizers as a sustainable solution for agriculture. Heliyon 8: e12609.
Anandham, R., Premalatha, N., Jee, H.J., Weon, H.Y., Kwon, S.W., Krishnamoorthy, R., et al., 2015. Cultivable bacterial diversity and early plant growth promotion by the traditional organic formulations prepared using organic waste materials. Int. J. Recycl. Org. Waste Agric. 4 (4), 279–289.
Bala, S., Garg, D., Sridhar, K., Inbaraj, B.S., Singh, R., Kamma, S., Tripathi, M. and Sharma, M., 2023. Transformation of agro-waste into value-added bioproducts and bioactive compounds: Micro/nano formulations and application in the agri-food-pharma sector. Bioengineering 10: 152.
Barzee, T.J., Edalati, A., El-Mashad, H., Wang, D., Scow, K. and Zhang, R., 2019. Digestate biofertilizers support similar or higher tomato yields and quality than mineral fertilizer in a subsurface drip fertigation system. Front. Sustain. Food Sys. 3: 58.
Chen, C.Y., Mei, H.C., Cheng, C.Y., Lin, J.H., Chung, Y.C., 2012. Enhancing the conversion of organic waste into biofertilizer with thermophilic bacteria. Environ. Eng. Sci. 29 (7), 726–730.
Devi, V. and Sumathy, V.J.H., 2017. Biofertilizer production from agro–wastes. World J. Pharma. Res. 6: 1112-1124.
Gaikwad, A., Jadhav, K. and Nayak, S., 2023. Conversion of lignocellulosic wastes into biofertilizer using bacterial consortium. Curr. Agric. Res. J. 11: 125-136.
Good, A.G. and Beatty, P.H., 2011. Fertilizing nature: a tragedy of excess in the commons. PLoS Biol. 9: e1001124.
Herrmann, L. and Lesueur, D., 2013. Challenges of formulation and quality of biofertilizers for successful inoculation. Appl. Microbiol. Biotechnol. 97: 8859-8873.
Ibanez, A., Garrido-Chamorro, S., Vasco-Cardenas, M.F. and Barreiro, C., 2023. From lab to field: Biofertilizers in the 21st century. Horticulturae, 9: 1306.
Indumathi, D., 2017. Microbial conversion of vegetable wastes for bio fertilizer production. IOSR J. Biotechnol. Biochem. 3 (2), 43–47.
Iocoli, G.A., Zabaloy, M.C., Pasdevicelli, G. and Gómez, M.A., 2019. Use of biogas digestates obtained by anaerobic digestion and co-digestion as fertilizers: Characterization, soil biological activity and growth dynamic of Lactuca sativa L. Sci. Total Environ. 647: 11-19.
Kumar, S., Sindhu, S.S. and Kumar, R., 2022. Biofertilizers: An ecofriendly technology for nutrient recycling and environmental sustainability. Curr. Res. Microb. Sci, 3: 100094.
Lim, S.F. and Matu, S.U., 2015. Utilization of agro-wastes to produce biofertilizer. Int. J. Energy Environ. Eng. 6: 31-35.
Mandal, M., Roy, A., Das, S., Rakwal, R., Agrawal, G.K., Singh, P., Awasthi, A. and Sarkar, A., 2024. Food waste-based bio-fertilizers production by bio-based fermenters and their potential impact on the environment. Chemosphere 353: 141539.
Naeem, U., Afzaal, M., ul Haq, I., Qazi, A., Naeem, A., Mahfooz, Y., 2022. A Comparative Role of Competent Microbes for Value-Added Composting of Agricultural Waste. Research Square Platform LLC.
Noor, R.S., Shah, A.N., Tahir, M.B., Umair, M., Nawaz, M., Ali, A., Ercisli, S., Abdelsalam, N.R., Ali, H.M., Yang, S.H. and Ullah, S., 2024. Recent trends and advances in additive-mediated composting technology for agricultural waste resources: A comprehensive review. ACS Omega, 9: 8632-8653.
Panwar, K.R. and Tripathi, G., 2021. Study of bio-fertilizer produced from agro-waste (Sesame Straw) and cow dung using Eisenia fetida and Perionyx sansibaricus in arid environment. Res. Ecol. 3: 14-20.
Puglia, D., Pezzolla, D., Gigliotti, G., Torre, L., Bartucca, M.L. and Del Buono, D., 2021. The opportunity of valorizing agricultural waste, through its conversion into biostimulants, biofertilizers, and biopolymers. Sustainability 13: 2710.
Qi, G., Pan, Z., Andriamanohiarisoamanana, F.J., Yamashiro, T., Iwasaki, M., Kawamoto, K., Umetsu, K., 2017. Isolation and characterization of plant growth promoting bacteria (PGPB) from anaerobic digestate and their effect on common wheat (Triticum aestivum) seedling growth. Int. J. Environ. Agric. Res. 3 (11), 46–52.
Raymond, F.C., Buraimoh, O.M., Akerele, O.S., Ilori, M.O., Ogundipe, O.T., 2020. Digestate as Biofertilizer for the Growth of Selected Vegetables and Illumina Analysis of Associated Bacterial Community. bioRxiv.
Riseh, R.S., Vazvani, M.G., Hassanisaadi, M. and Thakur, V.K., 2024. Agricultural wastes: a practical and potential source for the isolation and preparation of cellulose and application in agriculture and different industries. Ind. Crops Prod. 208: 117904.
Sadh, P.K., Duhan, S. and Duhan, J.S., 2018. Agro-industrial wastes and their utilization using solid state fermentation: a review. Bioresour. Bioprocess. 5: 1-15.
Sahu, P.K. and Brahmaprakash, G.P., 2016. Formulations of biofertilizers–approaches and advances. Microbial inoculants in sustainable agricultural productivity: Vol. 2: Functional Applications, 179-198.
Saif, S., Abid, Z., Ashiq, M.F., Altaf, M. and Ashraf, R.S., 2021. Biofertilizer formulations. Biofertilizers: Study and Impact, 211-256.
Saini, S., Kuhad, R.C. and Sharma, K.K., 2023. Valorization of rice straw biomass for co-production of bioethanol, biopesticide and biofertilizer following an eco-friendly biorefinery process. Process Saf. Environ. Prot. 173: 823-836.
Sakpirom, J., Nunkaew, T., Khan, E. and Kantachote, D., 2021. Optimization of carriers and packaging for effective biofertilizers to enhance Oryza sativa L. growth in paddy soil. Rhizosphere 19: 100383.
Sartori, R.B., Severo, I.A., de Oliveira, Á.S., Lasta, P., Zepka, L.Q. and Jacob‐Lopes, E., 2021. Biofertilizers from waste. Biofertilizers: study and impact, 517-540.
Sharma, P., Bano, A., Verma, K., Yadav, M., Varjani, S., Singh, S.P. and Tong, Y.W., 2023. Food waste digestate as biofertilizer and their direct applications in agriculture. Biores. Technol. Rep. 23: 101515.
Shyamsundar, P., Springer, N.P., Tallis, H., Polasky, S., Jat, M.L., Sidhu, H.S., Krishnapriya, P.P., Skiba, N., Ginn, W., Ahuja, V. and Cummins, J., 2019. Fields on fire: Alternatives to crop residue burning in India. Science 365: 536-538.
Biofertilizers from Agro-wastes: A Path towards Sustainable Agriculture
DOI: https://doi.org/10.56669/NCBE8246
ABSTRACT
Agriculture generates large quantities of wastes, most of which are not used productively and poses environmental and health risks. One sensible way to reduce the environmental and health risks caused by agricultural wastes (agro-wastes) is to convert these wastes into biofertilizer. Since agricultural wastes usually contain sugars, amino acids, organic acids, fats, oils, waxes, resins, pigments, proteins and minerals that can serve as a source of nutrients for microbial growth, it can be used as a carrier in the production of biofertilizers. Biofertilizers derived from agricultural wastes are economically viable and are considered an important component of sustainable agriculture as they utilize modern recycling methods, reduce greenhouse gas emissions, avoid landfill costs and improve soil health and crop yields. In this sense, this review aims to address (i) an overview of agro-wastes biofertilizer production, (ii) selection, characterization, and pre-treatment of agro-wastes as carrier material, (iii) isolation, characterization, and selection of desired microbial inoculants, (iv) inoculums preparation and inoculating the carrier and curing, (v) quality control and optimization, (vi) preservation and storage, and (vii) mass production of agro-waste biofertilizers. The potential of using agro-waste biofertilizers to improve soil health and crop yields while reducing environmental hazards is discussed.
Keywords: Agricultural waste, greenhouse gas emissions, soil carbon sequestration, soil health, and anaerobic digestion
INTRODUCTION
The demand for food production for a growing population and the necessity for sustainable agriculture have sparked interest in converting agricultural wastes into biofertilizers. Intensive agriculture produces a large number of agricultural wastes (agro-wastes), which mostly consist of crop residues, animal manure, and organic by-products (Sadh et al., 2018). Crop residues can be categorized into field residues and process residues. Field residues are what remain on the field after crops are harvested. Field residues include straw, stalks, stems, leaves and seed pods, while processing residues – husks, roots, seeds, bagasse and molasses – are what is left after processing the crop into another important resource. Even while agro-wastes are typically used for making organic fertilizers, animal feed, and other processes like making biofuel, biofertilizer, bioenzymes, and biopolymers, most of it is wasted and disposed (Sadh et al., 2018; Puglia et al., 2021). Indiscriminate dumping and improper management of agro-wastes are major environmental issues that pose a threat to the ecosystem and human health. For example, burning agro-wastes causes air pollution through the emission of particulate matter and toxic gases such as carbon monoxide (CO), sulfur dioxide (SO2), nitrogen oxides (NOx), gaseous hydrochloric acid (HCl), volatile organic compounds (VOC), carcinogenic polycyclic aromatic hydrocarbons (PAH), dioxins and furans, etc. (Shyamsundar et al., 2019). The burning of straw also contributes significantly to the formation of suspended particles [fine particles (PM2.5) and coarse dust particles (PM10)] that deteriorate the air quality and cause global warming by emitting greenhouse gases such as methane (CH4) and nitrous oxide (N2O). Significant losses of plant nutrients, such as nitrogen (~40%), potassium (~33%), and sulfur (~45%), are a worry for the agro-ecosystem in south-east Asia, particularly in India, due to the prevalent practice of burning straw (Shyamsundar et al., 2019). Likewise, dumping agro-wastes releases greenhouse gases such as CH4 and other harmful gases, mainly hydrogen sulfide (H2S). During the dumping and decomposition process, agricultural waste contaminated by pesticides releases toxic elements into groundwater, polluting the groundwater and discharging it into rivers, lakes, and ponds, causing eutrophication of water bodies (Puglia et al., 2021). Dumping of agro-wastes can also contaminate the surrounding environment, cause disease in humans and animals, and serve as a vector for subsequent fungal diseases of crops (Puglia et al., 2021). Prudent reuse and recycling of agro-wastes can mitigate the negative effects on the environment, health and safety risks, and aesthetic concerns resulting from improper handling of agro-wastes.
Chemical fertilizers have long been used in agriculture to provide essential nutrients to plants and increase crop yields. The overuse of chemical fertilizers has, however, resulted in environmental pollution, including greenhouse gas emissions and global warming, soil acidification, water eutrophication, loss of biodiversity, pest outbreaks, nutrient loss and its biomagnification in food chains, all of which pose challenges to our health (Good and Beatty, 2011). Biofertilizers, on the other hand, use beneficial microorganisms to enhance nutrient availability and promote plant growth (Kumar et al., 2022). Furthermore, biofertilizers can not only enhance plant growth and yield but also mitigate the adverse effects of chemical fertilizers due to the potential combination of organic wastes and microorganisms (Kumar et al., 2022). Agro wastes consist of various components, including soluble ones like sugars, amino acids, and organic acids, insoluble ones like cellulose and lignin, as well as other components like fats, oils, waxes, resins, pigments, proteins, and minerals that can serve as a source of nutrients for microbial growth (Bala et al., 2023). Agricultural wastes, such as decaying plants, is a major source of organic matter (Riseh et al., 2024). Agro-wastes are the most economical resource in the production of biofertilizers for sustainable agriculture.
Agro-wastes contain diverse biological, chemical, and physical characteristics since it originates from a variety of sources, including animal dung and organic byproducts (Bala et al., 2023). Plant growth promoting microorganisms (PGPM) have been identified in agro-wastes. These include phosphate-solubilizing microbes, N-fixers, siderophores producers, phytohormone producers, and K solubilizing microbes (Puglia et al., 2021). Microorganisms that may produce the enzymes amylase, cellulase, chitinase, pectinase, protease, and lipase are also present in agro-wastes (Bala et al., 2023). These have a major role in the degradation of agro-wastes. These harmonious mixture of microorganisms in agro-wastes establish symbiotic or associative relationships with plants, which facilitates the conversion of complex organic compounds in agro-wastes into forms readily assimilated by plants, thereby improving crop growth and yield. Furthermore, the biodegradable waste components in agro-wastes serve as a carrier for microorganisms, extend the life of active microorganisms, and directly benefit crops (Sadh et al., 2018). Since agro-wastes serve as a suitable niche for PGPM, it can be used for the production of a specific biofertilizer (phosphate-solubilizer, N-fixer, K-solubilizer, siderophores and phytohormone producers) or a cocktail of biofertilizers (Sadh et al., 2018). However, due to the fact that naturally occurring microorganisms often do not perform as efficiently as one would anticipate, the introduction of artificially replicated cultures of efficient microorganisms in the form of biofertilizer is essential for accelerating soil microbial processes. Biofertilizers made from agro-wastes can be used on a range of crops due to their significant variances in characteristics and microbial enrichment (Lim and Matu, 2015). Table 1 shows the example of agro-waste biofertilizers in improving fertilizer efficiency and crop performance.
Table 1. Carriers (agro-wastes), inoculants, and performance of agro-waste biofertilizers
Carriers (agro-wastes)
Microbial inoculants
Targeted plants
Performance
References
Sawdust, rice bran, and condensed molasses
Bacillus licheniformis, Gordonia terrae, and Virgibacillus halophilus (thermophilic)
Chinese cabbage
The germination percentage of Chinese cabbage markedly increased.
Chen et al., 2012
Vegetable Wastes
Bacillus sp. and Pseudomonas sp. (PGPB)
Green gram
In composting, the isolates decompose waste and enhance the growth of green gram plants.
Indumati, 2017
Vegetable wastes
Clostridium, Bacillus, Pseudomonas, Actinobacteria, and Micrococcus (PGPB)
-
Biofertilizer potential is conferred on the digestate by these N-fixers and P solubilizers.
Raymond et al., 2020
Dairy manure
Bacillus subtilis, Pseudomonas putida (Mesophilic)
Wheat
All isolates significantly promoted wheat growth, and anaerobic digestion (AD) was a potential source of PGPB.
Qi et al., 2017
Organic wastes
Bacilli, Cytophaga, Flavobacteria
Radish and Chinese cabbage
By using organic formulations with this bacteria, radish and Chinese cabbage growth was significantly improved.
Anandham et al., 2015
Agricultural waste press mud
Bacillus wiedmannii, B. altitudinis, B. velezensis, Pseudomonas aeruginosa, Aspergillus niger, (PGPB)
-
Compared to a non-inoculated substrate, these microbes accelerate the maturation of lignocellulosic waste and increase nutrient content.
Naeem et al., 2022
Soluble fermented solution (solid state fermentation) of watermelon, guava, papaya, custard apple and pineapple
Bacillus sp. and Aspergillus sp.
Watermelon guava, papaya, custard apple and pineapple
Improved germination, plant growth, and prevent root disease in the plants.
Devi and Sumathy, 2017
Lignocellulosic substrates in sugarcane, rice, cotton and fruit waste
Staphylococcus pseudintermedius, Granulicatella elegans, Kocuria varians (Cellulose and lignin degrading bacterial consortium)
Moong
Improved plant growth and yield.
Gaikwad et al., 2023
Cattle manure, poultry litter, pig slurry and onion wastes
-
Lettuce plant
Improved nutrient availability for lettuce plants and increased soil carbon storage.
Iocoli et al., 2019
Mixed dairy manure and food wastes
-
Tomato
Dairy manure and FW digestate improved tomato yield with better physicochemical properties than synthetic fertilizer.
Barzee et al., 2019
Rice straw
Aspergillus flavus, and Trichoderma citrinoviride
-
Improved fertilizer efficiency.
Saini et al., 2023
Organic wastes (crop residue) and food waste (rotton)
-
Chili
Improved plant growth and yield.
Solid state fermentation product from watermelon, papaya, pineapple, citrus orange, and banana waste
-
Mustard
Increased crop growth and yield.
Lim and Matu, 2015
Agro-wastes
(Sesame straw) plus cow dung
Earthworm species: Eisenia fetida and Perionyx sansibaricus
-
Improved fertilizer efficiency.
Panwar and Tripathi, 2021
While there are many formulations for making biofertilizers, agro-waste-based formulations have attracted a lot of attention not only because of their potential to improve soil fertility but also to reduce the environmental impact associated with waste disposal. Biofertilizers derived from the recycling of wastes are now considered a cornerstone of sustainable agriculture as they utilize modern recycling techniques, reduce negative carbon footprints and greenhouse gases, and circumvent the cost of landfilling (Mandal et al., 2024). However, with a share of only 1–2% of the global market, the use of agro-waste biofertilizers is still quite low compared to synthetic products or biofertilizers from other sources (Bala et al., 2023). The aim of this review is to provide an overview of the production of biofertilizers from agro-wastes and to highlight their importance in sustainable agriculture. By exploring the production process and its potential benefits, this study aims to contribute to the understanding and adoption of agro-waste-based biofertilizers as an effective strategy for sustainability and productivity in agriculture.
Production of biofertilizer from agro-wastes
Composting agro-wastes is an effective way to produce biofertilizers for personal use, but at the production scale, it may be less effective, requiring a scalable method (Noor et al., 2024). Important considerations for biofertilizer production include selection of appropriate microbial strains, formulation type, carrier materials, and field uses. Biofertilizers are typically prepared as carrier-based inoculants, which makes biofertilizers easy to handle, store for a long time and have high efficiency. The basic process for producing biofertilizers (including agro-waste-based biofertilizers) on a commercial scale consists of the following steps: (i) selection, characterization, and pre-treatment of the carrier material (in this case, agro-waste); (ii) isolation, characterization, and selection of desired microbial inoculants, such as PGPM, N2 fixer, phosphate solubilizer; (iii) inoculums preparation and inoculating the carrier, and curing; (iv) quality control and optimization; and (v) preservation and storage (Puglia et al., 2021). Through these steps, particularly the nutrient content, microbial population, and overall quality of the biofertilizer are optimized, guaranteeing its safety and effectiveness for use in agriculture. Below is a detailed description of these steps:
Selection, characterization, and pre-treatment of the carrier material (agro-waste)
The proper selection of carrier materials is essential for maintaining the viability and proper number of microorganisms in liquid formulations or carrier-based materials like granular, powder, or slurry (Sahu and Brahmaprakash, 2016). The properties of a good carrier material for the seed inoculation are: (1) non-toxic to the inoculating bacterial strain; (2) non-toxic to the plant; (3) good moisture absorption capacity; (4) easy to process and free of lump-forming materials; (5) easy to sterilize by autoclaving or gamma irradiation; (6) good adhesion to the seed; (7) good pH buffering capacity; (8) available in sufficient quantities; (9) inexpensive; and (10) easy handling and transportability (Mandal et al., 2024). As carriers for the production of agro-waste-based biofertilizers, various types of agro-wastes are used, including crop residues (plant remains like stalks, leaves, husks, crushed corn cobs, and sawdust), vegetative waste, animal manure, compost, biogas slurry, biochar, and organic by-products. The carrier material should be processed (e.g., dried, coarsely ground to obtain particles of 0.1–1.5 mm in size) and sterilized before use (Lim and Matu, 2015). Particles coarser than 0.1–1.5 mm in size can cause ‘balling-up’ when wetted and adhere poorly to the seed coat at the time of inoculation. The sterilization can be performed by autoclaving or Gamma-irradiation. It is important to note that during the autoclave process, some carrier materials change their properties and become toxic to certain bacterial strains (Lim and Matu, 2015). Because gamma-irradiation virtually completely preserves the material's chemical and physical characteristics, it is considered as the best method of carrier sterilization (Sahu and Brahmaprakash, 2016). It may be necessary to pre-treat carrier materials with sulfuric acid, nitric acid, hydrochloric acid, zinc metal, cupric sulphate, boric acid, calcium carbonate, and distilled water, depending on their properties (Mandal et al., 2024). Generally, carriers are mixed with calcium carbonate to neutralize the pH (6.5-7.0) and mixed with 10% water before sterilization (Lim and Matu, 2015). In order to create the ideal environmental conditions necessary for the inoculant organism's growth and survival during the curing and storage of biofertilizers, it is crucial to analyze the properties (mainly moisture content, bulk density, porosity and water absorption capacity) of the carrier materials (Lim and Matu, 2015).
Isolation, characterization, and selection of desired microbial inoculants
Microorganisms used for biofertilizer production are usually isolated from their natural habitats (Kumar et al., 2022), so the ideal source for isolating microorganisms for production of agro-waste biofertilizers is the carrier materials or the soil/rhizosphere of the crop to be inoculated. Isolating, identifying and functionally characterizing potentially active and non-toxic microorganisms that can aid in plant growth is the key to producing biofertilizers from agro-wastes (Puglia et al., 2021). Using standard laboratory methods like qualitative testing or differential culture media, the functional characterization of the microbial strains can be accomplished. The selection of a pure culture of the target strain(s) should be done based on the desired functionalities of biofertilizers in the field, such as nutrient solubilization and mobilization, nitrogen fixation, phosphorus solubilization, siderophore formation, phytohormone synthesis, or a combination of these functions (Sartori et al., 2021). Additional in vitro testing, such as growth on selective media and quantitative testing to ascertain potency, can be performed to confirm the appropriateness of the selected strain or strains. Before strains can be tested in the field, they must first be rigorously tested in greenhouse experiments to determine their efficacy.
Inoculums preparation and inoculating the carrier and curing
Preparation of an efficient inoculant involves a number of steps, including attaching one or more microbial strains to a specific carrier along with adhesive or additives that ensure the cells' protection during transportation and storage (Mandal et al., 2024). The inoculants must have a long shelf-life, as they are often stored under unfavorable conditions (e.g., high temperatures and exposure to light). This means that the microorganisms must be resilient or have a higher capacity to live in large quantities under unfavorable conditions. To ensure that host plants derive the greatest benefit from inoculation, a well-formulated mixture must effectively introduce the microorganisms into the soil and increase their activity (Kumar et al., 2022). For an inoculant to be readily embraced by farmers, it must be affordable, easy to handle, and ensure that the microorganisms are delivered to the target plant in the most appropriate way. Better inoculant formulations are urgently needed in order to create and market agro-waste biofertilizers that will meet agricultural needs and be more successful, stable over time, and of higher quality.
During the production of biofertilizers, there are a few crucial processes that need to be carefully examined. The decisions taken at these points can determine whether the inoculation is successful or not. The choice of which microorganisms to inoculate is very important. The inoculant strain's genetic stability, capacity to benefit target crops, ability to compete with native populations, ability to migrate from the inoculation site to the hosts, and capacity to survive in unfavorable soil in the absence of the host are some of its most important and desirable traits (Sahu and Brahmaprakash, 2016). Other crucial characteristics that are looked for during biofertilizer production include the strain's ability to grow in laboratory settings (with the exception of AMF, which cannot grow without a host plant), grow or survive in carriers (during curing or storage), on seeds, and in soil, as well as its compatibility with agrochemical products that may be used on seeds (Herrmann and Lesueur, 2013). Along with maintaining its functional properties, the live inoculant must be able to withstand a variety of technological challenges during the production processes. Bacterial inoculants are typically cultured in liquid media to achieve high biomass yields (Allouzi et al., 2022). Growing bacteria on a large scale in pure cultures in bioreactors is a well-accepted standard procedure. The physiological and biochemical characteristics of the specific strain or the type of inoculant to be produced are intimately correlated with the media composition and growth conditions (temperature, pH, agitation, aeration, etc.) (Herrmann and Lesueur, 2013). The bacterial cultures obtained are used to inoculate different carriers (encapsulation or impregnation of particles) or, after adding various additives, liquid formulations can be produced (Allouzi et al., 2022). The addition of carbon sources or cell protectants to biofertilizers could improve their shelf-life and efficiency. Some supplementary nutrients and/or cell protectants commonly used with carrier materials for maximizing cell viability and extending shelf-life include sucrose, maltose, trehalose, molasses, glucose, and glycerol (Agarwal et al., 2021). The addition of carriers, stabilizers, wetting agents, and dispersing agents to formulated goods also improves their shelf life, viability, and efficiency. The standard procedure for blending (mixing broth with the carrier) and curing is as follows: (1) grow the culture in a fermenter until the population reaches 109 cells/ml; (2) mix the inoculum broth with the finely ground and sterilized carrier; (3) add the broth at a ratio of 1/3 of the water holding of the carrier; (4) mix the broth culture with the sterilized carrier thoroughly and aseptically; and (5) allow the blended carrier for curing for 24 hours to ensure acclimatization of the strain with the carrier (Herrmann and Lesueur, 2013; Lim and Matu, 2015).
Quality control and optimization
For biofertilizers, including agro-waste biofertilizers to be successful and be accepted by farmers, their quality must be guaranteed. Maintaining the efficacy and integrity of biofertilizers is largely dependent on quality control procedures. By closely monitoring key quality criteria, regulatory requirements for biofertilizers can be met and the expected yield benefits for crops achieved. Quality control in the production of biofertilizers is crucial for several reasons: (1) It ensures that the active microorganisms are present in the biofertilizer in the desired concentration per gram or milliliter. This is important because the concentration and viability of the beneficial microorganisms contained in the biofertilizers determine how effective they are. (2) The efficacy and efficiency of biofertilizers might be adversely affected by contaminants present in it. Therefore, strict quality control procedures are necessary to reduce the impurities and to ensure the purity of the final product. (3) The production of biofertilizers relies heavily on quality control to ensure consistency and reliability. Producing biofertilizers that meet certain quality parameters can provide farmers with reliable results. This promotes confidence and trust in the product and helps it succeed in the market as a whole (Agarwal et al., 2021).
The production of biofertilizers requires careful monitoring of several key quality parameters. The parameters can vary depending on what type of biofertilizer is produced and the standards set by regulatory agencies. The following are some of the most important quality parameters to consider (Sahu and Brahmaprakash, 2016; Agarwal et al., 2021; Saif et al., 2021; Mandal et al., 2024):
Microbial Density: Among the most critical quality parameters in biofertilizers is the concentration or density of beneficial microorganisms. Having a sufficient number of active microorganisms in a biofertilizer will ensure its effectiveness. Measurement of the microbial density at the time of manufacture as well as following expiration is a good way to guarantee the product's viability. At 25–30°C, the inoculant should contain a minimum of 108 viable cells per gram of carrier.
Contamination Levels: Biofertilizer production is susceptible to contamination by non-target microorganisms. To keep the biofertilizer pure and effective, contamination levels must be monitored and controlled. It is important to set and follow guidelines for acceptable levels of contamination during production.
pH and Moisture: Two critical variables that can impact microbial activity and viability in biofertilizers are their pH level and moisture content. The microorganisms in the biofertilizer benefit from optimal conditions, which are maintained by monitoring and regulating these parameters. Inoculants should have a pH between 6.0 and 7.5.
Microbial Strain and Carrier: The precise labeling and conformity of the biofertilizer's microbial strains to the intended formulation are essential. Furthermore, the carrier material must be of the highest caliber and devoid of any impurities that can impair the effectiveness of the biofertilizer.
Expiry Period: In order to guarantee the product's safety and effectiveness, biofertilizers must have an expiration date. Based on how long the microorganisms in the biofertilizer are viable and stable, the expiration date of biofertilizer should be determined. The inoculants should have at least 6 months shelf-life from the manufacturing date in case of carrier based and 9 months in case of liquid based (Saif et al., 2021).
Biofertilizer manufacturers can make sure that their products fulfill the required criteria and provide farmers with the benefits they want by putting in place strong quality control procedures and regularly monitoring these important metrics. By giving farmers reliable and efficient biofertilizer options, this not only helps the biofertilizer industry succeed but also advances sustainable agricultural practices.
Preservation and storage
Agro-waste biofertilizers, as well as other biofertilizers, are usually stored in liquid form or dry form (Sakpirom et al., 2021). The loss of liquid due to evaporation and leakage must be prevented in liquid storage. It is necessary to agitate the contents of the vessel before applying it to soils with a spreader or watering system. One of the main advantages of liquid storage is the small loss of nutrients (especially nitrogen) (Allouzi et al., 2022). In a dry storage, the biofertilizer is dried in dry and hot weather. The main advantage of this storage method is the reduction of fertilizer volume and weight, while the disadvantage is that significant losses of nutrients, especially nitrogen, from biofertilizers are usually observed. The inoculant should be packed in low-density polythene bags with size of 50–75 micron for storage. Every product should have a clearly visible label that includes the following details: product name, microbial inoculant name, bioinoculant activity, intended crop, manufacturer's name and address, type of carrier, batch and code numbers, date of manufacture, expiration date, net quantity intended for 0.4 hectares, and storage guidelines (Sakpirom et al., 2021). It must be devoid of any impurities or contamination from other microbes.
Mass-scale production of agro-waste biofertilizer
Agro-waste-based fertilizers could be a more cost-effective source of nutrients than synthetic inorganic fertilizer sources like urea and diammonium phosphate because of their vast amounts and the possibility for mass-scale, low-cost manufacture (Sartori et al., 2021). Microbial fermentation (bio-based fermenter) in bioreactors produced value-added products for a number of industries, including agro-industrial waste transformation, biofuel production, food modification, and pharmaceuticals (Bala et al., 2023). There are two types of fermentation processes: submerged fermentation (SmF) and solid-state fermentation (SSF) (Sadh et al., 2018). While SSF uses solid substrates and requires little to no water, SmF refers to the microbial catabolism of components in the presence of water or a water-based nutritional medium, primarily in bioreactors where aeration and pH are regulated. To choose the best mode of fermentation, several variables must be optimized, including the type of biomass (e.g., lignocellulosic), the type of microorganisms (e.g., N2 fixers, phosphate solubilizers, plant growth-promoting bacteria, arbuscular mycorrhizal fungi), the type of bioformulation (solid and liquid), and the method of scaling up (Sartori et al., 2021). Anaerobic digestion (AD) and composting (aerobic digestion) are conversion processes generally used for the production of biofertilizers from agricultural waste in bio-based digesters (Ibanez et al., 2023; Mandal et al., 2024). The additional soil-improving properties of digestate show that it is a better soil nutrient conditioner due to its PGPB, which releases various nutrients, and the digestates have lower leaching and improved water retention capacity.
During AD, microorganisms break down agricultural waste in the absence of oxygen, producing biogas and nutrient-rich substances (Mandal et al., 2024). AD has been used to remove sludge from sewers for over a century and a half. Unlike chemical fertilizers, AD bio-fertilizers can be produced anywhere, at a cheap cost, using a greater range of source materials, such as agricultural, commercial, and residential wastes. Over the past few decades, the number of industrial-scale AD plants has increased worldwide (Mandal et al., 2024). The primary goals of AD facilities are the production of biogas and the treatment of wastewater (Noor et al., 2024). As an additional source of revenue, the solid waste from AD plants is handled to create compost, biofertilizer, and soil amendment (Sharma et al., 2023). Liquid products following AD can be used as a biofertilizer in many places (Mandal et al., 2024). Digestate bio-fertilizer demand will be contingent upon quality standards being met. Nevertheless, because of contaminants and pathogen count, agro-waste quality must be maintained throughout the synthesis of AD-based biofertilizers (Sharma et al., 2023). Physical impurities (glass and plastics), chemical pollutants (pesticides and heavy metals), organic contaminants (dioxin-like polychlorinated biphenyls, dibenzo-p-furans, and dioxins), and biological impurities (plant and animal pathogens) can all be fixed by applying various pretreatment techniques (Sharma et al., 2023). Dewatering, sanitizing, and controlling ammonia levels are required prior to employing AD as a soil amendment, solid compost, and biofertilizer. Enhanced AD efficiency would aid in the production of bio-fertilizers as well, since they are a byproduct of the AD process.
The four main processes that AD uses to break down an organic substrate of agro-waste are hydrolysis, acetogenesis, acidogenesis, and methanogenesis (Mandal et al., 2024; Noor et al., 2024). Larger molecules such as lipids, proteins, nucleotides, and carbohydrates are broken down into smaller molecules such as fatty acids, amino acids, and glucose by the hydrolysis process carried out by a fermentative bacterial community. During acidogenesis, the smaller molecules are then broken down into volatile fatty acid-based compounds (such as butyrate, propionate, and acetate) and various by-products (CO2, NH3, and H2S). Following acidogenesis, the volatile fatty acid components are converted into H2, acetate, and CO2 by the acetogenesis mechanism. Ultimately, acetate is mostly converted to CH4 by methanogens. AD-based biofertilizers have been shown to improve soil quality and increase crop yields (Sharma et al., 2023). In addition, digestate can be used as a soil amendment to improve dissolved organic matter, resulting in rapid and delayed nutrient release and increasing the soil’s ability to retain water (Sharma et al., 2023).
Agro-wastes can be managed by using composting or aerobic digestion techniques, in which microorganisms break down organic materials in an oxygen-rich environment (Noor et al., 2024). The final product is commonly used as a soil amendment. Various methods of composting exist, from backyard compost piles to large-scale operations that decompose thousands of tons of organic waste every year. In composting, three main stages are involved: the mesophilic phase (55 °C for 5-7 days), the thermophilic phase (75 °C for 2-3 days), and the maturation/cooling phase, where a variety of mesophilic and thermophilic microorganisms converge to stabilize and convert organic matter into humus (Noor et al., 2024). As composting progresses, different microorganisms become active and persist in different stages of composting. There are a variety of microbiomes involved in composting, such as fungi, bacteria, actinomycetes, and protozoa, which change depending on the temperature, moisture content, C/N ratio, and organic matter composition (Mandal et al., 2024). Because there are readily available C sources at the beginning, mesophiles carry out the first phase's breakdown of organic substances. During the oxidation of organic material, mesophilic microbes generate heat, which decomposes the bulk of the biodegradable components and enhances the integrity of the organic residue. The rise in temperature renders pathogens and weed seeds inactive. The mesophilic phase is succeeded by the thermophilic phase. During the thermophilic stage, the growth of mesophilic microorganisms will be replaced and regulated by heat-resistant thermophilic microorganisms. Just before compost reaches maturity, the temperature gradually drops and returns to the mesophilic stage because rising temperatures hasten compound breakdown and, consequently, nutrient exhaustion. During the cooling process, the mesophilic microorganisms in the compost become active and break down the remaining organic matter. The organic matter continues to decompose until stable humic compounds are produced (Noor et al., 2024). In order to optimize its fertilizing potential, composting necessitates continuous monitoring of certain parameters such as oxygen content, carbon to nitrogen ratio, pH, particle size, moisture content, curing time, and temperature (Sharma et al., 2023; Mandal et al., 2024).
Figure 1 shows a simple schematic diagram of agro-waste biofertilizer production.
CONCLUSIONS
Agro-wastes are converted into biofertilizers through a series of processes that include collection, pre-treatment, microorganism inoculation, fermentation, maturation, and quality testing. Through these processes, nutrients, microbial populations, and overall quality of the biofertilizer are optimized, ensuring its efficacy and safety. Biofertilizer production with agro wastes not only addresses the problem of waste management, it also promotes sustainable agriculture and economic benefits to farmers. By converting agro-wastes to biofertilizers can reduce the environmental impact associated with waste disposal, minimize the need for chemical fertilizers, and improve soil health in the long run.
Even while the benefits of agro-waste biofertilizer for sustainable agriculture have been acknowledged, it is clear that their application in agronomic practice and industrial production is still far behind expectations. To enable large-scale adoption, mass production, and global marketing, a more clearly defined legal framework is essential. Furthermore, the screening, characterization, and application of innovative agro-waste biofertilizers with the goal of reaching wide adaptation and industrial-scale production are being spurred by developing technologies (e.g., metaomics, encapsulation). However, research and interaction among laboratories, industry, policymakers, and farmers are needed to promote agro-waste biofertilizers as greener alternatives. The culmination of these various contributions ought to be distilled into highly efficient and economically viable bioformulations as a feasible substitute for the chemical fertilizer.
REFERENCES
Agarwal, S., Kumari, S. and Khan, S., 2021. Quality control of biofertilizers. Biofertilizers: Study and Impact, 413-428.
Allouzi, M.M.A., Allouzi, S.M.A., Keng, Z.X., Supramaniam, C.V., Singh, A. and Chong, S., 2022. Liquid biofertilizers as a sustainable solution for agriculture. Heliyon 8: e12609.
Anandham, R., Premalatha, N., Jee, H.J., Weon, H.Y., Kwon, S.W., Krishnamoorthy, R., et al., 2015. Cultivable bacterial diversity and early plant growth promotion by the traditional organic formulations prepared using organic waste materials. Int. J. Recycl. Org. Waste Agric. 4 (4), 279–289.
Bala, S., Garg, D., Sridhar, K., Inbaraj, B.S., Singh, R., Kamma, S., Tripathi, M. and Sharma, M., 2023. Transformation of agro-waste into value-added bioproducts and bioactive compounds: Micro/nano formulations and application in the agri-food-pharma sector. Bioengineering 10: 152.
Barzee, T.J., Edalati, A., El-Mashad, H., Wang, D., Scow, K. and Zhang, R., 2019. Digestate biofertilizers support similar or higher tomato yields and quality than mineral fertilizer in a subsurface drip fertigation system. Front. Sustain. Food Sys. 3: 58.
Chen, C.Y., Mei, H.C., Cheng, C.Y., Lin, J.H., Chung, Y.C., 2012. Enhancing the conversion of organic waste into biofertilizer with thermophilic bacteria. Environ. Eng. Sci. 29 (7), 726–730.
Devi, V. and Sumathy, V.J.H., 2017. Biofertilizer production from agro–wastes. World J. Pharma. Res. 6: 1112-1124.
Gaikwad, A., Jadhav, K. and Nayak, S., 2023. Conversion of lignocellulosic wastes into biofertilizer using bacterial consortium. Curr. Agric. Res. J. 11: 125-136.
Good, A.G. and Beatty, P.H., 2011. Fertilizing nature: a tragedy of excess in the commons. PLoS Biol. 9: e1001124.
Herrmann, L. and Lesueur, D., 2013. Challenges of formulation and quality of biofertilizers for successful inoculation. Appl. Microbiol. Biotechnol. 97: 8859-8873.
Ibanez, A., Garrido-Chamorro, S., Vasco-Cardenas, M.F. and Barreiro, C., 2023. From lab to field: Biofertilizers in the 21st century. Horticulturae, 9: 1306.
Indumathi, D., 2017. Microbial conversion of vegetable wastes for bio fertilizer production. IOSR J. Biotechnol. Biochem. 3 (2), 43–47.
Iocoli, G.A., Zabaloy, M.C., Pasdevicelli, G. and Gómez, M.A., 2019. Use of biogas digestates obtained by anaerobic digestion and co-digestion as fertilizers: Characterization, soil biological activity and growth dynamic of Lactuca sativa L. Sci. Total Environ. 647: 11-19.
Kumar, S., Sindhu, S.S. and Kumar, R., 2022. Biofertilizers: An ecofriendly technology for nutrient recycling and environmental sustainability. Curr. Res. Microb. Sci, 3: 100094.
Lim, S.F. and Matu, S.U., 2015. Utilization of agro-wastes to produce biofertilizer. Int. J. Energy Environ. Eng. 6: 31-35.
Mandal, M., Roy, A., Das, S., Rakwal, R., Agrawal, G.K., Singh, P., Awasthi, A. and Sarkar, A., 2024. Food waste-based bio-fertilizers production by bio-based fermenters and their potential impact on the environment. Chemosphere 353: 141539.
Naeem, U., Afzaal, M., ul Haq, I., Qazi, A., Naeem, A., Mahfooz, Y., 2022. A Comparative Role of Competent Microbes for Value-Added Composting of Agricultural Waste. Research Square Platform LLC.
Noor, R.S., Shah, A.N., Tahir, M.B., Umair, M., Nawaz, M., Ali, A., Ercisli, S., Abdelsalam, N.R., Ali, H.M., Yang, S.H. and Ullah, S., 2024. Recent trends and advances in additive-mediated composting technology for agricultural waste resources: A comprehensive review. ACS Omega, 9: 8632-8653.
Panwar, K.R. and Tripathi, G., 2021. Study of bio-fertilizer produced from agro-waste (Sesame Straw) and cow dung using Eisenia fetida and Perionyx sansibaricus in arid environment. Res. Ecol. 3: 14-20.
Puglia, D., Pezzolla, D., Gigliotti, G., Torre, L., Bartucca, M.L. and Del Buono, D., 2021. The opportunity of valorizing agricultural waste, through its conversion into biostimulants, biofertilizers, and biopolymers. Sustainability 13: 2710.
Qi, G., Pan, Z., Andriamanohiarisoamanana, F.J., Yamashiro, T., Iwasaki, M., Kawamoto, K., Umetsu, K., 2017. Isolation and characterization of plant growth promoting bacteria (PGPB) from anaerobic digestate and their effect on common wheat (Triticum aestivum) seedling growth. Int. J. Environ. Agric. Res. 3 (11), 46–52.
Raymond, F.C., Buraimoh, O.M., Akerele, O.S., Ilori, M.O., Ogundipe, O.T., 2020. Digestate as Biofertilizer for the Growth of Selected Vegetables and Illumina Analysis of Associated Bacterial Community. bioRxiv.
Riseh, R.S., Vazvani, M.G., Hassanisaadi, M. and Thakur, V.K., 2024. Agricultural wastes: a practical and potential source for the isolation and preparation of cellulose and application in agriculture and different industries. Ind. Crops Prod. 208: 117904.
Sadh, P.K., Duhan, S. and Duhan, J.S., 2018. Agro-industrial wastes and their utilization using solid state fermentation: a review. Bioresour. Bioprocess. 5: 1-15.
Sahu, P.K. and Brahmaprakash, G.P., 2016. Formulations of biofertilizers–approaches and advances. Microbial inoculants in sustainable agricultural productivity: Vol. 2: Functional Applications, 179-198.
Saif, S., Abid, Z., Ashiq, M.F., Altaf, M. and Ashraf, R.S., 2021. Biofertilizer formulations. Biofertilizers: Study and Impact, 211-256.
Saini, S., Kuhad, R.C. and Sharma, K.K., 2023. Valorization of rice straw biomass for co-production of bioethanol, biopesticide and biofertilizer following an eco-friendly biorefinery process. Process Saf. Environ. Prot. 173: 823-836.
Sakpirom, J., Nunkaew, T., Khan, E. and Kantachote, D., 2021. Optimization of carriers and packaging for effective biofertilizers to enhance Oryza sativa L. growth in paddy soil. Rhizosphere 19: 100383.
Sartori, R.B., Severo, I.A., de Oliveira, Á.S., Lasta, P., Zepka, L.Q. and Jacob‐Lopes, E., 2021. Biofertilizers from waste. Biofertilizers: study and impact, 517-540.
Sharma, P., Bano, A., Verma, K., Yadav, M., Varjani, S., Singh, S.P. and Tong, Y.W., 2023. Food waste digestate as biofertilizer and their direct applications in agriculture. Biores. Technol. Rep. 23: 101515.
Shyamsundar, P., Springer, N.P., Tallis, H., Polasky, S., Jat, M.L., Sidhu, H.S., Krishnapriya, P.P., Skiba, N., Ginn, W., Ahuja, V. and Cummins, J., 2019. Fields on fire: Alternatives to crop residue burning in India. Science 365: 536-538.