ABSTRACT
Plant disease management is a crucial component during crop production process, and the use of fungicides is a key strategy in current conventional farming practices. In terms of reducing the risk of pathogen drug resistance development and addressing consumer health concerns, the application of beneficial microorganisms demonstrates its advantages in disease control. Among beneficial microorganisms, Bacillus spp. is the most commonly utilized genus. Apart from its growth-promoting abilities in various crops, this microorganism is particularly notable for its excellent disease control characteristics. Due to the diverse mechanisms involved in disease control by Bacillus spp., which mainly include antagonism, disease resistance induction, and colonization, this review will briefly describe these mechanisms and propose ideas for screening multifunctional strains.
Keywords: Agricultural management, biological control, antagonism, induced resistance, colonization
INTRODUCTION
Crop production process will be affected by many factors, and the occurrence of diseases is likely to cause severe damage to the plants, resulting in reduced yield, or even no harvest at all. In order to reduce the occurrence of diseases, the use of fungicides is the most common strategy. At present, many fungicides in the group of FRAC C and G are the most widely used for fungal diseases, such as azoxystrobin and difenoconazole, etc.; while that in the group of FRAC A, D and M are the most important choices for the control of bacterial diseases, such as oxolinic acid, streptomycin and copper-containing agents, etc. (FRAC). In the use of these fungicides, it is necessary to pay attention to the professional use on specific pathogenic pathogen and the risk control to avoid the occurrence of drug resistance on pathogen. However, it is not possible to ensure that all growers have sufficient knowledge in the use, and it is common to use multiple fungicides at the same time in the field to ensure the disease control of crops. Therefore, the emergence of drug-resistant strains and the repeated use of the same mechanism of action have brought difficulties in disease management (da Silva Gündel et al., 2019; de Souza Espindola Santos et al., 2021), respectively. Under this situation, consumers may have concerns about the safety of agricultural products, and thus improving the professionalism of using fungicides in agricultural production is an important way to reduce the use of fungicides. In addition, the development of other materials that can reduce the occurrence of plant diseases is also a feasible solution to reduce the use of fungicides. Among the solutions, the use of microbial agents has gradually become a global trend. Taking some of the world's commercialized microbial agents as an example, the endospores produced by Bacillus bacteria are highly anticipated for their application due to their ability to withstand harsh environmental conditions such as high temperature, low temperature, chemicals, and ultraviolet rays (Nicholson et al., 2000). Although many species within the genus Bacillus may pose risks to human health, there are still many species that fall into categories generally regarded as safe (GRAS), and these could be potential strains for human or plant probiotics
(Azaiez et al., 2018; Cui et al., 2019), black rot (Hsiao et al., 2023; Mishra & Arora, 2012) and bacterial wilt
(Chen et al., 2013; Ho et al., 2020; Peng et al., 2017). Even, B. firmus has been reported to control beet cyst nematode (Huang et al., 2021). It can also be found in above studies that some Bacillus spp. strains also belong to plant growth promoting rhizobacteria (PGPR)
(Elsharkawy et al., 2022; Hsiao et al., 2023). Under the advantages, the occurrence of diseases is not conducive through various interactions on pathogen, plants and space brought by Bacillus spp. strains. For the purpose of applying Bacillus spp. in the control of plant diseases, the functional consideration becomes the decisive factor for the screening method. Therefore, understanding the mechanism of Bacillus spp. in controlling plant diseases is an important topic.
In this review, we will sort out how Bacillus spp. strains utilize the functions of antagonism, induced resistance, and colonization to control diseases, as a reference for agricultural research scientists to screen Bacillus spp. strains in the future.
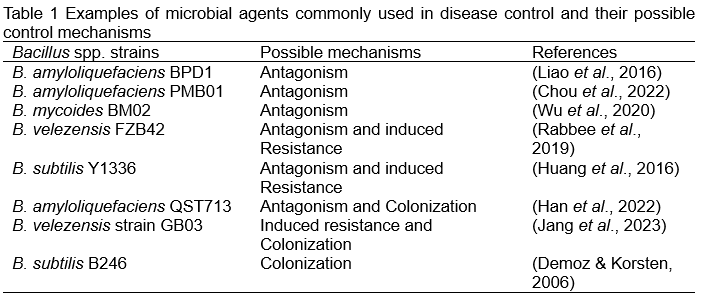
Antagonism of Bacillus spp. against plant pathogens
Currently, the most stable technology and the most important direction to develop microbial agents for controlling plant diseases is screening Bacillus spp. strains that are antagonistic to plant pathogen. This screening method mainly uses the size of the inhibitory zone of Bacillus spp. strains against the specific plant pathogen on the plate as the basis for evaluation. The inhibitory zone occurs due to some of the soluble secondary metabolites produced by Bacillus spp. strains in the medium which may inhibit the growth of pathogen. At present, common secondary metabolites may include volatile substances, polyketides, nonribosomally synthesized peptides, and cyclic lipopeptide compounds, among which cyclic lipopeptide compounds have been studied more
(Fira et al., 2018; Mora et al., 2011; Olishevska et al., 2019). These substances are often destructive to cells or cause cell membrane instability of pathogen to achieve control effects. For example, B. subtilis Z-14 uses fengycin and iturin to destroy the cytoplasm and organelles of Gaeumannomyces graminis (Sacc.) to achieve disease control (Xiao et al., 2021). B. amyloliquefaciens Ba01 is a strain that can produce surfactin, iturin A, and fengycin, and its application can control potato common scab by inhibiting the growth and sporulation of Streptomyces scabies (Lin et al., 2018).
In addition, some studies have shown that some enzymes can also achieve the effect of plant disease control by causing the decomposition of fungal cell walls. For example, the chitinase produced by B. thuringiensis and B. licheniformis has a good inhibitory effect on the growth of a variety of fungal pathogens on soybeans and reduces the reduction in the germination rate of soybeans caused by these pathogens (Gomaa, 2012). The chitinase produced by B. pumilus JUBCH08 causes swelling, vacuolation, rupture and distortion of the mycelium of Fusarium oxysporum (Bhattacharya et al., 2016). This evidence shows that antagonism is indeed an important indicator in the control of plant diseases. Therefore, as shown in Table 1, most of the commercialized microbial agents that have been developed are mainly based on antagonism.
Furthermore, in the process of developing microbial-based products, maintaining antagonistic effects against pathogens in the field to sustain the biocontrol efficacy confirmed in greenhouse experiments is also an important challenge. Currently, fermentation technology may be a viable approach. The results of many studies have demonstrated that adjusting fermentation formulations not only increases the population of Bacillus spp. strains but also enhance their antagonistic effects against pathogens and improves its effectiveness in disease control
(Chou et al., 2022; Sui et al., 2022; Tian et al., 2022). Additionally, using the strain B. amyloliquefaciens PMB04, which possesses antagonistic traits against Xanthomonas perforans, and that it has been shown that adjusting fermentation formulations significantly enhances disease control capabilities. This demonstrates that the application of fermentation broth is equally effective in controlling bacterial leaf spot disease in sweet pepper compared to copper-based fungicides (Wang et al., 2023). In summary, it can be confirmed that the secondary metabolites produced by Bacillus species play a crucial role in antagonizing pathogens and represent an important mechanism in disease control.
Induced resistance activated by Bacillus spp. in plants
There have been many examples to prove that applying some Bacillus spp. strains to control plant diseases is related to the traits on inducing disease resistance in the past decades. In the understanding of induced resistance, the induction process of disease resistance can be divided into two types: systemic acquired resistance (SAR) and induced systemic resistance (ISR). SAR primarily describes the systemic resistance that is induced in plants when they exhibit necrotic disease symptoms in response to pathogen attacks. This resistance is mediated by systemic signaling involving salicylic acid within the host plant, leading to overall disease resistance throughout the plant; ISR, on the other hand, refers to a non-pathogenic response, induced by factors such as chemicals, mechanical damage, or rhizosphere microorganisms, and it activates disease resistance through systemic signaling involving jasmonic acid in the plant. This type of resistance is often referred to as priming response
(Kamle et al., 2020; Pieterse et al., 2009; Yi et al., 2013).
A very clear pattern has been established in the aforementioned literatures. However, some studies on the use of Bacillus spp. for biological control have veered away from this pattern. For example, B. megaterium triggers salicylic acid-dependent resistance to reduce the spikelet rot disease on rice (Cheng et al., 2020). The salicylic acid dependent resistance of tomato can be induced by B. amyloliquefaciens MBI600 to control virus diseases (Beris et al., 2018). B. amyloliquefaciens FZB42 is a PGPR strain that has been successfully commercialized and can be applied to biological control. This strain can simultaneously induce the accumulation of salicylic acid and jasmonic acid and their downstream related genes were upregulated
(Borriss, 2020; Wu et al., 2018). Therefore, we also agree that it may be more appropriate to discuss the signal transduction pathway independently or use "induced resistance" as a general term as suggested by many scholars (Hammerschmidt et al., 2001). In terms of the entire process of inducing disease resistance in plants, the plant immune system proposed by Jones et al. can provide a better explanation on how to initiate disease resistance (Jones & Dangl, 2006). The plant immune system can be divided into two layers of recognition to initiate the plant's defense response
(Jones & Dangl, 2006; Muthamilarasan & Prasad, 2013; Yuan et al., 2021). The first layer of recognition occurs when pathogen approach plant cells. Pathogen-associated molecular patterns (PAMPs) can be recognized by receptors on the surface of plant cells, which further triggers a defense response, called PTI (Bigeard et al., 2015). At this time, if non-pathogenic microorganisms such as Bacillus spp. are approaching or attaching to the plant surface, the secreted Microbe-Associated Molecular Patterns (MAMP) may be recognized by the pattern recognizing receptors (PRRs), the triggered defense responses can be called as MAMP-triggered immunity (MTI) (Newman et al., 2013). The MTI is similar to the aforementioned priming effect. The second layer of identification is mainly after the pathogen invades the cell. In the process of pathogen infection, pathogenic fungi and pathogenic bacteria will secrete effectors into host cells through the use of haustoria and secretion systems (mainly T3SS), respectively. When these effectors are recognized by receptors encoded by resistance genes, it triggers a strong defense response in plants. This response is referred to as Effector-Triggered Immunity (ETI) (Jones & Dangl, 2006; Muthamilarasan & Prasad, 2013). In the context of defense responses, ETI is stronger than PTI and can trigger the occurrence of a hypersensitive response (HR), this concept is similar to the previously mentioned SAR. Additionally, the occurrence of ETI also initiates salicylic acid signaling (Ngou et al., 2022). Therefore, we believe that the concepts of induced resistance and plant immunity are closely related. Induced resistance emphasizes the subsequent disease resistance exhibited by the host plant, while plant immune responses focus more on the process of host plant's recognition to both pathogenic and non-pathogenic microbes. Subsequent disease resistance can be interconnected through two main signaling pathways. In the research involving the use of Bacillus spp. for biological control, the induced disease resistance brought about by MTI (Microbe-Triggered Immunity) is indeed an important mechanism. However, in recent years, a new approach has emerged, with B. amyloliquefaciens PMB05 as its primary representative. When B. amyloliquefaciens PMB05 is applied to plants, this bacterial strain cannot initiate disease resistance through MTI but instead must intensify subsequent disease defense signals through the activation of PTI during the recognition with PAMPs
(Chuang et al., 2022; Ho et al., 2020). We refer to this as PTI intensification. In other words, when this strain resides on the plant's surface, it can only exert its function of promoting plant growth. In addition to B. amyloliquefaciens PMB05, there have been reports indicating that B. cereus AR156 can also utilize the intensification of plant immune signals to control diseases caused by Pseudomonas syringae pv. syringae or Botrytis cinerea
(Jiang et al., 2020; Wang et al., 2018; Yu et al., 2021). Bacillus spp. strains with this capability to intensify plant defense signals significantly when pathogens approach the plant and trigger PTI, thereby boosting the disease resistance. In summary, these studies indicate that the Bacillus spp. strains have a positive impact on the induced resistance in plants. They can enhance plant defense responses through various mechanisms, ultimately reducing the occurrence of diseases.
Colonization of Bacillus spp. in plants
In the screening of Bacillus spp. strains for the control of plant diseases, in addition to the previously mentioned mechanisms related to antagonism and induced resistance, it is also crucial for Bacillus spp. to successfully occupy infection sites that can be targeted by pathogens. This reduces the opportunities for the pathogen to contact with the host. In related studies, there is a greater emphasis on utilizing the successful colonization of Bacillus spp. in the root system to protect plants from soil-borne pathogen attacks
(Samaras et al., 2021; Yuan et al., 2015). The factors determining whether Bacillus spp. can successfully colonize plants are primarily related to chemotaxis and biofilm formation
(Bais et al., 2004; de Weert et al., 2002). Chemotaxis is the process of moving towards a suitable growth environment based on specific chemical substances. In the case of root exudates, they are composed of amino acids, organic acids, phenols, sugars, and proteins, and these substances can attract bacteria to the surface of plant roots
(Bais et al., 2006; de Weert et al., 2002; Sharma et al., 2020).
Regardless of nutritional competition or root colonization, both are the foundation of Bacillus spp. in disease control (Lugtenberg & Kamilova, 2009). Regarding biofilm formation, several studies have demonstrated that the production of organic acids in plant root exudates can promote the attachment of Bacillus spp. strains to plant roots and facilitate biofilm formation
(Gao et al., 2023; Jin et al., 2019; Tan et al., 2013). Several literatures indicate that Bacillus spp. strains capable of forming integral biofilms can indeed enhance disease prevention abilities. For example, Bacillus subtilis N11 can attach to banana roots and effectively reduce the occurrence of banana yellow leaf disease (Zhang et al., 2011). B. amyloliquefaciens SQR 9 can attach to cucumber roots and reduce the incidence of cucumber wilt disease (Cao et al., 2011). Further research on B. amyloliquefaciens SQR 9 in the context of cucumber wilt disease demonstrates that biofilm biosynthesis is indeed related to its root attachment and disease control on host plants (Weng et al., 2013).
According to the literature, the biofilm biosynthesis of Bacillus spp. can be influenced by different nutrient conditions, affecting its characteristics and thickness
(Gallegos-Monterrosa et al., 2016; Zhang et al., 2014). Therefore, in the future application of Bacillus spp., adjusting formulations to enhance their performance in biofilm formation is an important research direction. In addition, aside from inhibiting pathogen colonization in ecological niches through biofilms and lipopeptides, Bacillus spp. also requires the presence of cyclic lipopeptide compounds for biofilm biosynthesis (Al-Ali et al., 2018; Ongena & Jacques, 2008). Furthermore, the production of Bacillus spp. biofilms in the rhizosphere can increase plant tolerance to drought or salt stress
(Kasim et al., 2016; Wang et al., 2019; Yasmeen et al., 2020).
In summary, the colonization of Bacillus spp. strains on plants is a key step in disease control, and the successful attachment of Bacillus spp. strains is primarily related to biofilm formation. Bacillus spp. can improve plant to both biotic and abiotic stresses through the formation of biofilms, highlighting the importance of screening Bacillus spp. strains capable of biofilm formation in the plant rhizosphere.
CONCLUSION
The mainstream approach in commercial development for plant disease control currently involves the use of Bacillus spp. strains. Among the commercially available strains of Bacillus spp., the primary function remains on strains that exhibit antagonistic activity against specific plant pathogens. In most cases, effective disease control is often achieved. However, it still requires individual testing on their effects for different pathogens before disease control. Currently, there are fewer Bacillus spp. products in the market that target mechanisms such as induced resistance or colonization. These mechanisms have the characteristic of being broad-spectrum, potentially effective against various plant diseases. In situations where growers face challenges in selecting the right product, it would be worth considering their development in the market.
As shown in Figure 1, multifunctional Bacillus spp. strains not only pose challenges for producers in terms of product selection but also have the potential to effectively control a wide range of plant diseases. In addition to using GRAS strains as a foundation, it is possible to screen for antagonistic effects against a wide range of crop pathogens and further test their ability to induce resistance and colonization on crops. With this concept in mind, we can anticipate that in the future, the multifunctional screening of strains will become the first step for scientists aiming to use Bacillus spp. for plant disease control.
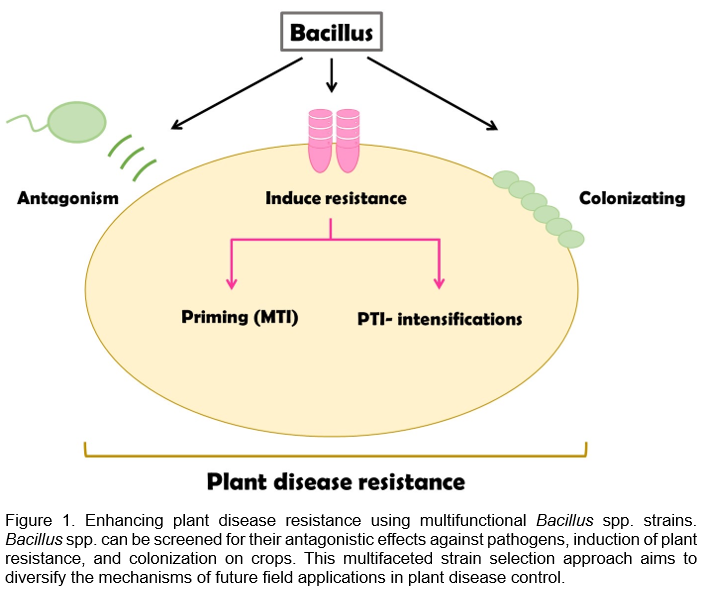
REFERENCES
Ahmad, T., Xing, F., Nie, C., Cao, C., Xiao, Y., Yu, X., Moosa, A., & Liu, Y. (2023). Biocontrol potential of lipopeptides produced by the novel Bacillus subtilis strain Y17B against postharvest Alternaria fruit rot of cherry. Front. Microbiol., 14, 1150217. https://doi.org/doi: 10.3389/fmicb.2023.1150217
Al-Ali, A., Deravel, J., Krier, F., Béchet, M., Ongena, M., & Jacques, P. (2018). Biofilm formation is determinant in tomato rhizosphere colonization by Bacillus velezensis FZB42. Environmental Science and Pollution Research, 25, 29910-29920.
Azaiez, S., Slimene, I. B., Karkouch, I., Essid, R., Jallouli, S., Djebali, N., Elkahoui, S., Limam, F., & Tabbene, O. (2018). Biological control of the soft rot bacterium Pectobacterium carotovorum by Bacillus amyloliquefaciens strain Ar10 producing glycolipid-like compounds. Microbiol. Res., 217, 23-33. https://doi.org/doi: 10.1016/j.micres.2018.08.013
Bais, H. P., Fall, R., & Vivanco, J. M. (2004). Biocontrol of Bacillus subtilis against infection of Arabidopsis roots by Pseudomonas syringae is facilitated by biofilm formation and surfactin production. Plant Physiol., 134, 307-319.
Bais, H. P., Weir, T. L., Perry, L. G., Gilroy, S., & Vivanco, J. M. (2006). The role of root exudates in rhizosphere interactions with plants and other organisms. Annu. Rev. Plant Biol., 57, 233-266.
Beris, D., Theologidis, I., Skandalis, N., & Vassilakos, N. (2018). Bacillus amyloliquefaciens strain MBI600 induces salicylic acid dependent resistance in tomato plants against Tomato spotted wilt virus and Potato virus Y. Scientific Reports, 8(1). https://doi.org/10.1038/s41598-018-28677-3
Bhattacharya, S., Das, A., Samadder, S., & Rajan, S. S. (2016). Biosynthesis and characterization of a thermostable, alkali-tolerant chitinase from Bacillus pumilus JUBCH08 displaying antagonism against phytopathogenic Fusarium oxysporum. 3 Biotech., 6(1). https://doi.org/10.1007/s13205-016-0406-x
Bibi, S., Weis, K., Kaur, A., Bhandari, R., Goss, E. M., Jones, J. B., & Potnis, N. A. (2023). Brief Evaluation of Copper Resistance Mobile Genetic Island in the Bacterial Leaf Spot Pathogen, Xanthomonas euvesicatoria pv. perforans. Phytopathology. https://doi.org/https://doi.org/10.1094/PHYTO-02-23-0077-SC
Bigeard, J., Colcombet, J., & Hirt, H. (2015). Signaling Mechanisms in Pattern-Triggered Immunity (PTI). Mol. Plant, 8, 521-539.
Borriss, R. (2020). Phytostimulation and Biocontrol by the Plant-Associated Bacillus amyloliquefaciens FZB42: An Update. In (pp. 1-20). Springer Singapore. https://doi.org/10.1007/978-981-15-2576-6_1
Cao, Y., Zhang, Z., Ling, N., Yuan, Y., Zheng, X., Shen, B., & Shen, Q. (2011). Bacillus subtilis SQR 9 can control Fusarium wilt in cucumber by colonizing plant roots. Biol. Fertil. Soils, 47, 495-506.
Chen, Y., Yan, F., Chai, Y., Liu, H., Kolter, R., Losick, R., & Guo, J.-H. (2013). Biocontrol of tomato wilt disease by Bacillus subtilis isolates from natural environments depends on conserved genes mediating biofilm formation. Environmental Microbiology, 15(3), 848-864. https://doi.org/10.1111/j.1462-2920.2012.02860.x
Cheng, T., Yao, X.-Z., Wu, C.-Y., Zhang, W., He, W., & Dai, C.-C. (2020). Endophytic Bacillus megaterium triggers salicylic acid-dependent resistance and improves the rhizosphere bacterial community to mitigate rice spikelet rot disease. Appl. Soil Ecol., 156, 103710. https://doi.org/https://doi.org/10.1016/j.apsoil.2020.103710
Chou, H.-P., Huang, Y.-C., Lin, Y.-H., & Deng, W.-L. (2022). Selection, Formulation, and Field Evaluation of Bacillus amyloliquefaciens PMB01 for Its Application to Manage Tomato Bacterial Wilt Disease. Agriculture, 12(10), 1714. https://doi.org/10.3390/agriculture12101714
Chuang, C.-Y., Lin, S.-T., Li, A.-T., Li, S.-H., Hsiao, C.-Y., & Lin, Y.-H. (2022). Bacillus amyloliquefaciens PMB05 Increases Resistance to Bacterial Wilt by Activating Mitogen-Activated Protein Kinase and Reactive Oxygen Species Pathway Crosstalk in Arabidopsis thaliana. Phytopathology, 112, 2495-2502.
Cooksey, D. A. (1994). Molecular mechanisms of copper resistance and accumulation in bacteria. FEMS Microbiol. Rev., 14, 381-386. https://doi.org/110.1111/j.1574-6976.1994.tb00112.x.
Cui, W., He, P., Munir, S., He, P., He, Y., Li, X., Yang, L., Wang, B., Wu, Y., & He, P. (2019). Biocontrol of Soft Rot of Chinese Cabbage Using an Endophytic Bacterial Strain. Front. Microbiol., 10, 1471. https://doi.org/doi: 10.3389/fmicb.2019.01471
da Silva Gündel, S., Dos Reis, T. R., Copetti, P. M., Favarin, F. R., Sagrillo, M. R., da Silva, A. S., Segat, J. C., Baretta, D., & Ourique, A. F. (2019). Evaluation of cytotoxicity, genotoxicity and ecotoxicity of nanoemulsions containing Mancozeb and Eugenol. Ecotoxicol. Environ. Saf., 169, 207-215. https://doi.org/doi:10.1016/j.ecoenv.2018.11.023
de Souza Espindola Santos, A., Parks, C. G., Senna, M. M., de Carvalho, L. V. B., & Meyer, A. (2021). Exposure to pesticides and oxidative stress in Brazilian agricultural communities. Biomarkers, 26, 539-547. https://doi.org/10.1080/1354750X.2021.1933593
de Weert, S., Vermeiren, H., Mulders, I. H., Kuiper, I., Hendrickx, N., Bloemberg, G. V., Vanderleyden, J., de Mot, R., & Lugtenberg, B. J. (2002). Flagella-Driven Chemotaxis Towards Exudate Components Is an Important Trait for Tomato Root Colonization by Pseudomonas fluorescens. . Mol. Plant-Microbe Interact., 15, 1173-1180.
Demoz, B. T., & Korsten, L. (2006). Bacillus subtilis attachment, colonization, and survival on avocado flowers and its mode of action on stem-end rot pathogens. Biological Control, 37(1), 68-74. https://doi.org/https://doi.org/10.1016/j.biocontrol.2005.11.010
Dong, W., Long, T., Ma, J., Wu, N., Mo, W., Zhou, Z., Jin, J., Zhou, H., & Ding, H. (2023). Effects of Bacillus velezensis GUAL210 control on edible rose black spot disease and soil fungal community structure. Front. Microbiol., 14, 1199024. https://doi.org/10.3389/fmicb.2023.1199024
Dorigan, A. F., Moreira, S. I., Ceresini, P. C., Pozza, E. A., Belan, L. L., da Silveira, P. R., & Alves, E. (2022). Higher fitness and competitive advantage of Pyricularia oryzae Triticum lineage resistant to QoI fungicides. Pest Manag. Sci., 78, . https://doi.org/10.1002/ps.7144.
Elshaghabee, F. M. F., Rokana, N., Gulhane, R. D., Sharma, C., & Panwar, H. (2017). Bacillus As Potential Probiotics: Status, Concerns, and Future Perspectives. Front. Microbiol. , 8, 1490. https://doi.org/10.3389/fmicb.2017.01490
Elsharkawy, M. M., Elsawy, M. M., & Ismail, I. A. (2022). Mechanism of resistance to Cucumber mosaic virus elicited by inoculation with Bacillus subtilis subsp. subtilis. Pest Manag. Sci., 78, 86-94. https://doi.org/https://doi.org/10.1002/ps.6610
Fira, D., Dimkić, I., Berić, T., Lozo, J., & Stanković, S. (2018). Biological control of plant pathogens by Bacillus species. J. Biotechnol., 285, 44-55. https://doi.org/https://doi.org/10.1016/j.jbiotec.2018.07.044
FRAC. FRAC Code List ©*2022: Fungal Control Agents Sorted by Cross Resistance Pattern and Mode of Action (Including Coding for FRAC Groups on Product Labels). Retrieved 30, August from https://www.frac.info/docs/default-source/publications/frac-code-list/frac-code-list-2022--final.pdf?sfvrsn=b6024e9a_2
Gallegos-Monterrosa, R., Mhatre, E., & Kovács, Á. T. (2016). Specific Bacillus subtilis 168 variants form biofilms on nutrient-rich medium. Microbiology, 162(11), 1922-1932.
Gao, T., Wang, X., Qin, Y., Ren, Z., & Zhao, X. (2023). Watermelon Root Exudates Enhance Root Colonization of Bacillus amyloliquefaciens TR2. Curr. Microbiol., 80, 110.
Gomaa, E. Z. (2012). Chitinase production by Bacillus thuringiensis and Bacillus licheniformis: Their potential in antifungal biocontrol. J. Microbiol., 50(1), 103-111. https://doi.org/10.1007/s12275-012-1343-y
Hammerschmidt, R., Métraux, J.-P., & Van Loon, L. C. (2001). Inducing Resistance: A Summary of Papers Presented at the First International Symposium on Induced Resistance to Plant Diseases, Corfu, May 2000. Eur. J. Plant Pathol., 107, 1-6.
Han, L., Zhang, M., Du, L., Zhang, L., & Li, B. (2022). Effects of Bacillus amyloliquefaciens QST713 on Photosynthesis and Antioxidant Characteristics of Alfalfa (Medicago sativa L.) under Drought Stress. Agronomy, 12(9), 2177. https://doi.org/10.3390/agronomy12092177
Ho, T.-H., Chuang, C.-Y., Zheng, J.-L., Chen, H.-H., Liang, Y.-S., Huang, T.-P., & Lin, Y.-H. (2020). Bacillus amyloliquefaciens Strain PMB05 Intensifies Plant Immune Responses to Confer Resistance Against Bacterial Wilt of Tomato. Phytopathology, 110(12), 1877-1885. https://doi.org/10.1094/phyto-01-20-0026-r
Hsiao, C.-Y., Blanco, S. D., Peng, A.-L., Fu, J.-Y., Chen, B.-W., Luo, M.-C., Xie, X.-Y., & Lin, Y.-H. (2023). Seed Treatment with Calcium Carbonate Containing Bacillus amyloliquefaciens PMB05 Powder Is an Efficient Way to Control Black Rot Disease of Cabbage. Agriculture, 13(5), 926. https://doi.org/10.3390/agriculture13050926
Huang, C.-N., Lin, C.-P., Hsieh, F.-C., Lee, S.-K., Cheng, K.-C., & Liu, C.-T. (2016). Characterization and evaluation of Bacillus amyloliquefaciens strain WF02 regarding its biocontrol activities and genetic responses against bacterial wilt in two different resistant tomato cultivars. World J. Microbiol. Biotechnol., 32(11). https://doi.org/10.1007/s11274-016-2143-z
Huang, M., Bulut, A., Shrestha, B., Matera, C., Grundler, F. M. W., & Schleker, A. S. S. (2021). Bacillus firmus I-1582 promotes plant growth and impairs infection and development of the cyst nematode Heterodera schachtii over two generations. Sci. Rep., 11, 14114. https://doi.org/doi: 10.1038/s41598-021-93567-0
Jang, S., Choi, S. K., Zhang, H., Zhang, S., Ryu, C. M., & Kloepper, J. W. (2023). History of a model plant growth-promoting rhizobacterium, Bacillus velezensis GB03: from isolation to commercialization. Front Plant Sci, 14, 1279896. https://doi.org/10.3389/fpls.2023.1279896
Jiang, C., Fan, Z., Li, Z., Niu, D., Li, Y., Zheng, M., Wang, Q., Jin, H., & Guo, J. (2020). Bacillus cereus AR156 triggers induced systemic resistance against Pseudomonas syringae pv. tomato DC3000 by suppressing miR472 and activating CNLs‐mediated basal immunity in Arabidopsis. Mol. Plant Pathol., 21(6), 854-870. https://doi.org/10.1111/mpp.12935
Jin, Y., Zhu, H., Luo, S., Yang, W., Zhang, L., Li, S., Jin, Q., Cao, Q., Sun, S., & Xiao, M. (2019). Role of Maize Root Exudates in Promotion of Colonization of Bacillus velezensis Strain S3-1 in Rhizosphere Soil and Root Tissue. . Curr. Microbiol., 76, 855-862.
Jones, J. D. G., & Dangl, J. L. (2006). The plant immune system. Nature, 444, 323-329.
Kamle, M., Borah, R., Bora, H., Jaiswal, A. K., Singh, R. K., & Kumar, P. (2020). Systemic Acquired Resistance (SAR) and Induced Systemic Resistance (ISR): Role and Mechanism of Action Against Phytopathogens. In (pp. 457-470). Springer International Publishing. https://doi.org/10.1007/978-3-030-41870-0_20
Kasim, W. A., Gaafar, R. M., Abou-Ali, R. M., Omar, M. N., & Hewait, H. M. (2016). Effect of biofilm forming plant growth promoting rhizobacteria on salinity tolerance in barley. Ann. Agric. Sci., 61(2), 217-227.
Leroux, P., Fritz, R., Debieu, D., Albertini, C., Lanen, C., Bach, J., Gredt, M., & Chapeland, F. (2002). Mechanisms of resistance to fungicides in field strains of Botrytis cinerea. Pest Manag. Sci., 58, 876-888.
Liang, Y.-S., Fu, J.-Y., Chao, S.-H., Tzean, Y., Hsiao, C.-Y., Yang, Y.-Y., Chen, Y.-K., & Lin, Y.-H. (2022). Postharvest Application of Bacillus amyloliquefaciens PMB04 Fermentation Broth Reduces Anthracnose Occurrence in Mango Fruit. Agriculture, 12(10), 1646. https://doi.org/10.3390/agriculture12101646
Liao, J.-H., Chen, P.-Y., Yang, Y.-L., Kan, S.-C., Hsieh, F.-C., & Liu, Y.-C. (2016). Clarification of the Antagonistic Effect of the Lipopeptides Produced by Bacillus amyloliquefaciens BPD1 against Pyricularia oryzae via In Situ MALDI-TOF IMS Analysis. Molecules, 21(12), 1670. https://doi.org/10.3390/molecules21121670
Lin, C., Tsai, C.-H., Chen, P.-Y., Wu, C.-Y., Chang, Y.-L., Yang, Y.-L., & Chen, Y.-L. (2018). Biological control of potato common scab by Bacillus amyloliquefaciens Ba01. PLOS ONE, 13(4), e0196520. https://doi.org/10.1371/journal.pone.0196520
Lugtenberg, B., & Kamilova, F. (2009). Plant-growth-promoting rhizobacteria. Annu. Rev. Microbiol., 63, 541-556.
Mishra, S., & Arora, N. K. (2012). Evaluation of rhizospheric Pseudomonas and Bacillus as biocontrol tool for Xanthomonas campestris pv campestris. World J. Microbiol. Biotechnol., 28, 693-702. https://doi.org/doi: 10.1007/s11274-011-0865-5.
Mora, I., Cabrefiga, J., & Montesinos, E. (2011). Antimicrobial peptide genes in Bacillus strains from plant environments. Int. Microbiol., 14, 213-223. https://doi.org/doi: 10.2436/20.1501.01.151.
Muthamilarasan, M., & Prasad, M. (2013). Plant innate immunity: An updated insight into defense mechanism. J. Biosci., 38, 433-449.
Newman, M.-A., Sundelin, T., Nielsen, J. T., & Erbs, G. (2013). MAMP (microbe-associated molecular pattern) triggered immunity in plants. Front. Plant Sci., 4, 139. https://doi.org/doi: 10.3389/fpls.2013.00139
Ngou, B. P. M., Jones, J. D. G., & Ding, P. (2022). Plant immune networks. Trends Plant Sci., 27(3), 255-273. https://doi.org/10.1016/j.tplants.2021.08.012
Nicholson, W. L., Munakata, N., Horneck, G., Melosh, H. J., & Setlow, P. (2000). Resistance of Bacillus Endospores to Extreme Terrestrial and Extraterrestrial Environments. Microbiol. Mol. Biol. Rev., 64, 548–572.
Olishevska, S., Nickzad, A., & Déziel, E. (2019). Bacillus and Paenibacillus secreted polyketides and peptides involved in controlling human and plant pathogens. Appl. Microbiol. Biotechnol., 103(3), 1189-1215. https://doi.org/10.1007/s00253-018-9541-0
Ongena, M., & Jacques, P. (2008). Bacillus lipopeptides: versatile weapons for plant disease biocontrol. Trends in Microbiology, 16(3), 115-125. https://doi.org/https://doi.org/10.1016/j.tim.2007.12.009
Pang, L., Xia, B., Liu, X., Yi, Y., Jiang, L., Chan, C., Li, P., Zhang, M., Deng, X., & Wang, R. (2021). Improvement of antifungal activity of a culture filtrate of endophytic Bacillus amyloliquefaciens isolated from kiwifruit and its effect on postharvest quality of kiwifruit. J Food Biochem., 45, e13551. https://doi.org/ https://doi.org/10.1111/jfbc.13551
Peng, D., Luo, K., Jiang, H., Deng, Y., Bai, L., & Zhou, X. (2017). Combined use of Bacillus subtilis strain B-001 and bactericide for the control of tomato bacterial wilt. Pest Manag. Sci., 73, 1253-1257. https://doi.org/doi: 10.1002/ps.4453.
Pieterse, C. M. J., Leon-Reyes, A., Van der Ent, S., & Van Wees, S. C. M. (2009). Networking by small-molecule hormones in plant immunity. Nature Chem. Biol., 5, 308-316.
Rabbee, M., Ali, M., Choi, J., Hwang, B., Jeong, S., & Baek, K.-H. (2019). Bacillus velezensis: A Valuable Member of Bioactive Molecules within Plant Microbiomes. Molecules, 24(6), 1046. https://doi.org/10.3390/molecules24061046
Radhakrishnan, R., Hashem, A., & Abd Allah, E. F. (2017). Bacillus: A Biological Tool for Crop Improvement through Bio-Molecular Changes in Adverse Environments. Front. Physiol., 8, 667. https://doi.org/doi: 10.3389/fphys.2017.00667
Rendina, N., Nuzzaci, M., Sofo, A., Campiglia, P., Scopa, A., Sommella, E., Pepe, G., De Nisco, M., Basilicata, M. G., & Manfra, M. (2019). Yield parameters and antioxidant compounds of tomato fruit: the role of plant defence inducers with or without Cucumber mosaic virus infection. J. Sci. Food Agri., 99(12), 5541-5549. https://doi.org/10.1002/jsfa.9818
Ribas E. Ribas, A. D., Spolti, P., Del Ponte, E. M., Donato, K. Z., Schrekker, H., & Fuentefria, A. M. (2016). Is the emergence of fungal resistance to medical triazoles related to their use in the agroecosystems? A mini review. Braz. J. Microbiol., 47, 793-799. https://doi.org/10.1016/j.bjm.2016.06.006
Rivelli Zea, S. M., & Toyotome, T. (2022). Azole-resistant Aspergillus fumigatus as an emerging worldwide pathogen. Microbiol. Immunol., 66, 135-144. https://doi.org/10.1111/1348-0421.12957.
Samaras, A., Nikolaidis, M., Antequera-Gómez, M. L., Cámara-Almirón, J., Romero, D., Moschakis, T., Amoutzias, G. D., & Karaoglanidis, G. S. (2021). Whole genome sequencing and root colonization studies reveal novel insights in the biocontrol potential and growth promotion by Bacillus subtilis MBI 600 on cucumber. Frontiers in Microbiology, 11, 600393.
Sharma, M., Saleh, D., Charron, J. B., & Jabaji, S. (2020). A crosstalk between Brachypodium root exudates, organic acids, and Bacillus velezensis B26, a growth promoting bacterium. Front. Microbiol., 11, 575578.
Sui, X., Han, X., Cao, J., Li, Y., Yuan, Y., Gou, J., Zheng, Y., Meng, C., & Zhang, C. (2022). Biocontrol potential of Bacillus velezensis EM-1 associated with suppressive rhizosphere soil microbes against tobacco bacterial wilt. Front. Microbiol., 13, 940156. https://doi.org/doi: 10.3389/fmicb.2022.940156
Tan, S., Yang, C., Mei, X., Shen, S., Raza, W., Shen, Q., & Xu, Y. (2013). The effect of organic acids from tomato root exudates on rhizosphere colonization of Bacillus amyloliquefaciens T-5. Appl. Soil Ecol., 64, 15-22.
Tian, X.-l., Zhao, X.-M., Zhao, S.-Y., Zhao, J.-l., & Mao, Z.-C. (2022). The Biocontrol Functions of Bacillus velezensis Strain Bv-25 Against Meloidogyne incognita. Front. Microbiol., 13, 843041. https://doi.org/ doi: 10.3389/fmicb.2022.843041
Wang, D.-C., Jiang, C.-H., Zhang, L.-N., Chen, L., Zhang, X.-Y., & Guo, J.-H. (2019). Biofilms Positively Contribute to Bacillus amyloliquefaciens 54-induced Drought Tolerance in Tomato Plants. Intl. J. Mol. Sci., 20(24), 6271. https://www.mdpi.com/1422-0067/20/24/6271
Wang, F., Chao, S.-H., Tsai, C.-H., Blanco, S. D., Yang, Y.-Y., & Lin, Y.-H. (2023). Developing Fermentation Liquid of Bacillus amyloliquefaciens PMB04 to Control Bacterial Leaf Spot of Sweet Pepper. Agriculture, 13(7), 1456. https://doi.org/10.3390/agriculture13071456
Wang, S., Zheng, Y., Gu, C., He, C., Yang, M., Zhang, X., Guo, J., Zhao, H., & Niu, D. (2018). Bacillus cereus AR156 Activates Defense Responses to Pseudomonas syringae pv. tomato in Arabidopsis thaliana Similarly to flg22. Mol. Plant-Microbe Interact., 31(3), 311-322. https://doi.org/10.1094/mpmi-10-17-0240-r
Weng, J., Wang, Y., Li, J., Shen, Q., & Zhang, R. (2013). Enhanced root colonization and biocontrol activity of Bacillus amyloliquefaciens SQR9 by abrB gene disruption. Appl. Microbiol. Biotechnol., 97, 8823-8830.
Wu, J.-J., Huang, J.-W., & Deng, W.-L. (2020). Phenylacetic Acid and Methylphenyl Acetate From the Biocontrol Bacterium Bacillus mycoides BM02 Suppress Spore Germination in Fusarium oxysporum f. sp. lycopersici. Front. Microbiol., 11, 569263. https://doi.org/10.3389/fmicb.2020.569263
Wu, L., Huang, Z., Li, X., Ma, L., Gu, Q., Wu, H., Liu, J., Borriss, R., Wu, Z., & Gao, X. (2018). Stomatal Closure and SA-, JA/ET-Signaling Pathways Are Essential for Bacillus amyloliquefaciens FZB42 to Restrict Leaf Disease Caused by Phytophthora nicotianae in Nicotiana benthamiana. Front. Microbiol., 9, 847. https://doi.org/doi: 10.3389/fmicb.2018.00847
Xiao, J., Guo, X., Qiao, X., Zhang, X., Chen, X., & Zhang, D. (2021). Activity of Fengycin and Iturin A Isolated From Bacillus subtilis Z-14 on Gaeumannomyces graminis Var. tritici and Soil Microbial Diversity. Front. Microbiol., 12, 682437. https://doi.org/doi: 10.3389/fmicb.2021.682437
Yasmeen, T., Ahmad, A., Arif, M. S., Mubin, M., Rehman, K., Shahzad, S. M., Iqbal, S., Rizwan, M., Ali, S., Alyemeni, M. N., & Wijaya, L. (2020). Biofilm forming rhizobacteria enhance growth and salt tolerance in sunflower plants by stimulating antioxidant enzymes activity. Plant Physiol. Biochem., 156, 242-256. https://doi.org/https://doi.org/10.1016/j.plaphy.2020.09.016
Yi, H.-S., Yang, J.-W., & Ryu, C.-M. (2013). ISR meets SAR outside: additive action of the endophyte Bacillus pumilus INR7 and the chemical inducer, benzothiadiazole, on induced resistance against bacterial spot in field-grown pepper. Frontiers in Plant Science, 4.
Yu, Y.-Y., Dou, G.-X., Sun, X.-X., Chen, L., Zheng, Y., Xiao, H.-M., Wang, Y.-P., Li, H.-Y., Guo, J.-H., & Jiang, C.-H. (2021). Transcriptome and Biochemical Analysis Jointly Reveal the Effects of Bacillus cereus AR156 on Postharvest Strawberry Gray Mold and Fruit Quality. Front. Plant Sci., 12, 700446. https://doi.org/doi: 10.3389/fpls.2021.700446
Yuan, J., Zhang, N., Huang, Q., Raza, W., Li, R., Vivanco, J. M., & Shen, Q. (2015). Organic acids from root exudates of banana help root colonization of PGPR strain Bacillus amyloliquefaciens NJN-6. Sci. Rep., 5, 13438.
Yuan, M., Ngou, B.-P.-M., Ding, P., & Xin, F.-X. (2021). PTI-ETI crosstalk: an integrative view of plant immunity. Curr. Opin. Plant Biol., 62.
Zhang, N., Wu, K., He, X., Li, S. Q., Zhang, Z. H., Shen, B., Yang, X. M., Zhang, R. F., Huang, Q. W., & Shen, Q. R. (2011). A new bioorganic fertilizer can effectively control banana wilt by strong colonization with Bacillus subtilis N11. Plant Soil, 344, 87-97.
Zhang, S., Zheng, Q., Xu, B., & Liu, J. (2019). Identification of the Fungal Pathogens of Postharvest Disease on Peach Fruits and the Control Mechanisms of Bacillus subtilis JK-14. Toxins, 11(6), 322. https://doi.org/10.3390/toxins11060322
Zhang, W., Seminara, A., Suaris, M., Brenner, M. P., Weitz, D. A., & Angelini, T. E. (2014). Nutrient depletion in Bacillus subtilis biofilms triggers matrix production. New J. Phys., 16(1), 015028.
Zhou, Q., Fu, M., Xu, M., Chen, X., Qiu, J., Wang, F., Yan, R., Wang, J., Zhao, S., Xin, X., & Chen, L. (2020). Application of antagonist Bacillus amyloliquefaciens NCPSJ7 against Botrytis cinerea in postharvest Red Globe grapes. Food Sci. Nutr., 8(3), 1499-1508. https://doi.org/10.1002/fsn3.1434
Bacillus spp. Control Plant Diseases Through Multiple Mechanisms
ABSTRACT
Plant disease management is a crucial component during crop production process, and the use of fungicides is a key strategy in current conventional farming practices. In terms of reducing the risk of pathogen drug resistance development and addressing consumer health concerns, the application of beneficial microorganisms demonstrates its advantages in disease control. Among beneficial microorganisms, Bacillus spp. is the most commonly utilized genus. Apart from its growth-promoting abilities in various crops, this microorganism is particularly notable for its excellent disease control characteristics. Due to the diverse mechanisms involved in disease control by Bacillus spp., which mainly include antagonism, disease resistance induction, and colonization, this review will briefly describe these mechanisms and propose ideas for screening multifunctional strains.
Keywords: Agricultural management, biological control, antagonism, induced resistance, colonization
INTRODUCTION
Crop production process will be affected by many factors, and the occurrence of diseases is likely to cause severe damage to the plants, resulting in reduced yield, or even no harvest at all. In order to reduce the occurrence of diseases, the use of fungicides is the most common strategy. At present, many fungicides in the group of FRAC C and G are the most widely used for fungal diseases, such as azoxystrobin and difenoconazole, etc.; while that in the group of FRAC A, D and M are the most important choices for the control of bacterial diseases, such as oxolinic acid, streptomycin and copper-containing agents, etc. (FRAC). In the use of these fungicides, it is necessary to pay attention to the professional use on specific pathogenic pathogen and the risk control to avoid the occurrence of drug resistance on pathogen. However, it is not possible to ensure that all growers have sufficient knowledge in the use, and it is common to use multiple fungicides at the same time in the field to ensure the disease control of crops. Therefore, the emergence of drug-resistant strains and the repeated use of the same mechanism of action have brought difficulties in disease management (da Silva Gündel et al., 2019; de Souza Espindola Santos et al., 2021), respectively. Under this situation, consumers may have concerns about the safety of agricultural products, and thus improving the professionalism of using fungicides in agricultural production is an important way to reduce the use of fungicides. In addition, the development of other materials that can reduce the occurrence of plant diseases is also a feasible solution to reduce the use of fungicides. Among the solutions, the use of microbial agents has gradually become a global trend. Taking some of the world's commercialized microbial agents as an example, the endospores produced by Bacillus bacteria are highly anticipated for their application due to their ability to withstand harsh environmental conditions such as high temperature, low temperature, chemicals, and ultraviolet rays (Nicholson et al., 2000). Although many species within the genus Bacillus may pose risks to human health, there are still many species that fall into categories generally regarded as safe (GRAS), and these could be potential strains for human or plant probiotics
(Azaiez et al., 2018; Cui et al., 2019), black rot (Hsiao et al., 2023; Mishra & Arora, 2012) and bacterial wilt
(Chen et al., 2013; Ho et al., 2020; Peng et al., 2017). Even, B. firmus has been reported to control beet cyst nematode (Huang et al., 2021). It can also be found in above studies that some Bacillus spp. strains also belong to plant growth promoting rhizobacteria (PGPR)
(Elsharkawy et al., 2022; Hsiao et al., 2023). Under the advantages, the occurrence of diseases is not conducive through various interactions on pathogen, plants and space brought by Bacillus spp. strains. For the purpose of applying Bacillus spp. in the control of plant diseases, the functional consideration becomes the decisive factor for the screening method. Therefore, understanding the mechanism of Bacillus spp. in controlling plant diseases is an important topic.
In this review, we will sort out how Bacillus spp. strains utilize the functions of antagonism, induced resistance, and colonization to control diseases, as a reference for agricultural research scientists to screen Bacillus spp. strains in the future.
Antagonism of Bacillus spp. against plant pathogens
Currently, the most stable technology and the most important direction to develop microbial agents for controlling plant diseases is screening Bacillus spp. strains that are antagonistic to plant pathogen. This screening method mainly uses the size of the inhibitory zone of Bacillus spp. strains against the specific plant pathogen on the plate as the basis for evaluation. The inhibitory zone occurs due to some of the soluble secondary metabolites produced by Bacillus spp. strains in the medium which may inhibit the growth of pathogen. At present, common secondary metabolites may include volatile substances, polyketides, nonribosomally synthesized peptides, and cyclic lipopeptide compounds, among which cyclic lipopeptide compounds have been studied more
(Fira et al., 2018; Mora et al., 2011; Olishevska et al., 2019). These substances are often destructive to cells or cause cell membrane instability of pathogen to achieve control effects. For example, B. subtilis Z-14 uses fengycin and iturin to destroy the cytoplasm and organelles of Gaeumannomyces graminis (Sacc.) to achieve disease control (Xiao et al., 2021). B. amyloliquefaciens Ba01 is a strain that can produce surfactin, iturin A, and fengycin, and its application can control potato common scab by inhibiting the growth and sporulation of Streptomyces scabies (Lin et al., 2018).
In addition, some studies have shown that some enzymes can also achieve the effect of plant disease control by causing the decomposition of fungal cell walls. For example, the chitinase produced by B. thuringiensis and B. licheniformis has a good inhibitory effect on the growth of a variety of fungal pathogens on soybeans and reduces the reduction in the germination rate of soybeans caused by these pathogens (Gomaa, 2012). The chitinase produced by B. pumilus JUBCH08 causes swelling, vacuolation, rupture and distortion of the mycelium of Fusarium oxysporum (Bhattacharya et al., 2016). This evidence shows that antagonism is indeed an important indicator in the control of plant diseases. Therefore, as shown in Table 1, most of the commercialized microbial agents that have been developed are mainly based on antagonism.
Furthermore, in the process of developing microbial-based products, maintaining antagonistic effects against pathogens in the field to sustain the biocontrol efficacy confirmed in greenhouse experiments is also an important challenge. Currently, fermentation technology may be a viable approach. The results of many studies have demonstrated that adjusting fermentation formulations not only increases the population of Bacillus spp. strains but also enhance their antagonistic effects against pathogens and improves its effectiveness in disease control
(Chou et al., 2022; Sui et al., 2022; Tian et al., 2022). Additionally, using the strain B. amyloliquefaciens PMB04, which possesses antagonistic traits against Xanthomonas perforans, and that it has been shown that adjusting fermentation formulations significantly enhances disease control capabilities. This demonstrates that the application of fermentation broth is equally effective in controlling bacterial leaf spot disease in sweet pepper compared to copper-based fungicides (Wang et al., 2023). In summary, it can be confirmed that the secondary metabolites produced by Bacillus species play a crucial role in antagonizing pathogens and represent an important mechanism in disease control.
Induced resistance activated by Bacillus spp. in plants
There have been many examples to prove that applying some Bacillus spp. strains to control plant diseases is related to the traits on inducing disease resistance in the past decades. In the understanding of induced resistance, the induction process of disease resistance can be divided into two types: systemic acquired resistance (SAR) and induced systemic resistance (ISR). SAR primarily describes the systemic resistance that is induced in plants when they exhibit necrotic disease symptoms in response to pathogen attacks. This resistance is mediated by systemic signaling involving salicylic acid within the host plant, leading to overall disease resistance throughout the plant; ISR, on the other hand, refers to a non-pathogenic response, induced by factors such as chemicals, mechanical damage, or rhizosphere microorganisms, and it activates disease resistance through systemic signaling involving jasmonic acid in the plant. This type of resistance is often referred to as priming response
(Kamle et al., 2020; Pieterse et al., 2009; Yi et al., 2013).
A very clear pattern has been established in the aforementioned literatures. However, some studies on the use of Bacillus spp. for biological control have veered away from this pattern. For example, B. megaterium triggers salicylic acid-dependent resistance to reduce the spikelet rot disease on rice (Cheng et al., 2020). The salicylic acid dependent resistance of tomato can be induced by B. amyloliquefaciens MBI600 to control virus diseases (Beris et al., 2018). B. amyloliquefaciens FZB42 is a PGPR strain that has been successfully commercialized and can be applied to biological control. This strain can simultaneously induce the accumulation of salicylic acid and jasmonic acid and their downstream related genes were upregulated
(Borriss, 2020; Wu et al., 2018). Therefore, we also agree that it may be more appropriate to discuss the signal transduction pathway independently or use "induced resistance" as a general term as suggested by many scholars (Hammerschmidt et al., 2001). In terms of the entire process of inducing disease resistance in plants, the plant immune system proposed by Jones et al. can provide a better explanation on how to initiate disease resistance (Jones & Dangl, 2006). The plant immune system can be divided into two layers of recognition to initiate the plant's defense response
(Jones & Dangl, 2006; Muthamilarasan & Prasad, 2013; Yuan et al., 2021). The first layer of recognition occurs when pathogen approach plant cells. Pathogen-associated molecular patterns (PAMPs) can be recognized by receptors on the surface of plant cells, which further triggers a defense response, called PTI (Bigeard et al., 2015). At this time, if non-pathogenic microorganisms such as Bacillus spp. are approaching or attaching to the plant surface, the secreted Microbe-Associated Molecular Patterns (MAMP) may be recognized by the pattern recognizing receptors (PRRs), the triggered defense responses can be called as MAMP-triggered immunity (MTI) (Newman et al., 2013). The MTI is similar to the aforementioned priming effect. The second layer of identification is mainly after the pathogen invades the cell. In the process of pathogen infection, pathogenic fungi and pathogenic bacteria will secrete effectors into host cells through the use of haustoria and secretion systems (mainly T3SS), respectively. When these effectors are recognized by receptors encoded by resistance genes, it triggers a strong defense response in plants. This response is referred to as Effector-Triggered Immunity (ETI) (Jones & Dangl, 2006; Muthamilarasan & Prasad, 2013). In the context of defense responses, ETI is stronger than PTI and can trigger the occurrence of a hypersensitive response (HR), this concept is similar to the previously mentioned SAR. Additionally, the occurrence of ETI also initiates salicylic acid signaling (Ngou et al., 2022). Therefore, we believe that the concepts of induced resistance and plant immunity are closely related. Induced resistance emphasizes the subsequent disease resistance exhibited by the host plant, while plant immune responses focus more on the process of host plant's recognition to both pathogenic and non-pathogenic microbes. Subsequent disease resistance can be interconnected through two main signaling pathways. In the research involving the use of Bacillus spp. for biological control, the induced disease resistance brought about by MTI (Microbe-Triggered Immunity) is indeed an important mechanism. However, in recent years, a new approach has emerged, with B. amyloliquefaciens PMB05 as its primary representative. When B. amyloliquefaciens PMB05 is applied to plants, this bacterial strain cannot initiate disease resistance through MTI but instead must intensify subsequent disease defense signals through the activation of PTI during the recognition with PAMPs
(Chuang et al., 2022; Ho et al., 2020). We refer to this as PTI intensification. In other words, when this strain resides on the plant's surface, it can only exert its function of promoting plant growth. In addition to B. amyloliquefaciens PMB05, there have been reports indicating that B. cereus AR156 can also utilize the intensification of plant immune signals to control diseases caused by Pseudomonas syringae pv. syringae or Botrytis cinerea
(Jiang et al., 2020; Wang et al., 2018; Yu et al., 2021). Bacillus spp. strains with this capability to intensify plant defense signals significantly when pathogens approach the plant and trigger PTI, thereby boosting the disease resistance. In summary, these studies indicate that the Bacillus spp. strains have a positive impact on the induced resistance in plants. They can enhance plant defense responses through various mechanisms, ultimately reducing the occurrence of diseases.
Colonization of Bacillus spp. in plants
In the screening of Bacillus spp. strains for the control of plant diseases, in addition to the previously mentioned mechanisms related to antagonism and induced resistance, it is also crucial for Bacillus spp. to successfully occupy infection sites that can be targeted by pathogens. This reduces the opportunities for the pathogen to contact with the host. In related studies, there is a greater emphasis on utilizing the successful colonization of Bacillus spp. in the root system to protect plants from soil-borne pathogen attacks
(Samaras et al., 2021; Yuan et al., 2015). The factors determining whether Bacillus spp. can successfully colonize plants are primarily related to chemotaxis and biofilm formation
(Bais et al., 2004; de Weert et al., 2002). Chemotaxis is the process of moving towards a suitable growth environment based on specific chemical substances. In the case of root exudates, they are composed of amino acids, organic acids, phenols, sugars, and proteins, and these substances can attract bacteria to the surface of plant roots
(Bais et al., 2006; de Weert et al., 2002; Sharma et al., 2020).
Regardless of nutritional competition or root colonization, both are the foundation of Bacillus spp. in disease control (Lugtenberg & Kamilova, 2009). Regarding biofilm formation, several studies have demonstrated that the production of organic acids in plant root exudates can promote the attachment of Bacillus spp. strains to plant roots and facilitate biofilm formation
(Gao et al., 2023; Jin et al., 2019; Tan et al., 2013). Several literatures indicate that Bacillus spp. strains capable of forming integral biofilms can indeed enhance disease prevention abilities. For example, Bacillus subtilis N11 can attach to banana roots and effectively reduce the occurrence of banana yellow leaf disease (Zhang et al., 2011). B. amyloliquefaciens SQR 9 can attach to cucumber roots and reduce the incidence of cucumber wilt disease (Cao et al., 2011). Further research on B. amyloliquefaciens SQR 9 in the context of cucumber wilt disease demonstrates that biofilm biosynthesis is indeed related to its root attachment and disease control on host plants (Weng et al., 2013).
According to the literature, the biofilm biosynthesis of Bacillus spp. can be influenced by different nutrient conditions, affecting its characteristics and thickness
(Gallegos-Monterrosa et al., 2016; Zhang et al., 2014). Therefore, in the future application of Bacillus spp., adjusting formulations to enhance their performance in biofilm formation is an important research direction. In addition, aside from inhibiting pathogen colonization in ecological niches through biofilms and lipopeptides, Bacillus spp. also requires the presence of cyclic lipopeptide compounds for biofilm biosynthesis (Al-Ali et al., 2018; Ongena & Jacques, 2008). Furthermore, the production of Bacillus spp. biofilms in the rhizosphere can increase plant tolerance to drought or salt stress
(Kasim et al., 2016; Wang et al., 2019; Yasmeen et al., 2020).
In summary, the colonization of Bacillus spp. strains on plants is a key step in disease control, and the successful attachment of Bacillus spp. strains is primarily related to biofilm formation. Bacillus spp. can improve plant to both biotic and abiotic stresses through the formation of biofilms, highlighting the importance of screening Bacillus spp. strains capable of biofilm formation in the plant rhizosphere.
CONCLUSION
The mainstream approach in commercial development for plant disease control currently involves the use of Bacillus spp. strains. Among the commercially available strains of Bacillus spp., the primary function remains on strains that exhibit antagonistic activity against specific plant pathogens. In most cases, effective disease control is often achieved. However, it still requires individual testing on their effects for different pathogens before disease control. Currently, there are fewer Bacillus spp. products in the market that target mechanisms such as induced resistance or colonization. These mechanisms have the characteristic of being broad-spectrum, potentially effective against various plant diseases. In situations where growers face challenges in selecting the right product, it would be worth considering their development in the market.
As shown in Figure 1, multifunctional Bacillus spp. strains not only pose challenges for producers in terms of product selection but also have the potential to effectively control a wide range of plant diseases. In addition to using GRAS strains as a foundation, it is possible to screen for antagonistic effects against a wide range of crop pathogens and further test their ability to induce resistance and colonization on crops. With this concept in mind, we can anticipate that in the future, the multifunctional screening of strains will become the first step for scientists aiming to use Bacillus spp. for plant disease control.
REFERENCES
Ahmad, T., Xing, F., Nie, C., Cao, C., Xiao, Y., Yu, X., Moosa, A., & Liu, Y. (2023). Biocontrol potential of lipopeptides produced by the novel Bacillus subtilis strain Y17B against postharvest Alternaria fruit rot of cherry. Front. Microbiol., 14, 1150217. https://doi.org/doi: 10.3389/fmicb.2023.1150217
Al-Ali, A., Deravel, J., Krier, F., Béchet, M., Ongena, M., & Jacques, P. (2018). Biofilm formation is determinant in tomato rhizosphere colonization by Bacillus velezensis FZB42. Environmental Science and Pollution Research, 25, 29910-29920.
Azaiez, S., Slimene, I. B., Karkouch, I., Essid, R., Jallouli, S., Djebali, N., Elkahoui, S., Limam, F., & Tabbene, O. (2018). Biological control of the soft rot bacterium Pectobacterium carotovorum by Bacillus amyloliquefaciens strain Ar10 producing glycolipid-like compounds. Microbiol. Res., 217, 23-33. https://doi.org/doi: 10.1016/j.micres.2018.08.013
Bais, H. P., Fall, R., & Vivanco, J. M. (2004). Biocontrol of Bacillus subtilis against infection of Arabidopsis roots by Pseudomonas syringae is facilitated by biofilm formation and surfactin production. Plant Physiol., 134, 307-319.
Bais, H. P., Weir, T. L., Perry, L. G., Gilroy, S., & Vivanco, J. M. (2006). The role of root exudates in rhizosphere interactions with plants and other organisms. Annu. Rev. Plant Biol., 57, 233-266.
Beris, D., Theologidis, I., Skandalis, N., & Vassilakos, N. (2018). Bacillus amyloliquefaciens strain MBI600 induces salicylic acid dependent resistance in tomato plants against Tomato spotted wilt virus and Potato virus Y. Scientific Reports, 8(1). https://doi.org/10.1038/s41598-018-28677-3
Bhattacharya, S., Das, A., Samadder, S., & Rajan, S. S. (2016). Biosynthesis and characterization of a thermostable, alkali-tolerant chitinase from Bacillus pumilus JUBCH08 displaying antagonism against phytopathogenic Fusarium oxysporum. 3 Biotech., 6(1). https://doi.org/10.1007/s13205-016-0406-x
Bibi, S., Weis, K., Kaur, A., Bhandari, R., Goss, E. M., Jones, J. B., & Potnis, N. A. (2023). Brief Evaluation of Copper Resistance Mobile Genetic Island in the Bacterial Leaf Spot Pathogen, Xanthomonas euvesicatoria pv. perforans. Phytopathology. https://doi.org/https://doi.org/10.1094/PHYTO-02-23-0077-SC
Bigeard, J., Colcombet, J., & Hirt, H. (2015). Signaling Mechanisms in Pattern-Triggered Immunity (PTI). Mol. Plant, 8, 521-539.
Borriss, R. (2020). Phytostimulation and Biocontrol by the Plant-Associated Bacillus amyloliquefaciens FZB42: An Update. In (pp. 1-20). Springer Singapore. https://doi.org/10.1007/978-981-15-2576-6_1
Cao, Y., Zhang, Z., Ling, N., Yuan, Y., Zheng, X., Shen, B., & Shen, Q. (2011). Bacillus subtilis SQR 9 can control Fusarium wilt in cucumber by colonizing plant roots. Biol. Fertil. Soils, 47, 495-506.
Chen, Y., Yan, F., Chai, Y., Liu, H., Kolter, R., Losick, R., & Guo, J.-H. (2013). Biocontrol of tomato wilt disease by Bacillus subtilis isolates from natural environments depends on conserved genes mediating biofilm formation. Environmental Microbiology, 15(3), 848-864. https://doi.org/10.1111/j.1462-2920.2012.02860.x
Cheng, T., Yao, X.-Z., Wu, C.-Y., Zhang, W., He, W., & Dai, C.-C. (2020). Endophytic Bacillus megaterium triggers salicylic acid-dependent resistance and improves the rhizosphere bacterial community to mitigate rice spikelet rot disease. Appl. Soil Ecol., 156, 103710. https://doi.org/https://doi.org/10.1016/j.apsoil.2020.103710
Chou, H.-P., Huang, Y.-C., Lin, Y.-H., & Deng, W.-L. (2022). Selection, Formulation, and Field Evaluation of Bacillus amyloliquefaciens PMB01 for Its Application to Manage Tomato Bacterial Wilt Disease. Agriculture, 12(10), 1714. https://doi.org/10.3390/agriculture12101714
Chuang, C.-Y., Lin, S.-T., Li, A.-T., Li, S.-H., Hsiao, C.-Y., & Lin, Y.-H. (2022). Bacillus amyloliquefaciens PMB05 Increases Resistance to Bacterial Wilt by Activating Mitogen-Activated Protein Kinase and Reactive Oxygen Species Pathway Crosstalk in Arabidopsis thaliana. Phytopathology, 112, 2495-2502.
Cooksey, D. A. (1994). Molecular mechanisms of copper resistance and accumulation in bacteria. FEMS Microbiol. Rev., 14, 381-386. https://doi.org/110.1111/j.1574-6976.1994.tb00112.x.
Cui, W., He, P., Munir, S., He, P., He, Y., Li, X., Yang, L., Wang, B., Wu, Y., & He, P. (2019). Biocontrol of Soft Rot of Chinese Cabbage Using an Endophytic Bacterial Strain. Front. Microbiol., 10, 1471. https://doi.org/doi: 10.3389/fmicb.2019.01471
da Silva Gündel, S., Dos Reis, T. R., Copetti, P. M., Favarin, F. R., Sagrillo, M. R., da Silva, A. S., Segat, J. C., Baretta, D., & Ourique, A. F. (2019). Evaluation of cytotoxicity, genotoxicity and ecotoxicity of nanoemulsions containing Mancozeb and Eugenol. Ecotoxicol. Environ. Saf., 169, 207-215. https://doi.org/doi:10.1016/j.ecoenv.2018.11.023
de Souza Espindola Santos, A., Parks, C. G., Senna, M. M., de Carvalho, L. V. B., & Meyer, A. (2021). Exposure to pesticides and oxidative stress in Brazilian agricultural communities. Biomarkers, 26, 539-547. https://doi.org/10.1080/1354750X.2021.1933593
de Weert, S., Vermeiren, H., Mulders, I. H., Kuiper, I., Hendrickx, N., Bloemberg, G. V., Vanderleyden, J., de Mot, R., & Lugtenberg, B. J. (2002). Flagella-Driven Chemotaxis Towards Exudate Components Is an Important Trait for Tomato Root Colonization by Pseudomonas fluorescens. . Mol. Plant-Microbe Interact., 15, 1173-1180.
Demoz, B. T., & Korsten, L. (2006). Bacillus subtilis attachment, colonization, and survival on avocado flowers and its mode of action on stem-end rot pathogens. Biological Control, 37(1), 68-74. https://doi.org/https://doi.org/10.1016/j.biocontrol.2005.11.010
Dong, W., Long, T., Ma, J., Wu, N., Mo, W., Zhou, Z., Jin, J., Zhou, H., & Ding, H. (2023). Effects of Bacillus velezensis GUAL210 control on edible rose black spot disease and soil fungal community structure. Front. Microbiol., 14, 1199024. https://doi.org/10.3389/fmicb.2023.1199024
Dorigan, A. F., Moreira, S. I., Ceresini, P. C., Pozza, E. A., Belan, L. L., da Silveira, P. R., & Alves, E. (2022). Higher fitness and competitive advantage of Pyricularia oryzae Triticum lineage resistant to QoI fungicides. Pest Manag. Sci., 78, . https://doi.org/10.1002/ps.7144.
Elshaghabee, F. M. F., Rokana, N., Gulhane, R. D., Sharma, C., & Panwar, H. (2017). Bacillus As Potential Probiotics: Status, Concerns, and Future Perspectives. Front. Microbiol. , 8, 1490. https://doi.org/10.3389/fmicb.2017.01490
Elsharkawy, M. M., Elsawy, M. M., & Ismail, I. A. (2022). Mechanism of resistance to Cucumber mosaic virus elicited by inoculation with Bacillus subtilis subsp. subtilis. Pest Manag. Sci., 78, 86-94. https://doi.org/https://doi.org/10.1002/ps.6610
Fira, D., Dimkić, I., Berić, T., Lozo, J., & Stanković, S. (2018). Biological control of plant pathogens by Bacillus species. J. Biotechnol., 285, 44-55. https://doi.org/https://doi.org/10.1016/j.jbiotec.2018.07.044
FRAC. FRAC Code List ©*2022: Fungal Control Agents Sorted by Cross Resistance Pattern and Mode of Action (Including Coding for FRAC Groups on Product Labels). Retrieved 30, August from https://www.frac.info/docs/default-source/publications/frac-code-list/frac-code-list-2022--final.pdf?sfvrsn=b6024e9a_2
Gallegos-Monterrosa, R., Mhatre, E., & Kovács, Á. T. (2016). Specific Bacillus subtilis 168 variants form biofilms on nutrient-rich medium. Microbiology, 162(11), 1922-1932.
Gao, T., Wang, X., Qin, Y., Ren, Z., & Zhao, X. (2023). Watermelon Root Exudates Enhance Root Colonization of Bacillus amyloliquefaciens TR2. Curr. Microbiol., 80, 110.
Gomaa, E. Z. (2012). Chitinase production by Bacillus thuringiensis and Bacillus licheniformis: Their potential in antifungal biocontrol. J. Microbiol., 50(1), 103-111. https://doi.org/10.1007/s12275-012-1343-y
Hammerschmidt, R., Métraux, J.-P., & Van Loon, L. C. (2001). Inducing Resistance: A Summary of Papers Presented at the First International Symposium on Induced Resistance to Plant Diseases, Corfu, May 2000. Eur. J. Plant Pathol., 107, 1-6.
Han, L., Zhang, M., Du, L., Zhang, L., & Li, B. (2022). Effects of Bacillus amyloliquefaciens QST713 on Photosynthesis and Antioxidant Characteristics of Alfalfa (Medicago sativa L.) under Drought Stress. Agronomy, 12(9), 2177. https://doi.org/10.3390/agronomy12092177
Ho, T.-H., Chuang, C.-Y., Zheng, J.-L., Chen, H.-H., Liang, Y.-S., Huang, T.-P., & Lin, Y.-H. (2020). Bacillus amyloliquefaciens Strain PMB05 Intensifies Plant Immune Responses to Confer Resistance Against Bacterial Wilt of Tomato. Phytopathology, 110(12), 1877-1885. https://doi.org/10.1094/phyto-01-20-0026-r
Hsiao, C.-Y., Blanco, S. D., Peng, A.-L., Fu, J.-Y., Chen, B.-W., Luo, M.-C., Xie, X.-Y., & Lin, Y.-H. (2023). Seed Treatment with Calcium Carbonate Containing Bacillus amyloliquefaciens PMB05 Powder Is an Efficient Way to Control Black Rot Disease of Cabbage. Agriculture, 13(5), 926. https://doi.org/10.3390/agriculture13050926
Huang, C.-N., Lin, C.-P., Hsieh, F.-C., Lee, S.-K., Cheng, K.-C., & Liu, C.-T. (2016). Characterization and evaluation of Bacillus amyloliquefaciens strain WF02 regarding its biocontrol activities and genetic responses against bacterial wilt in two different resistant tomato cultivars. World J. Microbiol. Biotechnol., 32(11). https://doi.org/10.1007/s11274-016-2143-z
Huang, M., Bulut, A., Shrestha, B., Matera, C., Grundler, F. M. W., & Schleker, A. S. S. (2021). Bacillus firmus I-1582 promotes plant growth and impairs infection and development of the cyst nematode Heterodera schachtii over two generations. Sci. Rep., 11, 14114. https://doi.org/doi: 10.1038/s41598-021-93567-0
Jang, S., Choi, S. K., Zhang, H., Zhang, S., Ryu, C. M., & Kloepper, J. W. (2023). History of a model plant growth-promoting rhizobacterium, Bacillus velezensis GB03: from isolation to commercialization. Front Plant Sci, 14, 1279896. https://doi.org/10.3389/fpls.2023.1279896
Jiang, C., Fan, Z., Li, Z., Niu, D., Li, Y., Zheng, M., Wang, Q., Jin, H., & Guo, J. (2020). Bacillus cereus AR156 triggers induced systemic resistance against Pseudomonas syringae pv. tomato DC3000 by suppressing miR472 and activating CNLs‐mediated basal immunity in Arabidopsis. Mol. Plant Pathol., 21(6), 854-870. https://doi.org/10.1111/mpp.12935
Jin, Y., Zhu, H., Luo, S., Yang, W., Zhang, L., Li, S., Jin, Q., Cao, Q., Sun, S., & Xiao, M. (2019). Role of Maize Root Exudates in Promotion of Colonization of Bacillus velezensis Strain S3-1 in Rhizosphere Soil and Root Tissue. . Curr. Microbiol., 76, 855-862.
Jones, J. D. G., & Dangl, J. L. (2006). The plant immune system. Nature, 444, 323-329.
Kamle, M., Borah, R., Bora, H., Jaiswal, A. K., Singh, R. K., & Kumar, P. (2020). Systemic Acquired Resistance (SAR) and Induced Systemic Resistance (ISR): Role and Mechanism of Action Against Phytopathogens. In (pp. 457-470). Springer International Publishing. https://doi.org/10.1007/978-3-030-41870-0_20
Kasim, W. A., Gaafar, R. M., Abou-Ali, R. M., Omar, M. N., & Hewait, H. M. (2016). Effect of biofilm forming plant growth promoting rhizobacteria on salinity tolerance in barley. Ann. Agric. Sci., 61(2), 217-227.
Leroux, P., Fritz, R., Debieu, D., Albertini, C., Lanen, C., Bach, J., Gredt, M., & Chapeland, F. (2002). Mechanisms of resistance to fungicides in field strains of Botrytis cinerea. Pest Manag. Sci., 58, 876-888.
Liang, Y.-S., Fu, J.-Y., Chao, S.-H., Tzean, Y., Hsiao, C.-Y., Yang, Y.-Y., Chen, Y.-K., & Lin, Y.-H. (2022). Postharvest Application of Bacillus amyloliquefaciens PMB04 Fermentation Broth Reduces Anthracnose Occurrence in Mango Fruit. Agriculture, 12(10), 1646. https://doi.org/10.3390/agriculture12101646
Liao, J.-H., Chen, P.-Y., Yang, Y.-L., Kan, S.-C., Hsieh, F.-C., & Liu, Y.-C. (2016). Clarification of the Antagonistic Effect of the Lipopeptides Produced by Bacillus amyloliquefaciens BPD1 against Pyricularia oryzae via In Situ MALDI-TOF IMS Analysis. Molecules, 21(12), 1670. https://doi.org/10.3390/molecules21121670
Lin, C., Tsai, C.-H., Chen, P.-Y., Wu, C.-Y., Chang, Y.-L., Yang, Y.-L., & Chen, Y.-L. (2018). Biological control of potato common scab by Bacillus amyloliquefaciens Ba01. PLOS ONE, 13(4), e0196520. https://doi.org/10.1371/journal.pone.0196520
Lugtenberg, B., & Kamilova, F. (2009). Plant-growth-promoting rhizobacteria. Annu. Rev. Microbiol., 63, 541-556.
Mishra, S., & Arora, N. K. (2012). Evaluation of rhizospheric Pseudomonas and Bacillus as biocontrol tool for Xanthomonas campestris pv campestris. World J. Microbiol. Biotechnol., 28, 693-702. https://doi.org/doi: 10.1007/s11274-011-0865-5.
Mora, I., Cabrefiga, J., & Montesinos, E. (2011). Antimicrobial peptide genes in Bacillus strains from plant environments. Int. Microbiol., 14, 213-223. https://doi.org/doi: 10.2436/20.1501.01.151.
Muthamilarasan, M., & Prasad, M. (2013). Plant innate immunity: An updated insight into defense mechanism. J. Biosci., 38, 433-449.
Newman, M.-A., Sundelin, T., Nielsen, J. T., & Erbs, G. (2013). MAMP (microbe-associated molecular pattern) triggered immunity in plants. Front. Plant Sci., 4, 139. https://doi.org/doi: 10.3389/fpls.2013.00139
Ngou, B. P. M., Jones, J. D. G., & Ding, P. (2022). Plant immune networks. Trends Plant Sci., 27(3), 255-273. https://doi.org/10.1016/j.tplants.2021.08.012
Nicholson, W. L., Munakata, N., Horneck, G., Melosh, H. J., & Setlow, P. (2000). Resistance of Bacillus Endospores to Extreme Terrestrial and Extraterrestrial Environments. Microbiol. Mol. Biol. Rev., 64, 548–572.
Olishevska, S., Nickzad, A., & Déziel, E. (2019). Bacillus and Paenibacillus secreted polyketides and peptides involved in controlling human and plant pathogens. Appl. Microbiol. Biotechnol., 103(3), 1189-1215. https://doi.org/10.1007/s00253-018-9541-0
Ongena, M., & Jacques, P. (2008). Bacillus lipopeptides: versatile weapons for plant disease biocontrol. Trends in Microbiology, 16(3), 115-125. https://doi.org/https://doi.org/10.1016/j.tim.2007.12.009
Pang, L., Xia, B., Liu, X., Yi, Y., Jiang, L., Chan, C., Li, P., Zhang, M., Deng, X., & Wang, R. (2021). Improvement of antifungal activity of a culture filtrate of endophytic Bacillus amyloliquefaciens isolated from kiwifruit and its effect on postharvest quality of kiwifruit. J Food Biochem., 45, e13551. https://doi.org/ https://doi.org/10.1111/jfbc.13551
Peng, D., Luo, K., Jiang, H., Deng, Y., Bai, L., & Zhou, X. (2017). Combined use of Bacillus subtilis strain B-001 and bactericide for the control of tomato bacterial wilt. Pest Manag. Sci., 73, 1253-1257. https://doi.org/doi: 10.1002/ps.4453.
Pieterse, C. M. J., Leon-Reyes, A., Van der Ent, S., & Van Wees, S. C. M. (2009). Networking by small-molecule hormones in plant immunity. Nature Chem. Biol., 5, 308-316.
Rabbee, M., Ali, M., Choi, J., Hwang, B., Jeong, S., & Baek, K.-H. (2019). Bacillus velezensis: A Valuable Member of Bioactive Molecules within Plant Microbiomes. Molecules, 24(6), 1046. https://doi.org/10.3390/molecules24061046
Radhakrishnan, R., Hashem, A., & Abd Allah, E. F. (2017). Bacillus: A Biological Tool for Crop Improvement through Bio-Molecular Changes in Adverse Environments. Front. Physiol., 8, 667. https://doi.org/doi: 10.3389/fphys.2017.00667
Rendina, N., Nuzzaci, M., Sofo, A., Campiglia, P., Scopa, A., Sommella, E., Pepe, G., De Nisco, M., Basilicata, M. G., & Manfra, M. (2019). Yield parameters and antioxidant compounds of tomato fruit: the role of plant defence inducers with or without Cucumber mosaic virus infection. J. Sci. Food Agri., 99(12), 5541-5549. https://doi.org/10.1002/jsfa.9818
Ribas E. Ribas, A. D., Spolti, P., Del Ponte, E. M., Donato, K. Z., Schrekker, H., & Fuentefria, A. M. (2016). Is the emergence of fungal resistance to medical triazoles related to their use in the agroecosystems? A mini review. Braz. J. Microbiol., 47, 793-799. https://doi.org/10.1016/j.bjm.2016.06.006
Rivelli Zea, S. M., & Toyotome, T. (2022). Azole-resistant Aspergillus fumigatus as an emerging worldwide pathogen. Microbiol. Immunol., 66, 135-144. https://doi.org/10.1111/1348-0421.12957.
Samaras, A., Nikolaidis, M., Antequera-Gómez, M. L., Cámara-Almirón, J., Romero, D., Moschakis, T., Amoutzias, G. D., & Karaoglanidis, G. S. (2021). Whole genome sequencing and root colonization studies reveal novel insights in the biocontrol potential and growth promotion by Bacillus subtilis MBI 600 on cucumber. Frontiers in Microbiology, 11, 600393.
Sharma, M., Saleh, D., Charron, J. B., & Jabaji, S. (2020). A crosstalk between Brachypodium root exudates, organic acids, and Bacillus velezensis B26, a growth promoting bacterium. Front. Microbiol., 11, 575578.
Sui, X., Han, X., Cao, J., Li, Y., Yuan, Y., Gou, J., Zheng, Y., Meng, C., & Zhang, C. (2022). Biocontrol potential of Bacillus velezensis EM-1 associated with suppressive rhizosphere soil microbes against tobacco bacterial wilt. Front. Microbiol., 13, 940156. https://doi.org/doi: 10.3389/fmicb.2022.940156
Tan, S., Yang, C., Mei, X., Shen, S., Raza, W., Shen, Q., & Xu, Y. (2013). The effect of organic acids from tomato root exudates on rhizosphere colonization of Bacillus amyloliquefaciens T-5. Appl. Soil Ecol., 64, 15-22.
Tian, X.-l., Zhao, X.-M., Zhao, S.-Y., Zhao, J.-l., & Mao, Z.-C. (2022). The Biocontrol Functions of Bacillus velezensis Strain Bv-25 Against Meloidogyne incognita. Front. Microbiol., 13, 843041. https://doi.org/ doi: 10.3389/fmicb.2022.843041
Wang, D.-C., Jiang, C.-H., Zhang, L.-N., Chen, L., Zhang, X.-Y., & Guo, J.-H. (2019). Biofilms Positively Contribute to Bacillus amyloliquefaciens 54-induced Drought Tolerance in Tomato Plants. Intl. J. Mol. Sci., 20(24), 6271. https://www.mdpi.com/1422-0067/20/24/6271
Wang, F., Chao, S.-H., Tsai, C.-H., Blanco, S. D., Yang, Y.-Y., & Lin, Y.-H. (2023). Developing Fermentation Liquid of Bacillus amyloliquefaciens PMB04 to Control Bacterial Leaf Spot of Sweet Pepper. Agriculture, 13(7), 1456. https://doi.org/10.3390/agriculture13071456
Wang, S., Zheng, Y., Gu, C., He, C., Yang, M., Zhang, X., Guo, J., Zhao, H., & Niu, D. (2018). Bacillus cereus AR156 Activates Defense Responses to Pseudomonas syringae pv. tomato in Arabidopsis thaliana Similarly to flg22. Mol. Plant-Microbe Interact., 31(3), 311-322. https://doi.org/10.1094/mpmi-10-17-0240-r
Weng, J., Wang, Y., Li, J., Shen, Q., & Zhang, R. (2013). Enhanced root colonization and biocontrol activity of Bacillus amyloliquefaciens SQR9 by abrB gene disruption. Appl. Microbiol. Biotechnol., 97, 8823-8830.
Wu, J.-J., Huang, J.-W., & Deng, W.-L. (2020). Phenylacetic Acid and Methylphenyl Acetate From the Biocontrol Bacterium Bacillus mycoides BM02 Suppress Spore Germination in Fusarium oxysporum f. sp. lycopersici. Front. Microbiol., 11, 569263. https://doi.org/10.3389/fmicb.2020.569263
Wu, L., Huang, Z., Li, X., Ma, L., Gu, Q., Wu, H., Liu, J., Borriss, R., Wu, Z., & Gao, X. (2018). Stomatal Closure and SA-, JA/ET-Signaling Pathways Are Essential for Bacillus amyloliquefaciens FZB42 to Restrict Leaf Disease Caused by Phytophthora nicotianae in Nicotiana benthamiana. Front. Microbiol., 9, 847. https://doi.org/doi: 10.3389/fmicb.2018.00847
Xiao, J., Guo, X., Qiao, X., Zhang, X., Chen, X., & Zhang, D. (2021). Activity of Fengycin and Iturin A Isolated From Bacillus subtilis Z-14 on Gaeumannomyces graminis Var. tritici and Soil Microbial Diversity. Front. Microbiol., 12, 682437. https://doi.org/doi: 10.3389/fmicb.2021.682437
Yasmeen, T., Ahmad, A., Arif, M. S., Mubin, M., Rehman, K., Shahzad, S. M., Iqbal, S., Rizwan, M., Ali, S., Alyemeni, M. N., & Wijaya, L. (2020). Biofilm forming rhizobacteria enhance growth and salt tolerance in sunflower plants by stimulating antioxidant enzymes activity. Plant Physiol. Biochem., 156, 242-256. https://doi.org/https://doi.org/10.1016/j.plaphy.2020.09.016
Yi, H.-S., Yang, J.-W., & Ryu, C.-M. (2013). ISR meets SAR outside: additive action of the endophyte Bacillus pumilus INR7 and the chemical inducer, benzothiadiazole, on induced resistance against bacterial spot in field-grown pepper. Frontiers in Plant Science, 4.
Yu, Y.-Y., Dou, G.-X., Sun, X.-X., Chen, L., Zheng, Y., Xiao, H.-M., Wang, Y.-P., Li, H.-Y., Guo, J.-H., & Jiang, C.-H. (2021). Transcriptome and Biochemical Analysis Jointly Reveal the Effects of Bacillus cereus AR156 on Postharvest Strawberry Gray Mold and Fruit Quality. Front. Plant Sci., 12, 700446. https://doi.org/doi: 10.3389/fpls.2021.700446
Yuan, J., Zhang, N., Huang, Q., Raza, W., Li, R., Vivanco, J. M., & Shen, Q. (2015). Organic acids from root exudates of banana help root colonization of PGPR strain Bacillus amyloliquefaciens NJN-6. Sci. Rep., 5, 13438.
Yuan, M., Ngou, B.-P.-M., Ding, P., & Xin, F.-X. (2021). PTI-ETI crosstalk: an integrative view of plant immunity. Curr. Opin. Plant Biol., 62.
Zhang, N., Wu, K., He, X., Li, S. Q., Zhang, Z. H., Shen, B., Yang, X. M., Zhang, R. F., Huang, Q. W., & Shen, Q. R. (2011). A new bioorganic fertilizer can effectively control banana wilt by strong colonization with Bacillus subtilis N11. Plant Soil, 344, 87-97.
Zhang, S., Zheng, Q., Xu, B., & Liu, J. (2019). Identification of the Fungal Pathogens of Postharvest Disease on Peach Fruits and the Control Mechanisms of Bacillus subtilis JK-14. Toxins, 11(6), 322. https://doi.org/10.3390/toxins11060322
Zhang, W., Seminara, A., Suaris, M., Brenner, M. P., Weitz, D. A., & Angelini, T. E. (2014). Nutrient depletion in Bacillus subtilis biofilms triggers matrix production. New J. Phys., 16(1), 015028.
Zhou, Q., Fu, M., Xu, M., Chen, X., Qiu, J., Wang, F., Yan, R., Wang, J., Zhao, S., Xin, X., & Chen, L. (2020). Application of antagonist Bacillus amyloliquefaciens NCPSJ7 against Botrytis cinerea in postharvest Red Globe grapes. Food Sci. Nutr., 8(3), 1499-1508. https://doi.org/10.1002/fsn3.1434