DOI: https://doi.org/10.56669/VNFT9299
ABSTRACT
Biological pesticides, derived from biocontrol agents, manage agricultural pests and diseases, and boost crop production. These pesticides come from microorganisms (metabolites), plants (exudates, essential oils, and extracts from bark, root, and leaves), and biologically derived nanoparticles (like copper and zinc-based nanoparticles). In India, key microbial formulations include Bacillus thuringiensis, Trichoderma, NPV, and neem-based insecticides. These microbes release specific metabolites that protect plants from pests and diseases, making them highly effective. Unlike synthetic pesticides, microbial formulations are specific in action, cost-effective, and environmentally friendly, leaving no harmful residues. The use of biopesticides in India has increased, with consumption rising to 8,647 metric tons in 2020-21 and 8,898 metric tons in 2021-22. Despite their benefits, field-level adoption remains limited. Addressing challenges in delivery and consistent performance is crucial for broader adoption.
Keywords: Microbial formulation, Biopesticides, Integrated Pest Management, Chemical pesticides
INTRODUCTION
In modern agriculture, pesticides have been crucial for boosting crop yields, regulating plant growth, and controlling pests and diseases. However, the global economy and heightened concerns over chemical pesticide residues have led to stricter trade standards, especially in Europe. Ideally, pesticides should target pests effectively without harming non-target species, including humans. Biopesticides present a promising alternative, effective in pest management and promoting sustainable agriculture (Prabha et al., 2016). Despite their potential, technical challenges have hindered their widespread adoption (Nielsen et al., 2008). In the European Union, Integrated Pest Management (IPM) policies emphasize innovative pest control methods, particularly biological pesticides derived from living microorganisms or natural products (Chandler et al., 2011). These biopesticides, sourced from naturally occurring bacteria, fungi, protozoa, yeasts, and viruses, are recognized for their target specificity, environmental safety, efficacy, biodegradability, and suitability in integrated disease management programs. They include various types, such as microbial formulations, plant-incorporated protectants, and bio-chemicals, playing a crucial role in IPM strategies that prioritize eco-friendly and safer alternatives to chemical pesticides. Since their introduction, many biopesticide products have been developed and some have gained market prominence.
This paper discusses the current status, potential to replace chemical pesticides, future prospects, and the challenges associated with biopesticides in plant pest and disease management.
Can microbe based pesticides be the option of chemical pesticides?
Several factors indicate that microbe based pesticides are excellent alternatives to synthetic pesticides. Specifically, they are highly effective, target-specific and have fewer environmental risks. Microbial formulations can come from a wide diversity of organisms and many products have been released and registered in the agromarket. Microbial formulations allow for a sustainable approach for improved crop production, which should increase their use and popularity in the coming years (Mishra et al., 2015). Moreover, opinions about microbial formulation use have begun to change because of the recent recognition of the environmental consequences of chemical pesticides. Other factors contributing to their increased use are advances in activity spectra spots and improved options for delivery and application (Glare et al., 2012). Current research into microbial formulations focuses on the improvement of their mode of action, including mechanisms to replace the use of chemical pesticides in integrated disease management programe (Nawaz et al., 2016). IPM is a method to incorporate chemical, biological and physical methods for pest and disease control (Ghewande and Nandagopal, 1997). The main challenges of new biological pesticides in the growth and utilization are how to market or promote it (Tripathi et al., 2020) and how to enhance the stability and residual action of microbial formulation (Damalas and Koutroubas, 2018). Previously, there were several methods applied for handling pests, such as traditional methods, chemical methods and biological methods (Lindsey et al., 2020).
Microbial formulations
Microbial formulations are compounds used to control plant pests and diseases that are derived from beneficial microorganisms or the metabolic products they produce. The microbiome or microorganisms as a biological pesticide is another option that has been shown to be effective against insect pests and diseases. Therefore, switching to biopesticides is absolutely necessary to replace these chemical pesticides, as they offer a more sustainable and environmentally friendly alternative, reducing the harmful effects on ecosystems, human health, and beneficial organisms while addressing the growing issue of pesticide resistance (Kumari et al., 2014). Microbial formulations and biopesticides (PGPR, bio stimulants) based on living microbes and their bioactive components have been intensively studied, published, and promoted in order to efficiently manage plant pests and diseases and stimulate plant growth. These are done by applying large varieties of microorganisms including fungi, bacteria viruses, protozoans, and nematodes as microbial formulations (Singh, 2022). Pseudomonas fluorescens, Bacillus subtilis, Beauvaria bassiana, Gliocladium spp., Trichoderma, and Metarrhizium anisopliae are among the nine microbes listed in a schedule published in the Indian Gazette on March 26, 1999, as an amendment to the Insecticides Act, 1968, for the commercial production of biopesticides. The Insecticide Act of 1968 included 26 additional microorganisms on its list of targets for the development of microbial biopesticides (Singh, 2022).
Why microbial formulation?
Microbial formulations are species-specific and do not harm other beneficial organisms (Kumari et al., 2014). They may occur naturally in the environment or be genetically modified for the production of toxicants, which exhibits species-specific reactions. Today's commonly used microbial formulations are microorganisms that have a harmful effect on pest and pathogen populations. These biopesticides are also effective in small amounts and dissolve quickly, resulting in a poorer revelation and more environmentally friendly technique (Thakur et al., 2020). These biopesticides break down quickly, resulting in reduced residual effects and a more environmentally friendly approach. Although traditional agrochemical pesticides have been effective in controlling pests and diseases for many years, their long-term use has led to diminishing efficacy. This is due to the rise of pesticide-resistant pests and diseases and the growing concern over environmental and human health risks, leading to the suspension or deregulation of many chemical pesticides. Consequently, there is an urgent need for eco-friendly alternatives. Higher quality and increased quantity of agricultural products are examples of pest management strategy advancements. As a result, there is a critical need for the development of beneficial, sustainable, and non-toxic biopesticides. To avoid the negative effects of chemical pesticides, priority should be given to the use of biopesticides against pests and diseases (Mazid et al., 2011 and Pathak et al., 2017).
Types and function of microbial formulations
Bacteria
For decades, bacteria have been introduced into soil, seeds, roots, and other plant structures to enhance plant growth and development. These bacteria promote beneficial relationships such as nitrogen fixation, hazardous chemical degradation, plant growth promotion, and biological control of pathogenic microorganisms. Numerous bacterial genera, including Acinetobacter, Agrobacterium, Alcaligenes, Arthrobacter, Azospirillum, Azotobacter, Bacillus, Bradyrhizobium, Frankia, Pantoea, Pseudomonas, Rhizobium, Serratia, Stenotrophomonas, Streptomyces, Enterobacter, Erwinia, Thiobacillus, and Xanthomonas, have been reported to protect plants against fungal and bacterial pathogens (Whipps 2001, Montesinos and Bonaterra, 2009). Among these, Pseudomonas spp., Bacillus spp., and Streptomyces spp. have been intensively studied and registered as commercial products.
Research has shown that these bacteria can prevent various plant diseases, including nematode infestations, fungal infections, and bacterial diseases (Tariq et al., 2020). Bacterial biocontrol agents employ a wide range of strategies to protect plants against pathogens. They can compete for resources and space, interfere with pathogen pathogenicity, and secrete antimicrobial compounds. Many bacterial biocontrol agents release antimicrobial metabolites such as lipopeptides, bacteriocins, antibiotics, biosurfactants, cell-wall disintegrating enzymes, or microbial volatile compounds. They can also disrupt the quorum sensing (QS) systems of pathogens, interfering with signal molecules used to initiate infections (Kalia et al., 2019; Bonaterra et al., 2022). Table 1 shows a list of commercially available bacterial bioformulations for pest management of food crops worldwide.
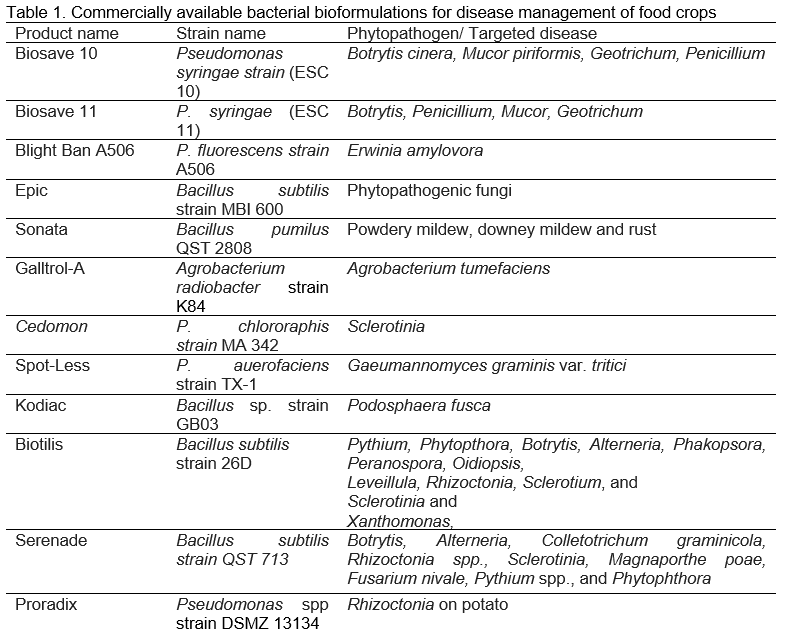
Fungi
Fungi have evolved mechanisms to inhibit the growth of pathogenic fungi detrimental to plant development. The focus on developing fungal strains as biocontrol agents for plant diseases has been significant, with Trichoderma spp. being extensively studied. These fungi, particularly Trichoderma viride and Trichoderma harzianum, are used in various products due to their ability to reduce abiotic and biotic stress on host plants by suppressing a range of plant diseases and nematodes. T. viride is effective against soil-borne pathogens such as Fusarium, Sclerotium, Rhizoctonia, and Pythium, while T. harzianum also targets Botrytis, Gaeumannomyces, Sclerotinia, Verticillium, and wood-rot fungi (Vosátka et al., 2012).
Other fungal species, including Gliocladium and Aspergillus, exhibit antagonistic activities against pathogens like Alternaria, Pythium, Aspergillus, Fusarium, Rhizoctonia, Phytophthora, Botrytis, Pyricularia, and Gaeumannomyces (Pal and Gardener, 2006). Nematophagous fungi have shown promise as biological agents for nematode management, with key genera including Arthrobotrys, Duddingtonia flagrans, Monacrosporium, and Dactylaria (Senthilkumar et al., 2020). Paecilomyces lilacinus, a soil-borne fungus prevalent in tropical and subtropical regions, has significant potential as a biocontrol agent against plant-parasitic nematodes.
Entomopathogenic fungi, which infect and kill insects by penetrating their cuticle, are divided into several groups: Ascomycota, Zygomycota, Deuteromycota, Oomycota, and Chytridiomycota (Esparza-Mora et al., 2017). Most commercial products are based on three entomopathogenic fungal genera: Beauveria bassiana, Metarhizium, and Paecilomyces lilacinus. These fungi produce conidia that adhere to the insect cuticle and release enzymes, such as fungal protease, that break down the cuticle and allow the fungus to penetrate and kill the insect by consuming its tissues (Brunner-Mendoza et al., 2019; Dara, 2017). Table 2 shows a list of commercially available fungal formulations for targeted pathogens.
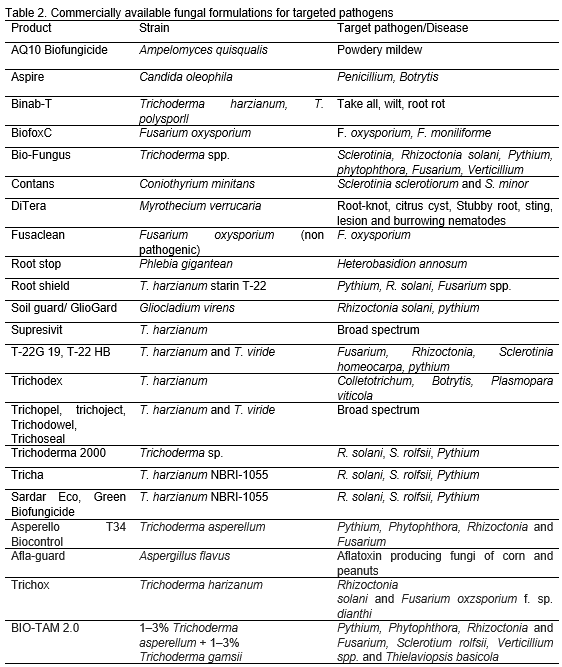
Actinomycetes
Actinomycetes can be used as a biocontrol agent on phytopathogenic fungus as an alternative to applying synthetic fungicides in recent years (Boukaew et al., 2022). Actinomycetes are Gram-positive bacteria that can live in a range of environments with varying humidity, pH levels, and temperatures. Actinomycetes have been isolated from a variety of habitats, including wetlands, terrestrial, marine, and hypersaline conditions (Jagannathan et al. 2021).
Competence for space and nutrients, production of antibiotics, siderophores, lytic enzymes, volatile organic compounds (VOCs), and host resistance induction are the principal antagonistic methods used by Actinomycetes to suppress phytopathogenic fungi. Actinomycetes also aid in the production of phytohormones, atmospheric nitrogen fixation, mineral solubilization, and other processes that aid in the growth and development of plants. Actinomycetes have been the subject of several research, however due to their utility as biocontrol agents in agriculture and their antagonistic processes to phytopathogenic fungi, few of these studies have concentrated on Actinomycetes isolated from different environments.
Actinomycetes as biocontrol agents
The significance of using Actinomycetes as biocontrol agents is explained by their inherent beneficial traits, which include the following: (1) they don't affect human or animal health; (2) they're not poisonous to plants; (3) they increase plant yield; and (4) they reduce the need for synthetic fungicides. Streptomyces has received the most research attention among the several genera since it is simple to isolate. Bacteria grow faster than Actinomycetes. To acquire desired Actinomycetes in culture media, growth enhancement approaches should be used. These methods are based on the pretreatment of samples using wet, chemical, and other pretreatment methods as well as selected isolation media and soil with calcium carbonate. By incorporating biostimulants and organic fertilisers like compost and vermicompost, one can encourage the populations of Actinomycetes in the soil. The maximum antagonistic activity was demonstrated by S. sampsonii and S. flavovariabilis isolates from soil enriched with vermicompost towards Rhizoctonia solani, Alternaria tenuissima, Aspergillus niger, and Penicillium expansum. Actinomycetes populations in the soil were also increased by adding Brassica napus and Brassica rapa leaf residue to the soil. The decline in the R. solani wilt disease was strongly correlated with the rise in the Actinomycetes population (Ascencion et al., 2015). Table 3 shows a list of commercially available Actinomycetes based formulations for targeted diseases.
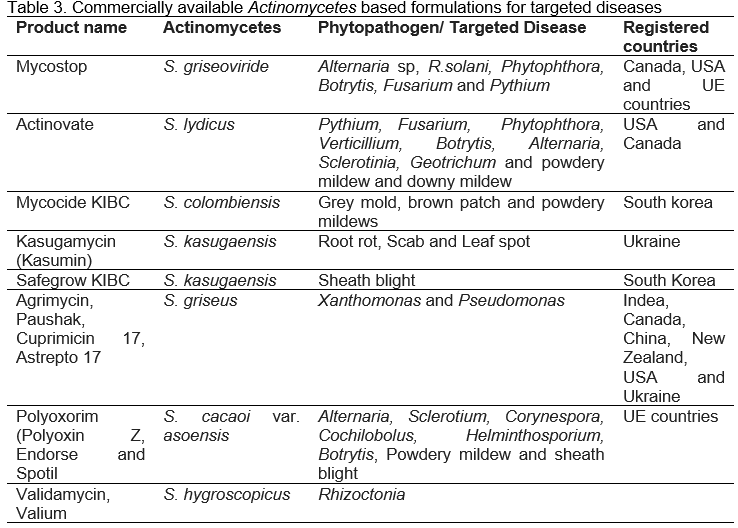
Mycovirus
Fungal viruses, also known as mycoviruses, are not known to kill or infect their hosts, but they are known to alter gene expression, morphology, growth, sporulation, pathogenicity, and interactions with other species. The possibility that mycoviruses could be a useful tool for biocontrol of fungi has sparked research into mycoviruses. Mycoviruses are known to slow down the development rate and/or virulence of their hosts.
Mycoviruses as biocontrol agents
The majority of plant diseases are brought on by fungi. Fungicide application is a typical technique for managing fungal phytopathogenic diseases. Continuous use of fungicides results in the development of fungicide-resistant strains, which has potential negative impacts on both humans and the environment. The use of biocontrol agents as an alternative to chemical control has grown significantly in relevance in recent years. Mycoviruses could also be employed as biocontrol agents. Nuss (1992) was the first to demonstrate the use of a dsRNA virus as a biocontrol agent against chestnut blight. The production of a cDNA copy of the viral RNA associated with the hypovirus and its transformation into a virulent fungal strain turned the compatible, virulent strain into a hypo virulent strain. Chestnut fungus was successfully managed in Europe with the use of CHV-1 cDNA, but in North America, the fungus had a wider genetic range and was more difficult to manage (Nuss, 1992). There are barriers to the viruses' successful transmission when they are transmitted by hyphal contact. The beneficial infection of viruses that decreases the pathogenicity of plant pathogenic fungi is known as hypovirulence. The most prevalent mycovirus trait, hypo virulence, is utilized to biologically control a number of plant diseases, including Cryphonecteria parasitica (chestnut blight), woody plant white root rot, rice blast, and a variety of soil-borne infections (Zhang and Nuss, 2016).
Mechanism
The exact mechanism underlying hypo pathogenicity is still unknown, although numerous theories have been offered by various researchers, including signal transduction pathways, RNA silencing of the fungus, and counter-silencing processes exploited by the hypovirus (Nuss, 2011). There are also other documented pathways for low virulence, including nuclear alterations, plasmids, and mutations of the mitochondria. The expansion of the host range of mycoviruses without the challenges of vegetative incompatibility is made possible by the production of cDNA infectious clones and other transformation techniques (Chen et al., 1994; Abid et al., 2018).
Bacteriophages
Viruses that infect and multiply in bacteria are known as phages. Temperate and lytic pathways are used in phage replication cycles, with the lytic pathway being the easier and more essential pathway for use in phage biocontrol. The challenges with antibiotic resistance, ineffective cultural practices, copper-based pesticides, and environmental chemical contamination have sparked global interest in Xanthomonas phage research and the use of biocontrol in agriculture (Nakayinga et al., 2021).
Bacteriophage-based biocontrol for the phytopathogen Xanthomonas has a long history, going all the way back to the early nineteenth century, when a filtrate of decaying cabbage prevented the spread of a disease known as Xanthomonas campestris pv. campestris. A few decades later, similar biocontrol efficacy was noted with phage-containing lysates that prevented Xanthomonas campestris pv. pruni-caused bacterial spot disease in peaches. Phage applications have advanced from in-vitro studies to field testing in a number of instances. Against bacterial leaf blight of rice (X. oryzae pv. oryzae), leaf blight of onion (X. axonopodis pv. allii), bacterial spot of tomato (X. campestris pv. vesicatoria), citrus canker (X. axonopodis pv. citri) and citrus bacterial spot (X. axonopodis pv. citrumelo), number of bacteriophages were isolated and evaluated. It has been demonstrated that two Xanthomonas phage products made by AgriPhage are effective in managing the pathogens responsible for citrus canker and tomato & pepper spot disease (Nakayinga et al., 2021).
Application of microbial formulations in plant disease management
The production of microbial formulations, which are used to manage a variety of diseases in agricultural crops all over the world, involves the use of fungi, bacteria, actinomycetes, bacteriophages, mycoviruses, and nematodes (Yadav and Devi, 2017). They are applied by spraying, soaking, or directly applying them to the roots in the form of a tablet, coating the seeds or treating the roots before planting, as well as by various insects (Montesinos and Bonaterra, 2009).
A variety of microbial formulations are available, including wettable powders, suspensions, oil suspensions, water-dispersible granules, and suspension seed coatings (Table 4). However, microbial formulation manufacturing is more difficult than chemical pesticide production (Lin et al., 2023). The microorganisms in pesticides are more susceptible to outside environmental conditions during preparation, such as UV radiation, temperature, and humidity.
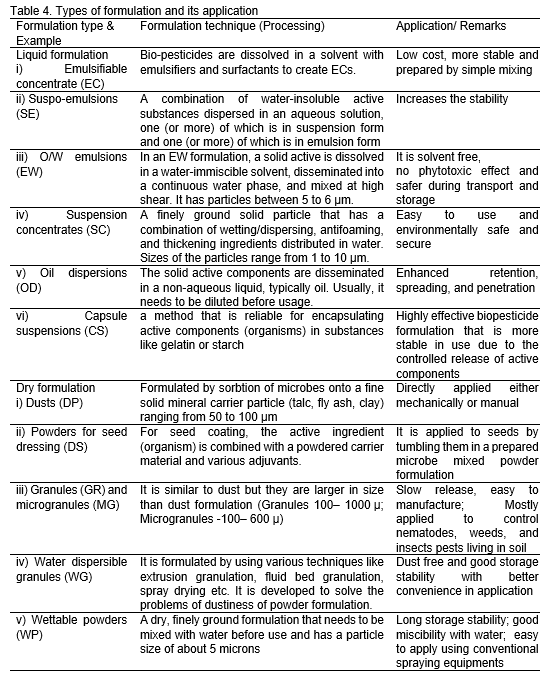
Formulation
The term "formulation" describes the process of creating a product from an active ingredient by adding specific active (functional) and inert (inactive) substances.
Principles of formulation
In order to maximize lifespan in storage, optimize application to the target, and protect the organisms after application, prepared organisms are suspended in a suitable carrier that is supplemented by additives. They differ from chemical active components in that they are particulate, living, or proteinous in nature, making them more susceptible to environmental factors and storage conditions (Bergis and Jones, 1998).
There are two types of biopesticide formulations based on their physical states:
• Liquid formulations; and
• Dry formulations.
Liquid formulations may be water, oil or polymer based or the combination of all. Water based formulations like suspension concentrate (SC), capsule suspension (CS) and suspo-emulsions (SE) requires the addition of inert materials viz., surfactants, stickers, stabilizers, antifreezing agents, coloring agents and nutritive materials.
Dry formulations like dusts (DP), granules (G), micro granules (MG), wettable powders (WP), and water dispersible granules (WG) are prepared by adding wetting agents, binding agents, dispersants etc., using a technologies like spray drying, air drying or freeze drying (Tadros, 2005; Brar,2006; Knowles, 2008).
Classifications and functions of adjuvants used for microbial formulations
The four basic categories of adjuvants employed in the production of microbial formulations are surfactants, transporters, protective agents, nutritional adjuvants etc.
Carriers
The basic function of a carrier is to serve as a tiny container or diluent for the active components of microbial formulations. High concentration powders, wettable powders, or granules can be produced using carriers with strong adsorption capabilities, such as diatomite, attapulgite, silica, and bentonite. To create low-concentration powders, diluents and fillers such as talc, pyrophyllite, sepiolite, and clay materials with low or moderate adsorption capabilities are typically utilised. Biodegradable and environmentally safe materials such as chitosan, carbon nanotube nanocomposite, biodiesel, and plant based substances like bagasse, corn bagasse, chaff powder, tobacco powder, and walnut shell powder are used as carriers. Though plant-based carriers have the property of absorbing ultraviolet rays (UV), their use in commercial production is very rare.
Surfactants
Surfactants are crucial in preserving the physical stability of biopesticides over the long term and enhancing their biological capabilities. Generally, microbial formulations comprise one or more surfactants. This surfactant helps to increase cost-effectiveness, save energy and raw materials, and increase processing efficiency.
Protective Agents
UV protection agents
UV ray absorbers and anti-oxidative UV protection agents are the two categories of UV protection agents utilized in the manufacturing of microbial formulations. Milk, optical brighteners, lignosulfonates, and locust toxin are examples of UV ray absorbers that may absorb the UV portion of sunlight and fluorescent light sources without transforming themselves. Rubus oil, hemp seed oil, kojic acid, hydroxykynurenic acid, flavonoids, and lecithin are anti-oxidative UV protection agents which have strong antioxidative effect (Lin etal, 2023).
Nutritional adjuvants
Microorganisms require water, inorganic salts, carbon sources, nitrogen sources, and growth nutrients in order to function normally throughout the growth phase. Nutritional adjuvants can give nutrition to the microorganisms that make up microbial formulations so they can reproduce more easily, grow and proliferate more quickly in the field.
Challenges and opportunities for commercialization of microbial formulation
Synthetic chemical pesticides were formerly the mainstay of crop protection, but their use is now declining due to new rules and restrictions, as well as the evolution of pest and pathogen resistance. As a result, a new pest and disease management strategy is required. A microbial formulation is an ideal solution for replacing synthetic chemical pesticides based on natural or living microorganisms. Microbial formulations have acquired importance as an essential part of IPM due to their economic feasibility and sustainability in comparison to chemical synthetic pesticides (Birch 2011). Microbial formulations are able to overcome the limitations of conventional pesticides as part of IPM programs since they are host-specific, biodegradable, and less toxic to beneficial microbes (Matyjaszczyk, 2015).
The effectiveness of microbial formulations in combating plant diseases depends on the microbial agent (mechanism of action, conditioning, dose, methods of application), the host (cultivar type, physical characteristics), the targeted plant pathogens (sensitivity), and environmental factors (biotic and abiotic factors, chemical residues, nutrient availability, temperature, and moisture). The variable factors like location, time period, host plants and their interactions may alter the effectiveness of microbial agents. Lack of efficacy and varied field performance have frequently been documented. The effectiveness and consistency of biological control must therefore be compared to those of conventional chemical fungicide and bactericide treatments under sufficiently broad production settings in fields representing various environments and agricultural practices (Montesinos, 2003; Köhl et al., 2011; and Bonaterra, 2022).
The demand for safer plant pest and disease control products leads to a preference for microbial biopesticide formulations with effective antagonistic activity and good stability. Biopesticides offer environmentally benign alternatives to synthetic pesticides, but they encounter a number of challenges during their development, formulation, and use. Maximum attention is required to maintain the microbial population and efficacy throughout the entire use of biopesticides because they typically contain living organisms. The commercialization of biopesticides could benefit greatly from research into their manufacture and formulation. As their application methods advance and less expensive inert components are acknowledged for various formulations, it looks that biopesticides will be used more widely in the future.
The introduction of new adjuvants demonstrated a notable increase in microbial activity and suggested a new avenue of research. The stability of the product, its longer shelf life, and the performance of the microorganisms in the field may all be improved by choosing the right formulation. In the future, formulation products should have a better balance between production cost and efficiency, as is the case with biopesticides, which provide a more balanced plant protection product application (El-Sayed, 2005; Rao et al., 2007; Glare et al., 2012; Khater, 2012). The formulation type could evolve from dusts to granules, from suspension concentrates and wettable powders to water-dispersible granules, and from products based on a single bacterium to formulations based on microbial consortiums.
CONCLUSION
The fact that components of microbial formulations are widely regarded as safe and are target-specific means that they generally have no negative effects on the environment and human beings. Compared to chemical pesticides, the use of them produces fewer greenhouse gas emissions. Moreover, a wide range of organisms can be used to develop microbial biopesticides, to tackle the issue of resistance and guarantee sustainability. It may be difficult for producers, marketers, and end users to manage their storage and transportation because different microorganisms employed as biopesticides may require varied storage conditions. Therefore, more study is required to ensure that microbial formulations have a sustainable and extended shelf life.
REFERENCES
Ascencion L.C., Liang W.J. and Yen T.B. (2015) Control of Rhizoctonia solani damping-off disease after soil amendment with dry tissues of Brassica results from increase in Actinomycetes population. Biol. Control 82: 21–30.
Birch A.N.E. (2011) How agro-ecological research helps to address food security issues under new IPM and pesticide reduction policies for global crop production systems. J. Exp. Bot., 62: 3251–3261.
Bonaterra A., Badosa E., Daranas N., Francés J., Roselló G. and Montesinos E. (2022) Bacteria as Biological Control Agents of Plant Diseases. Microorganisms 10: 1759. https://doi.org/10.3390/ microorganisms10091759
Boukaew S., Yossan S., Cheirsilp B. and Prasertsan P. (2022) Impact of environmental factors on Streptomyces spp. metabolites against Botrytis cinerea. J. Basic Microbiol., 62, 611–622.
Brar S.K., Tyagi V.R.D. and Valero JR (2006) Recent advances in downstream processes and formulations of Bacillus thuringiensis based biopesticide. Process Biochem., 41: 323–342.
Brunner-Mendoza C., Reyes-Montes M.R., Moonjely S., Bidochka M.J. and Toriello C. (2019) A review on the genus Metarhizium as an entomopathogenic microbial biocontrol agent with emphasis on its use and utility in Mexico. Biocontrol. Sci. Tech. 29: 83102.
Chandler D., Bailey A.S., Tatchell G.M., Davidson G. and Greaves J. et al. (2011) The development, regulation and use of biopesticides for integrated pest management. Philosophical Trans. Royal Society B: Biol. Sci., 366: 1987-1998. DOI: 10.1098/rstb.2010.0390
Chen B., Choi G.H. and Nuss D.L. (1994) Attenuation of fungal virulence by synthetic infectious hypovirus transcripts. Science, 264(5166): 1762-1764.
Damalas C.A. and Koutroubas S.D. (2018) Current status and recent developments in biopesticide use. Agriculture, 8: 1-6.
Dara S.K. (2017) Entomopathogenic microorganisms: modes of action and role in intregrated pest management. UC ANR e-Journal of Entomology and Biologicals, 20 May 2017. https://ucanr.edu/blogs/blogcore/postdetail.cfm?postnum 5 24119.
Dasgupta M.K. and Khan M.K. (2015) Nematophagous Fungi: Ecology, Diversity and Geographical Distribution. In: Biocontrol Agents of Phytonematodes, Editor(s): Askary, T.H., Martinelli, P.R.P. CAB International. P 126.
Mora M.A.E., Conteiro A.M., Castillo C. and Fraga M.E. (2017) Classification and infection mechanism of entomopathogenic fungi. Arq. Inst. Biol. 84: 110. https://doi.org/10.1590/1808-1657000552015
Ghewande M.P. and Nandagopal V. (1997) Integrated pest management in groundnut (Arachishypogaea L.) in India. Integr. Pest Manag. Rev., 2: 1-15. https://doi.org/10.1023/a:1018488326980
Glare T., Caradus J., Gelernter W., Jackson T., Keyhani N., Köhl J., et al. (2012). Have biopesticides come of age? Trends Biotechnol. 30: 250–258. https://doi.org/10.1016/j.tibtech.2012.01.003
Jagannathan S.V., Manemann E.M., Rowe S.E., Callender M.C. and Soto W. (2021) Marine actinomycetes, new sources of biotechnological products. Mar. Drugs, 19: 365.
Kalia V.C., Patel S.K.S., Kang Y.C. and Lee J.K. (2019) Quorum sensing inhibitors as antipathogens: Biotechnological applications. Biotechnol. Adv., 37: 68–90.
Knowles A. (2008) Recent developments of safer formulations of agrochemicals. Environmentalist, 28(1):35–44. https://doi.org/10.1007/s10669-007-9045-4
Köhl J., Kolnaar R. and Ravensberg W.J. (2019) Mode of action of microbial biological control agents against plant diseases: Relevance beyond efficacy. Front. Plant Sci., 10: 845.
Köhl J., Postma J., Nicot P., Ruocco M. and Blum B. (2011) Stepwise screening of microorganisms for commercial use in biological control of plant-pathogenic fungi and bacteria. Biol. Control., 57: 1–12.
Kumari S., Kumar S.C., Jha M.N., Kant R., Upendra S. and Kumar P. (2014) Microbial pesticide: a boom for sustainable agriculture. Int. J. Sci. Eng. Res., 5(6): 1394-1397.
Lin F., Mao Y., Zhao F., Idris A.L., Liu Q., Zou S., Guan X. and Huang, T. (2023) Towards Sustainable Green Adjuvants for Microbial Pesticides: Recent Progress, Upcoming Challenges and Future Perspectives. Microorganisms, 11: 364. https://doi.org/10.3390/ microorganisms11020364
Lindsey, A.P.J., Murugan S. and Renitta R.E. (2020) Biocatalysis and agricultural biotechnology microbial disease management in agriculture: Current status and future prospects. Biocatalysis Agric. Biotechnol., 23: 1-12.
Matyjaszczyk E. (2015) Products containing microorganisms as a tool in integrated pest management and the rules of their market placement in the European Union. Pest Manag. Sci. 71:1201–1206.
Mazid S., Kalita J.C. and Rajkhowa R.C. (2011) A review on the use of biopesticides in insect pest management. Int. J. Sci. Adv. Technol. 1: 169–178.
Mishra J., Tewari S., Singh S. and Arora NK (2015) Biopesticides: Where we stand?, In: NK Arora (ed), Plant microbes symbiosis: applied facets, Springer, New Delhi. Pp 37-75.
Montesinos E. (2003) Development, registration and commercialization of microbial pesticides for plant protection. Int. Microbiol., 6: 245–252.
Montesinos E. and Bonaterra A. (2009) Microbial Pesticides. In Encyclopedia of Microbiology, 3rd ed.; Schaechter, M., Ed.; Elsevier: Amsterdam, The Netherlands, pp. 110–120.
Nakayinga R., Makumi A., Tumuhaise V. and Tinzaara W. (2021) Xanthomonas bacteriophages: a review of their biology and biocontrol applications in agriculture BMC Microbiol., 21:291 https://doi.org/10.1186/s12866-021-02351-7
Nawaz M., Mabubu J.I. and Hua H. (2016) Current status and advancement of biopesticides: Microbial and botanical pesticides. J. Entomol. Zool. Stud., 4: 241-246.
Nielsen A.L., Spence K.O. and Lewis, E.E. (2008) Efficacy patterns of biopesticides used in potting media. Biopesticide Int., 4: 87-101.
Nuss D.L. (1992) Biological control of chestnut blight: An example of virus-mediated attenuation of fungal pathogenesis. Microbiol. Rev., 56(4): 561-576.
Nuss D.L. (2011) Mycoviruses, RNA silencing, and viral RNArecombination. In: Advances in virus research. Elsevier: pp: 25-48.
Pal K.K. and Gardener B.M. (2006) Biological control of plant pathogens. Plant Health Instr., 1–25. https://doi.org/10.1094/ PHI-A-2006-1117-02.
Pathak D., Yadav R. and Kumar M. (2017) Microbial pesticides: development, prospects and popularization in India. In: Singh, D.P. et al., (Eds.), Plant Microbe Interactions in Agro-Ecological Perspectives. Springer, New Delhi, pp. 455–471.
Prabha S., Yadav Ashwani A. Kumar and Yadav et al. (2016) Biopesticides - an alternative and eco-friendly source for the control of pests in agricultural crops. Plant Archives, 16: 902-906.
Senthilkumar M., Anandham R., Krishnamoorthy R. (2020) Chapter 41 - Paecilomyces, Editor(s): N. Amaresan, M. Senthil Kumar, K. Annapurna, Krishna Kumar, A. Sankaranarayanan, In: Beneficial Microbes in Agro-Ecology, Academic Press, Pp 793-808.
Singh H.B. (2022) Current Status and Recent Developments in Microbial Pesticide Use in India. Indian J. Plant Genet. Resour., 35(3): 369-374. https://doi.org/10.5958/0976-1926.2022.00102.4
Tadros F. (2005) Applied surfactants, principles, and applications. Wiley-VCH Verlag GmbH and Co. KGaA, Weinheim, pp 187–256.
Tariq M., Khan A., Asif M., Khan F., Ansari T., Shariq M. and Siddiqui M.A. (2020) Biological control: a sustainable and practical approach for plant disease management. Acta Agric. Scand. -B Soil Plant Sci., 70:6, 507-524, https://doi.org/ 10.1080/09064710.2020.1784262
Thakur N., Kaur S., Tomar P,, Thakur S. and Yadav A.N. (2020) Chapter 15 - Microbial biopesticides: Current status and advancement for sustainable agriculture and environment. Editor(s): Ali Asghar Rastegari, Ajar Nath Yadav, Neelam Yadav, In: New and Future Developments in Microbial Biotechnology and Bioengineering, Elsevier, Pp. 243-282.
Tripathi Y.N., Divyanshu K., Kumar S., Jaiswal L.K. and Khan A. et al. (2020) Biopesticides: Current Status and Future Prospects in India. In: Bioeconomy for Sustainable Development, Keswani, C. (Eds.), Springer, Singapore, ISBN-13: 978-981-13-9431-7, pp: 79-109
Vosátka M., Látr A., Gianinazzi S. and Albrechtová J. (2012) Development of arbuscular mycorrhizal biotechnology and industry: Current achievements and bottlenecks. Symbiosis, 58:29–37, https://doi.org/ 10.1007/s13199-012-0208-9.
Whipps J.M. (2001) Microbial interactions and biocontrol in the rhizosphere. J. Exp. Bot., 52:487–511.
Yadav I.C. and Devi N.L. (2017) Pesticides classification and its impact on human and environment. Environ Sci Engg 6: Toxicology.
Zhang D.X. and Nuss D. L. (2016) Engineering super mycovirus donor strains of chestnut blight fungus by systematic disruption of multilocusvic genes. Proc. Nat. Aca. Sci: 201522219.
Microbial Formulations: An Alternative to Chemical Pesticides
DOI: https://doi.org/10.56669/VNFT9299
ABSTRACT
Biological pesticides, derived from biocontrol agents, manage agricultural pests and diseases, and boost crop production. These pesticides come from microorganisms (metabolites), plants (exudates, essential oils, and extracts from bark, root, and leaves), and biologically derived nanoparticles (like copper and zinc-based nanoparticles). In India, key microbial formulations include Bacillus thuringiensis, Trichoderma, NPV, and neem-based insecticides. These microbes release specific metabolites that protect plants from pests and diseases, making them highly effective. Unlike synthetic pesticides, microbial formulations are specific in action, cost-effective, and environmentally friendly, leaving no harmful residues. The use of biopesticides in India has increased, with consumption rising to 8,647 metric tons in 2020-21 and 8,898 metric tons in 2021-22. Despite their benefits, field-level adoption remains limited. Addressing challenges in delivery and consistent performance is crucial for broader adoption.
Keywords: Microbial formulation, Biopesticides, Integrated Pest Management, Chemical pesticides
INTRODUCTION
In modern agriculture, pesticides have been crucial for boosting crop yields, regulating plant growth, and controlling pests and diseases. However, the global economy and heightened concerns over chemical pesticide residues have led to stricter trade standards, especially in Europe. Ideally, pesticides should target pests effectively without harming non-target species, including humans. Biopesticides present a promising alternative, effective in pest management and promoting sustainable agriculture (Prabha et al., 2016). Despite their potential, technical challenges have hindered their widespread adoption (Nielsen et al., 2008). In the European Union, Integrated Pest Management (IPM) policies emphasize innovative pest control methods, particularly biological pesticides derived from living microorganisms or natural products (Chandler et al., 2011). These biopesticides, sourced from naturally occurring bacteria, fungi, protozoa, yeasts, and viruses, are recognized for their target specificity, environmental safety, efficacy, biodegradability, and suitability in integrated disease management programs. They include various types, such as microbial formulations, plant-incorporated protectants, and bio-chemicals, playing a crucial role in IPM strategies that prioritize eco-friendly and safer alternatives to chemical pesticides. Since their introduction, many biopesticide products have been developed and some have gained market prominence.
This paper discusses the current status, potential to replace chemical pesticides, future prospects, and the challenges associated with biopesticides in plant pest and disease management.
Can microbe based pesticides be the option of chemical pesticides?
Several factors indicate that microbe based pesticides are excellent alternatives to synthetic pesticides. Specifically, they are highly effective, target-specific and have fewer environmental risks. Microbial formulations can come from a wide diversity of organisms and many products have been released and registered in the agromarket. Microbial formulations allow for a sustainable approach for improved crop production, which should increase their use and popularity in the coming years (Mishra et al., 2015). Moreover, opinions about microbial formulation use have begun to change because of the recent recognition of the environmental consequences of chemical pesticides. Other factors contributing to their increased use are advances in activity spectra spots and improved options for delivery and application (Glare et al., 2012). Current research into microbial formulations focuses on the improvement of their mode of action, including mechanisms to replace the use of chemical pesticides in integrated disease management programe (Nawaz et al., 2016). IPM is a method to incorporate chemical, biological and physical methods for pest and disease control (Ghewande and Nandagopal, 1997). The main challenges of new biological pesticides in the growth and utilization are how to market or promote it (Tripathi et al., 2020) and how to enhance the stability and residual action of microbial formulation (Damalas and Koutroubas, 2018). Previously, there were several methods applied for handling pests, such as traditional methods, chemical methods and biological methods (Lindsey et al., 2020).
Microbial formulations
Microbial formulations are compounds used to control plant pests and diseases that are derived from beneficial microorganisms or the metabolic products they produce. The microbiome or microorganisms as a biological pesticide is another option that has been shown to be effective against insect pests and diseases. Therefore, switching to biopesticides is absolutely necessary to replace these chemical pesticides, as they offer a more sustainable and environmentally friendly alternative, reducing the harmful effects on ecosystems, human health, and beneficial organisms while addressing the growing issue of pesticide resistance (Kumari et al., 2014). Microbial formulations and biopesticides (PGPR, bio stimulants) based on living microbes and their bioactive components have been intensively studied, published, and promoted in order to efficiently manage plant pests and diseases and stimulate plant growth. These are done by applying large varieties of microorganisms including fungi, bacteria viruses, protozoans, and nematodes as microbial formulations (Singh, 2022). Pseudomonas fluorescens, Bacillus subtilis, Beauvaria bassiana, Gliocladium spp., Trichoderma, and Metarrhizium anisopliae are among the nine microbes listed in a schedule published in the Indian Gazette on March 26, 1999, as an amendment to the Insecticides Act, 1968, for the commercial production of biopesticides. The Insecticide Act of 1968 included 26 additional microorganisms on its list of targets for the development of microbial biopesticides (Singh, 2022).
Why microbial formulation?
Microbial formulations are species-specific and do not harm other beneficial organisms (Kumari et al., 2014). They may occur naturally in the environment or be genetically modified for the production of toxicants, which exhibits species-specific reactions. Today's commonly used microbial formulations are microorganisms that have a harmful effect on pest and pathogen populations. These biopesticides are also effective in small amounts and dissolve quickly, resulting in a poorer revelation and more environmentally friendly technique (Thakur et al., 2020). These biopesticides break down quickly, resulting in reduced residual effects and a more environmentally friendly approach. Although traditional agrochemical pesticides have been effective in controlling pests and diseases for many years, their long-term use has led to diminishing efficacy. This is due to the rise of pesticide-resistant pests and diseases and the growing concern over environmental and human health risks, leading to the suspension or deregulation of many chemical pesticides. Consequently, there is an urgent need for eco-friendly alternatives. Higher quality and increased quantity of agricultural products are examples of pest management strategy advancements. As a result, there is a critical need for the development of beneficial, sustainable, and non-toxic biopesticides. To avoid the negative effects of chemical pesticides, priority should be given to the use of biopesticides against pests and diseases (Mazid et al., 2011 and Pathak et al., 2017).
Types and function of microbial formulations
Bacteria
For decades, bacteria have been introduced into soil, seeds, roots, and other plant structures to enhance plant growth and development. These bacteria promote beneficial relationships such as nitrogen fixation, hazardous chemical degradation, plant growth promotion, and biological control of pathogenic microorganisms. Numerous bacterial genera, including Acinetobacter, Agrobacterium, Alcaligenes, Arthrobacter, Azospirillum, Azotobacter, Bacillus, Bradyrhizobium, Frankia, Pantoea, Pseudomonas, Rhizobium, Serratia, Stenotrophomonas, Streptomyces, Enterobacter, Erwinia, Thiobacillus, and Xanthomonas, have been reported to protect plants against fungal and bacterial pathogens (Whipps 2001, Montesinos and Bonaterra, 2009). Among these, Pseudomonas spp., Bacillus spp., and Streptomyces spp. have been intensively studied and registered as commercial products.
Research has shown that these bacteria can prevent various plant diseases, including nematode infestations, fungal infections, and bacterial diseases (Tariq et al., 2020). Bacterial biocontrol agents employ a wide range of strategies to protect plants against pathogens. They can compete for resources and space, interfere with pathogen pathogenicity, and secrete antimicrobial compounds. Many bacterial biocontrol agents release antimicrobial metabolites such as lipopeptides, bacteriocins, antibiotics, biosurfactants, cell-wall disintegrating enzymes, or microbial volatile compounds. They can also disrupt the quorum sensing (QS) systems of pathogens, interfering with signal molecules used to initiate infections (Kalia et al., 2019; Bonaterra et al., 2022). Table 1 shows a list of commercially available bacterial bioformulations for pest management of food crops worldwide.
Fungi
Fungi have evolved mechanisms to inhibit the growth of pathogenic fungi detrimental to plant development. The focus on developing fungal strains as biocontrol agents for plant diseases has been significant, with Trichoderma spp. being extensively studied. These fungi, particularly Trichoderma viride and Trichoderma harzianum, are used in various products due to their ability to reduce abiotic and biotic stress on host plants by suppressing a range of plant diseases and nematodes. T. viride is effective against soil-borne pathogens such as Fusarium, Sclerotium, Rhizoctonia, and Pythium, while T. harzianum also targets Botrytis, Gaeumannomyces, Sclerotinia, Verticillium, and wood-rot fungi (Vosátka et al., 2012).
Other fungal species, including Gliocladium and Aspergillus, exhibit antagonistic activities against pathogens like Alternaria, Pythium, Aspergillus, Fusarium, Rhizoctonia, Phytophthora, Botrytis, Pyricularia, and Gaeumannomyces (Pal and Gardener, 2006). Nematophagous fungi have shown promise as biological agents for nematode management, with key genera including Arthrobotrys, Duddingtonia flagrans, Monacrosporium, and Dactylaria (Senthilkumar et al., 2020). Paecilomyces lilacinus, a soil-borne fungus prevalent in tropical and subtropical regions, has significant potential as a biocontrol agent against plant-parasitic nematodes.
Entomopathogenic fungi, which infect and kill insects by penetrating their cuticle, are divided into several groups: Ascomycota, Zygomycota, Deuteromycota, Oomycota, and Chytridiomycota (Esparza-Mora et al., 2017). Most commercial products are based on three entomopathogenic fungal genera: Beauveria bassiana, Metarhizium, and Paecilomyces lilacinus. These fungi produce conidia that adhere to the insect cuticle and release enzymes, such as fungal protease, that break down the cuticle and allow the fungus to penetrate and kill the insect by consuming its tissues (Brunner-Mendoza et al., 2019; Dara, 2017). Table 2 shows a list of commercially available fungal formulations for targeted pathogens.
Actinomycetes
Actinomycetes can be used as a biocontrol agent on phytopathogenic fungus as an alternative to applying synthetic fungicides in recent years (Boukaew et al., 2022). Actinomycetes are Gram-positive bacteria that can live in a range of environments with varying humidity, pH levels, and temperatures. Actinomycetes have been isolated from a variety of habitats, including wetlands, terrestrial, marine, and hypersaline conditions (Jagannathan et al. 2021).
Competence for space and nutrients, production of antibiotics, siderophores, lytic enzymes, volatile organic compounds (VOCs), and host resistance induction are the principal antagonistic methods used by Actinomycetes to suppress phytopathogenic fungi. Actinomycetes also aid in the production of phytohormones, atmospheric nitrogen fixation, mineral solubilization, and other processes that aid in the growth and development of plants. Actinomycetes have been the subject of several research, however due to their utility as biocontrol agents in agriculture and their antagonistic processes to phytopathogenic fungi, few of these studies have concentrated on Actinomycetes isolated from different environments.
Actinomycetes as biocontrol agents
The significance of using Actinomycetes as biocontrol agents is explained by their inherent beneficial traits, which include the following: (1) they don't affect human or animal health; (2) they're not poisonous to plants; (3) they increase plant yield; and (4) they reduce the need for synthetic fungicides. Streptomyces has received the most research attention among the several genera since it is simple to isolate. Bacteria grow faster than Actinomycetes. To acquire desired Actinomycetes in culture media, growth enhancement approaches should be used. These methods are based on the pretreatment of samples using wet, chemical, and other pretreatment methods as well as selected isolation media and soil with calcium carbonate. By incorporating biostimulants and organic fertilisers like compost and vermicompost, one can encourage the populations of Actinomycetes in the soil. The maximum antagonistic activity was demonstrated by S. sampsonii and S. flavovariabilis isolates from soil enriched with vermicompost towards Rhizoctonia solani, Alternaria tenuissima, Aspergillus niger, and Penicillium expansum. Actinomycetes populations in the soil were also increased by adding Brassica napus and Brassica rapa leaf residue to the soil. The decline in the R. solani wilt disease was strongly correlated with the rise in the Actinomycetes population (Ascencion et al., 2015). Table 3 shows a list of commercially available Actinomycetes based formulations for targeted diseases.
Mycovirus
Fungal viruses, also known as mycoviruses, are not known to kill or infect their hosts, but they are known to alter gene expression, morphology, growth, sporulation, pathogenicity, and interactions with other species. The possibility that mycoviruses could be a useful tool for biocontrol of fungi has sparked research into mycoviruses. Mycoviruses are known to slow down the development rate and/or virulence of their hosts.
Mycoviruses as biocontrol agents
The majority of plant diseases are brought on by fungi. Fungicide application is a typical technique for managing fungal phytopathogenic diseases. Continuous use of fungicides results in the development of fungicide-resistant strains, which has potential negative impacts on both humans and the environment. The use of biocontrol agents as an alternative to chemical control has grown significantly in relevance in recent years. Mycoviruses could also be employed as biocontrol agents. Nuss (1992) was the first to demonstrate the use of a dsRNA virus as a biocontrol agent against chestnut blight. The production of a cDNA copy of the viral RNA associated with the hypovirus and its transformation into a virulent fungal strain turned the compatible, virulent strain into a hypo virulent strain. Chestnut fungus was successfully managed in Europe with the use of CHV-1 cDNA, but in North America, the fungus had a wider genetic range and was more difficult to manage (Nuss, 1992). There are barriers to the viruses' successful transmission when they are transmitted by hyphal contact. The beneficial infection of viruses that decreases the pathogenicity of plant pathogenic fungi is known as hypovirulence. The most prevalent mycovirus trait, hypo virulence, is utilized to biologically control a number of plant diseases, including Cryphonecteria parasitica (chestnut blight), woody plant white root rot, rice blast, and a variety of soil-borne infections (Zhang and Nuss, 2016).
Mechanism
The exact mechanism underlying hypo pathogenicity is still unknown, although numerous theories have been offered by various researchers, including signal transduction pathways, RNA silencing of the fungus, and counter-silencing processes exploited by the hypovirus (Nuss, 2011). There are also other documented pathways for low virulence, including nuclear alterations, plasmids, and mutations of the mitochondria. The expansion of the host range of mycoviruses without the challenges of vegetative incompatibility is made possible by the production of cDNA infectious clones and other transformation techniques (Chen et al., 1994; Abid et al., 2018).
Bacteriophages
Viruses that infect and multiply in bacteria are known as phages. Temperate and lytic pathways are used in phage replication cycles, with the lytic pathway being the easier and more essential pathway for use in phage biocontrol. The challenges with antibiotic resistance, ineffective cultural practices, copper-based pesticides, and environmental chemical contamination have sparked global interest in Xanthomonas phage research and the use of biocontrol in agriculture (Nakayinga et al., 2021).
Bacteriophage-based biocontrol for the phytopathogen Xanthomonas has a long history, going all the way back to the early nineteenth century, when a filtrate of decaying cabbage prevented the spread of a disease known as Xanthomonas campestris pv. campestris. A few decades later, similar biocontrol efficacy was noted with phage-containing lysates that prevented Xanthomonas campestris pv. pruni-caused bacterial spot disease in peaches. Phage applications have advanced from in-vitro studies to field testing in a number of instances. Against bacterial leaf blight of rice (X. oryzae pv. oryzae), leaf blight of onion (X. axonopodis pv. allii), bacterial spot of tomato (X. campestris pv. vesicatoria), citrus canker (X. axonopodis pv. citri) and citrus bacterial spot (X. axonopodis pv. citrumelo), number of bacteriophages were isolated and evaluated. It has been demonstrated that two Xanthomonas phage products made by AgriPhage are effective in managing the pathogens responsible for citrus canker and tomato & pepper spot disease (Nakayinga et al., 2021).
Application of microbial formulations in plant disease management
The production of microbial formulations, which are used to manage a variety of diseases in agricultural crops all over the world, involves the use of fungi, bacteria, actinomycetes, bacteriophages, mycoviruses, and nematodes (Yadav and Devi, 2017). They are applied by spraying, soaking, or directly applying them to the roots in the form of a tablet, coating the seeds or treating the roots before planting, as well as by various insects (Montesinos and Bonaterra, 2009).
A variety of microbial formulations are available, including wettable powders, suspensions, oil suspensions, water-dispersible granules, and suspension seed coatings (Table 4). However, microbial formulation manufacturing is more difficult than chemical pesticide production (Lin et al., 2023). The microorganisms in pesticides are more susceptible to outside environmental conditions during preparation, such as UV radiation, temperature, and humidity.
Formulation
The term "formulation" describes the process of creating a product from an active ingredient by adding specific active (functional) and inert (inactive) substances.
Principles of formulation
In order to maximize lifespan in storage, optimize application to the target, and protect the organisms after application, prepared organisms are suspended in a suitable carrier that is supplemented by additives. They differ from chemical active components in that they are particulate, living, or proteinous in nature, making them more susceptible to environmental factors and storage conditions (Bergis and Jones, 1998).
There are two types of biopesticide formulations based on their physical states:
• Liquid formulations; and
• Dry formulations.
Liquid formulations may be water, oil or polymer based or the combination of all. Water based formulations like suspension concentrate (SC), capsule suspension (CS) and suspo-emulsions (SE) requires the addition of inert materials viz., surfactants, stickers, stabilizers, antifreezing agents, coloring agents and nutritive materials.
Dry formulations like dusts (DP), granules (G), micro granules (MG), wettable powders (WP), and water dispersible granules (WG) are prepared by adding wetting agents, binding agents, dispersants etc., using a technologies like spray drying, air drying or freeze drying (Tadros, 2005; Brar,2006; Knowles, 2008).
Classifications and functions of adjuvants used for microbial formulations
The four basic categories of adjuvants employed in the production of microbial formulations are surfactants, transporters, protective agents, nutritional adjuvants etc.
Carriers
The basic function of a carrier is to serve as a tiny container or diluent for the active components of microbial formulations. High concentration powders, wettable powders, or granules can be produced using carriers with strong adsorption capabilities, such as diatomite, attapulgite, silica, and bentonite. To create low-concentration powders, diluents and fillers such as talc, pyrophyllite, sepiolite, and clay materials with low or moderate adsorption capabilities are typically utilised. Biodegradable and environmentally safe materials such as chitosan, carbon nanotube nanocomposite, biodiesel, and plant based substances like bagasse, corn bagasse, chaff powder, tobacco powder, and walnut shell powder are used as carriers. Though plant-based carriers have the property of absorbing ultraviolet rays (UV), their use in commercial production is very rare.
Surfactants
Surfactants are crucial in preserving the physical stability of biopesticides over the long term and enhancing their biological capabilities. Generally, microbial formulations comprise one or more surfactants. This surfactant helps to increase cost-effectiveness, save energy and raw materials, and increase processing efficiency.
Protective Agents
UV protection agents
UV ray absorbers and anti-oxidative UV protection agents are the two categories of UV protection agents utilized in the manufacturing of microbial formulations. Milk, optical brighteners, lignosulfonates, and locust toxin are examples of UV ray absorbers that may absorb the UV portion of sunlight and fluorescent light sources without transforming themselves. Rubus oil, hemp seed oil, kojic acid, hydroxykynurenic acid, flavonoids, and lecithin are anti-oxidative UV protection agents which have strong antioxidative effect (Lin etal, 2023).
Nutritional adjuvants
Microorganisms require water, inorganic salts, carbon sources, nitrogen sources, and growth nutrients in order to function normally throughout the growth phase. Nutritional adjuvants can give nutrition to the microorganisms that make up microbial formulations so they can reproduce more easily, grow and proliferate more quickly in the field.
Challenges and opportunities for commercialization of microbial formulation
Synthetic chemical pesticides were formerly the mainstay of crop protection, but their use is now declining due to new rules and restrictions, as well as the evolution of pest and pathogen resistance. As a result, a new pest and disease management strategy is required. A microbial formulation is an ideal solution for replacing synthetic chemical pesticides based on natural or living microorganisms. Microbial formulations have acquired importance as an essential part of IPM due to their economic feasibility and sustainability in comparison to chemical synthetic pesticides (Birch 2011). Microbial formulations are able to overcome the limitations of conventional pesticides as part of IPM programs since they are host-specific, biodegradable, and less toxic to beneficial microbes (Matyjaszczyk, 2015).
The effectiveness of microbial formulations in combating plant diseases depends on the microbial agent (mechanism of action, conditioning, dose, methods of application), the host (cultivar type, physical characteristics), the targeted plant pathogens (sensitivity), and environmental factors (biotic and abiotic factors, chemical residues, nutrient availability, temperature, and moisture). The variable factors like location, time period, host plants and their interactions may alter the effectiveness of microbial agents. Lack of efficacy and varied field performance have frequently been documented. The effectiveness and consistency of biological control must therefore be compared to those of conventional chemical fungicide and bactericide treatments under sufficiently broad production settings in fields representing various environments and agricultural practices (Montesinos, 2003; Köhl et al., 2011; and Bonaterra, 2022).
The demand for safer plant pest and disease control products leads to a preference for microbial biopesticide formulations with effective antagonistic activity and good stability. Biopesticides offer environmentally benign alternatives to synthetic pesticides, but they encounter a number of challenges during their development, formulation, and use. Maximum attention is required to maintain the microbial population and efficacy throughout the entire use of biopesticides because they typically contain living organisms. The commercialization of biopesticides could benefit greatly from research into their manufacture and formulation. As their application methods advance and less expensive inert components are acknowledged for various formulations, it looks that biopesticides will be used more widely in the future.
The introduction of new adjuvants demonstrated a notable increase in microbial activity and suggested a new avenue of research. The stability of the product, its longer shelf life, and the performance of the microorganisms in the field may all be improved by choosing the right formulation. In the future, formulation products should have a better balance between production cost and efficiency, as is the case with biopesticides, which provide a more balanced plant protection product application (El-Sayed, 2005; Rao et al., 2007; Glare et al., 2012; Khater, 2012). The formulation type could evolve from dusts to granules, from suspension concentrates and wettable powders to water-dispersible granules, and from products based on a single bacterium to formulations based on microbial consortiums.
CONCLUSION
The fact that components of microbial formulations are widely regarded as safe and are target-specific means that they generally have no negative effects on the environment and human beings. Compared to chemical pesticides, the use of them produces fewer greenhouse gas emissions. Moreover, a wide range of organisms can be used to develop microbial biopesticides, to tackle the issue of resistance and guarantee sustainability. It may be difficult for producers, marketers, and end users to manage their storage and transportation because different microorganisms employed as biopesticides may require varied storage conditions. Therefore, more study is required to ensure that microbial formulations have a sustainable and extended shelf life.
REFERENCES
Ascencion L.C., Liang W.J. and Yen T.B. (2015) Control of Rhizoctonia solani damping-off disease after soil amendment with dry tissues of Brassica results from increase in Actinomycetes population. Biol. Control 82: 21–30.
Birch A.N.E. (2011) How agro-ecological research helps to address food security issues under new IPM and pesticide reduction policies for global crop production systems. J. Exp. Bot., 62: 3251–3261.
Bonaterra A., Badosa E., Daranas N., Francés J., Roselló G. and Montesinos E. (2022) Bacteria as Biological Control Agents of Plant Diseases. Microorganisms 10: 1759. https://doi.org/10.3390/ microorganisms10091759
Boukaew S., Yossan S., Cheirsilp B. and Prasertsan P. (2022) Impact of environmental factors on Streptomyces spp. metabolites against Botrytis cinerea. J. Basic Microbiol., 62, 611–622.
Brar S.K., Tyagi V.R.D. and Valero JR (2006) Recent advances in downstream processes and formulations of Bacillus thuringiensis based biopesticide. Process Biochem., 41: 323–342.
Brunner-Mendoza C., Reyes-Montes M.R., Moonjely S., Bidochka M.J. and Toriello C. (2019) A review on the genus Metarhizium as an entomopathogenic microbial biocontrol agent with emphasis on its use and utility in Mexico. Biocontrol. Sci. Tech. 29: 83102.
Chandler D., Bailey A.S., Tatchell G.M., Davidson G. and Greaves J. et al. (2011) The development, regulation and use of biopesticides for integrated pest management. Philosophical Trans. Royal Society B: Biol. Sci., 366: 1987-1998. DOI: 10.1098/rstb.2010.0390
Chen B., Choi G.H. and Nuss D.L. (1994) Attenuation of fungal virulence by synthetic infectious hypovirus transcripts. Science, 264(5166): 1762-1764.
Damalas C.A. and Koutroubas S.D. (2018) Current status and recent developments in biopesticide use. Agriculture, 8: 1-6.
Dara S.K. (2017) Entomopathogenic microorganisms: modes of action and role in intregrated pest management. UC ANR e-Journal of Entomology and Biologicals, 20 May 2017. https://ucanr.edu/blogs/blogcore/postdetail.cfm?postnum 5 24119.
Dasgupta M.K. and Khan M.K. (2015) Nematophagous Fungi: Ecology, Diversity and Geographical Distribution. In: Biocontrol Agents of Phytonematodes, Editor(s): Askary, T.H., Martinelli, P.R.P. CAB International. P 126.
Mora M.A.E., Conteiro A.M., Castillo C. and Fraga M.E. (2017) Classification and infection mechanism of entomopathogenic fungi. Arq. Inst. Biol. 84: 110. https://doi.org/10.1590/1808-1657000552015
Ghewande M.P. and Nandagopal V. (1997) Integrated pest management in groundnut (Arachishypogaea L.) in India. Integr. Pest Manag. Rev., 2: 1-15. https://doi.org/10.1023/a:1018488326980
Glare T., Caradus J., Gelernter W., Jackson T., Keyhani N., Köhl J., et al. (2012). Have biopesticides come of age? Trends Biotechnol. 30: 250–258. https://doi.org/10.1016/j.tibtech.2012.01.003
Jagannathan S.V., Manemann E.M., Rowe S.E., Callender M.C. and Soto W. (2021) Marine actinomycetes, new sources of biotechnological products. Mar. Drugs, 19: 365.
Kalia V.C., Patel S.K.S., Kang Y.C. and Lee J.K. (2019) Quorum sensing inhibitors as antipathogens: Biotechnological applications. Biotechnol. Adv., 37: 68–90.
Knowles A. (2008) Recent developments of safer formulations of agrochemicals. Environmentalist, 28(1):35–44. https://doi.org/10.1007/s10669-007-9045-4
Köhl J., Kolnaar R. and Ravensberg W.J. (2019) Mode of action of microbial biological control agents against plant diseases: Relevance beyond efficacy. Front. Plant Sci., 10: 845.
Köhl J., Postma J., Nicot P., Ruocco M. and Blum B. (2011) Stepwise screening of microorganisms for commercial use in biological control of plant-pathogenic fungi and bacteria. Biol. Control., 57: 1–12.
Kumari S., Kumar S.C., Jha M.N., Kant R., Upendra S. and Kumar P. (2014) Microbial pesticide: a boom for sustainable agriculture. Int. J. Sci. Eng. Res., 5(6): 1394-1397.
Lin F., Mao Y., Zhao F., Idris A.L., Liu Q., Zou S., Guan X. and Huang, T. (2023) Towards Sustainable Green Adjuvants for Microbial Pesticides: Recent Progress, Upcoming Challenges and Future Perspectives. Microorganisms, 11: 364. https://doi.org/10.3390/ microorganisms11020364
Lindsey, A.P.J., Murugan S. and Renitta R.E. (2020) Biocatalysis and agricultural biotechnology microbial disease management in agriculture: Current status and future prospects. Biocatalysis Agric. Biotechnol., 23: 1-12.
Matyjaszczyk E. (2015) Products containing microorganisms as a tool in integrated pest management and the rules of their market placement in the European Union. Pest Manag. Sci. 71:1201–1206.
Mazid S., Kalita J.C. and Rajkhowa R.C. (2011) A review on the use of biopesticides in insect pest management. Int. J. Sci. Adv. Technol. 1: 169–178.
Mishra J., Tewari S., Singh S. and Arora NK (2015) Biopesticides: Where we stand?, In: NK Arora (ed), Plant microbes symbiosis: applied facets, Springer, New Delhi. Pp 37-75.
Montesinos E. (2003) Development, registration and commercialization of microbial pesticides for plant protection. Int. Microbiol., 6: 245–252.
Montesinos E. and Bonaterra A. (2009) Microbial Pesticides. In Encyclopedia of Microbiology, 3rd ed.; Schaechter, M., Ed.; Elsevier: Amsterdam, The Netherlands, pp. 110–120.
Nakayinga R., Makumi A., Tumuhaise V. and Tinzaara W. (2021) Xanthomonas bacteriophages: a review of their biology and biocontrol applications in agriculture BMC Microbiol., 21:291 https://doi.org/10.1186/s12866-021-02351-7
Nawaz M., Mabubu J.I. and Hua H. (2016) Current status and advancement of biopesticides: Microbial and botanical pesticides. J. Entomol. Zool. Stud., 4: 241-246.
Nielsen A.L., Spence K.O. and Lewis, E.E. (2008) Efficacy patterns of biopesticides used in potting media. Biopesticide Int., 4: 87-101.
Nuss D.L. (1992) Biological control of chestnut blight: An example of virus-mediated attenuation of fungal pathogenesis. Microbiol. Rev., 56(4): 561-576.
Nuss D.L. (2011) Mycoviruses, RNA silencing, and viral RNArecombination. In: Advances in virus research. Elsevier: pp: 25-48.
Pal K.K. and Gardener B.M. (2006) Biological control of plant pathogens. Plant Health Instr., 1–25. https://doi.org/10.1094/ PHI-A-2006-1117-02.
Pathak D., Yadav R. and Kumar M. (2017) Microbial pesticides: development, prospects and popularization in India. In: Singh, D.P. et al., (Eds.), Plant Microbe Interactions in Agro-Ecological Perspectives. Springer, New Delhi, pp. 455–471.
Prabha S., Yadav Ashwani A. Kumar and Yadav et al. (2016) Biopesticides - an alternative and eco-friendly source for the control of pests in agricultural crops. Plant Archives, 16: 902-906.
Senthilkumar M., Anandham R., Krishnamoorthy R. (2020) Chapter 41 - Paecilomyces, Editor(s): N. Amaresan, M. Senthil Kumar, K. Annapurna, Krishna Kumar, A. Sankaranarayanan, In: Beneficial Microbes in Agro-Ecology, Academic Press, Pp 793-808.
Singh H.B. (2022) Current Status and Recent Developments in Microbial Pesticide Use in India. Indian J. Plant Genet. Resour., 35(3): 369-374. https://doi.org/10.5958/0976-1926.2022.00102.4
Tadros F. (2005) Applied surfactants, principles, and applications. Wiley-VCH Verlag GmbH and Co. KGaA, Weinheim, pp 187–256.
Tariq M., Khan A., Asif M., Khan F., Ansari T., Shariq M. and Siddiqui M.A. (2020) Biological control: a sustainable and practical approach for plant disease management. Acta Agric. Scand. -B Soil Plant Sci., 70:6, 507-524, https://doi.org/ 10.1080/09064710.2020.1784262
Thakur N., Kaur S., Tomar P,, Thakur S. and Yadav A.N. (2020) Chapter 15 - Microbial biopesticides: Current status and advancement for sustainable agriculture and environment. Editor(s): Ali Asghar Rastegari, Ajar Nath Yadav, Neelam Yadav, In: New and Future Developments in Microbial Biotechnology and Bioengineering, Elsevier, Pp. 243-282.
Tripathi Y.N., Divyanshu K., Kumar S., Jaiswal L.K. and Khan A. et al. (2020) Biopesticides: Current Status and Future Prospects in India. In: Bioeconomy for Sustainable Development, Keswani, C. (Eds.), Springer, Singapore, ISBN-13: 978-981-13-9431-7, pp: 79-109
Vosátka M., Látr A., Gianinazzi S. and Albrechtová J. (2012) Development of arbuscular mycorrhizal biotechnology and industry: Current achievements and bottlenecks. Symbiosis, 58:29–37, https://doi.org/ 10.1007/s13199-012-0208-9.
Whipps J.M. (2001) Microbial interactions and biocontrol in the rhizosphere. J. Exp. Bot., 52:487–511.
Yadav I.C. and Devi N.L. (2017) Pesticides classification and its impact on human and environment. Environ Sci Engg 6: Toxicology.
Zhang D.X. and Nuss D. L. (2016) Engineering super mycovirus donor strains of chestnut blight fungus by systematic disruption of multilocusvic genes. Proc. Nat. Aca. Sci: 201522219.