DOI: https://doi.org/10.56669/CIZI6125
ABSTRACT
Finding a solution to combat plant disease and control pests in organic farming remains a challenge. Biological control is becoming an indispensable tool in crop protection and maintaining plant health care. Biocontrol agents such as Bacillus species have multiple functions that can suppress plant pathogens, promote plant growth, and induce plant resistance to abiotic factors in the agroecosystem. They have been developed as biopesticides, biofertilizers, and biostimulants to date. In Taiwan, our transdisciplinary team aimed to devise a solution to eliminate the bottleneck effect during the mass production of biocontrol agents and expand their applications. We discovered three probiotics as biocontrol agents (Bacillus mycoides BM02, Bacillus licheniformis EC34-01, and Bacillus subtilis 151B1), established a standard protocol of their fermentation and formulation technology using the pilot plant and industrial-scale facilities, and determined their application potential in crop protection, livestock farming, aquaculture, and agricultural pollutants. Among these probiotics, B. mycoides BM02 products are commercialized and licensed as biopesticides and biofertilizers. The influence of introducing these Bacillus-based probiotics on the microbiome of the plant rhizosphere and fish guts will also be discussed in this paper.
Keywords: Bacillus licheniformis, Bacillus mycoides, Bacillus subtilis, aquafeeds, biopesticides, biofertilizers, feed additives, next-generation sequencing, probiotics, microbiome
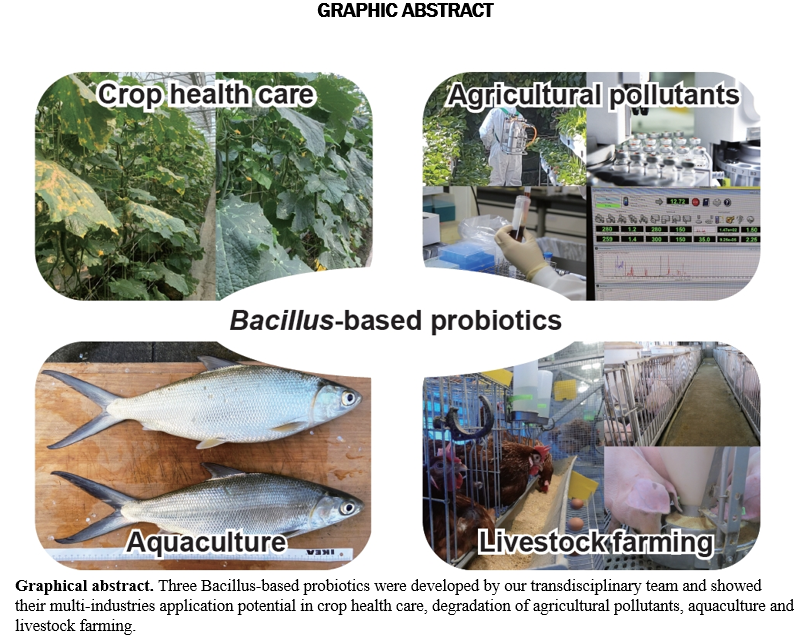
INTRODUCTION
With the growing demand for organic cultivation, food safety, and a sustainable environment, it has become necessary to develop biological control agents as alternatives to chemical pesticides in organic farming and as a part of integrated pest management strategies. The research on biological control and its application using introduced probiotics has been investigated and developed for more than half a century (Cook, 1990); however, progress in the commercialization of biocontrol products has been slow, implicating a gap between research and product development (Huang, 2003). Baker and Stavely (1985) first reported the application of a probiotic bacterium (Bacillus subtilis) in the field of agriculture to control bean rust. Their findings indicated that the bean rust severity was reduced by at least 75% following once per week for three applications of B. subtilis isolates PPL-3 and APPL-1 (Baker and Stavely, 1985). Additionally, isolate PPL-3 had a stimulatory effect on plant growth (Baker and Stavely, 1985). However, the first commercial B. subtilis FZB24® product (ABiTEP, GmbH, Berlin) for seed potato coating was unavailable until 1999. Another phyllosphere-inhabiting Bacillus specie, Bacillus mycoides isolate Bac J, was found to induce systemic resistance in sugar beet, and reduce Cercospora leaf spot (Bargabus et al., 2002). The B. mycoides isolate Bac J product was commercialized by Certis Biologicals (Columbia, MD, USA) as a biopesticide, and was granted registration till the year of 2016 by the U.S Environmental Protection Agency and Health Canada's Pest Management Regulatory Agency. With the market driven factors such as the technical advance in mass production, the increasing efficacy in biocontrol products, lower raw material costs, fast regulatory approvals, and favorable government initiatives to promote the adoption of biocontrol agents, the microbial agent market for agriculture is projected to grow (Huang, 2003). According to the 2021 Research and Markets report, the global biopesticide market reached a value of USD 3.96 billion in 2020 and is expected to exhibit strong growth from 2021 to 2026.
Probiotic microorganisms have multiple functions. Their significant beneficial effects on plants include protecting plants from pathogen infections, promoting the absorption and utilization of plant nutrients, and enhancing plant vigor (Chojnacka, 2015). Thus, they could be commercialized as biopesticides, biofertilizers, and biostimulants (Chojnacka, 2015). Probiotics such as well-known lactic acid bacteria also exhibit the potential to improve animal health, efficiency, and meat quality (Al-Shawi et al., 2020). In Taiwan, our transdisciplinary team with expertise in plant pathology, pesticide analysis, aquatic management, livestock production, and microbial fermentation aimed to develop a solution to eliminate the bottleneck effect during the mass production of biocontrol products and to expand the applications of probiotic products. We jointly discovered three probiotics (Bacillus mycoides BM02, Bacillus licheniformis EC34-01, and Bacillus subtilis 151B1), established a standard protocol of the fermentation and formulation technology using the pilot plant and industrial-scale facilities, and demonstrated their high potential for application in crop protection, circular agriculture, livestock farming, and aquaculture. Among the three probiotics, B. mycoides BM02-based products registered as biopesticides or biofertilizers are currently commercialized by several enterprises through technology transfer from the Intellectual Property Center of the National Chung-Hsing University. The mass production and application technology of B. subtilis 151B1 was also transferred to two enterprises for commercialization. The efficacy of these three probiotics and their potential mechanisms in plant protection is also discussed in this paper.
The biological control of plant diseases with introduced antagonists is a common strategy. However, the effect of modifying the balance of the flora and microbial ecology by augmenting a specific population can only be temporary (Garrett, 1965). (Huang, 2003) suggested that the ecological effect of biocontrol organisms on the environment should be considered to improve the efficacy of introduced biocontrol agents. Technical advances in next-generation sequencing have allowed us to understand the compositions of microbial communities associated with plants or animals. The effect of introducing Bacillus-based probiotics on the microbiome of the plant rhizosphere, hog feces, and fish guts is also discussed in the latter part of the paper.
Combating disease and pests without suitable and enough resources is always a challenge in organic cultivation. Here, we describe our experience in developing biological control agents through the stages of laboratory research to commercialization, from basic mechanism studies to field applications in Taiwan. B. mycoides BM02 products are licensed as biopesticides and biofertilizers and effectively control diseases and promote the growth of several crops. Additionally, our transdisciplinary team—led by Professor Jenn-Wen Huang—collaborated to explore and develop B. subtilis 151B1 and B. licheniformis agents EC34-01 (originally designed for plant protection) for multi-industry applications in sectors including crop health care, livestock farming, aquaculture, and agricultural pollutants.
BACILLUS-BASED PROBIOTIC PRODUCTS, APPLICATIONS, AND MECHANISMS
Bacillus-based probiotic products
B. mycoides is commonly found in soils and the plant rhizosphere or phyllosphere, exhibits rhizoid colony morphology on nutrient agar, and forms stress-resistant endospores (Buyer, 1995; Di Franco et al., 2002). B. mycoides BM02 isolated from a tomato rhizosphere in central Taiwan was selected from more than 1,000 isolated strains for their effects on crop growth promotion and disease control (Huang et al., 2018). Strain BM02 enhanced the growth of various plants, including tomato, lettuce, edible rape, cabbage, and asparagus bean, and effectively controlled tomato Fusarium wilt, cucumber damping-off, powdery mildew of tomato and cucumber, strawberry gray mold, cabbage clubroot, rice blast, scallion rust, and strawberry mites (Table 1; (Huang et al., 2018). The research results related to B. mycoides were granted two invention patents (Taiwan Patent Numbers I510619 and I486444) non-exclusively licensed to six enterprises. B. mycoides BM02 products with different formulations and processes are commercialized and licensed as biofertilizers (e.g., “Shen Zen Shui No. 2” and “Syun Meng Nong”, and biopesticides (e.g., “Jhih Huang Ye”) for the control of orchid Fusarium yellows in Taiwan.
B. subtilis and B. licheniformis are also endospore-forming bacteria. They are phylogenetically and phenotypically related and are, therefore, taxonomically arranged in the same “B. subtilis group” (Fritze, 2004). Both Bacillus species are certified as “Generally Recognized As Safe (GRAS)” bacteria by the US Food and Drug Administration. Moreover, they feature in the “Qualified Presumption of Safety (QPS)” list by the European Food Safety Authority and in the feed additives list by the Council of Agriculture, Executive Yuan in Taiwan, suggesting that they are safe for agriculture and human use (Sorokulova et al., 2008; Olmos and Paniagua-Michel, 2014). Here, we obtained B. subtilis 151B1 and B. licheniformis EC-34-01 from the rhizosphere of tea plants and soil-planted water chestnut in Taiwan, respectively (Chen et al., 2021; Chiang, 2011). Both strains possessed antagonistic activities against various plant pathogens, protected crops from pathogen infections, and promoted plant growth (Tables 1 and 2; Figures 2 and 3). The microbial agents of B. subtilis 151B1 and B. licheniformis EC34-01 were developed in liquid and powder formulations and spray-coating granules using a pilot plant and industrial-scale facilities to assess their efficacy in plant protection, pesticide degradation, livestock production, and aquaculture applications.
Plant disease suppression and growth promotion by three probiotics
Applications of B. mycoides BM02 products in the fields displayed a reduction in the disease severity (ranging from 18% to 66%) of various plant diseases, including cabbage damping-off, cucumber Pythium damping-off, tomato Fusarium wilt, rice sheath blight, rice blast, rice bacterial leaf blight, orchid Fusarium yellows, eggplant bacterial wilt, strawberry Fusarium wilt, and jewel orchid stem rot, compared to non-treated fields (Table 1; Figures 1A, B, and C). B. mycoides BM02 products not only suppressed plant diseases but also promoted the growth of strawberry and rice plants, as well as enhanced the yield of rice and strawberry fruits by up to 67% (Table 2; Figures 2A, C, and E).
B. subtilis 151B1 in liquid formulations was effective in suppressing passion fruit Fusarium disease, passion fruit anthracnose, tea brown blight, cucumber damping-off, cucumber Fusarium wilt, cucumber downy mildew, and tomato bacterial wilt (Table 1; Figure 1D). Application of B. subtilis 151B1 in the field promoted the growth of cucumber plants (a twofold increase in height was observed in treated plants, compared with untreated controls) and increased the yields of passion fruit, tea, and cucumber (Table 2; Figures 2B and D). Compared with untreated plants, the average yield of cucumber per day increased to 29.6% in plants treated with B. subtilis 151B1 liquid formulations (Table 2; Figure 2D). The cucumber yield further increased to 40.3% when a combination of B. subtilis 151B1 and CH100 plant extracts was used (Figure 2 D). CH100 is a commercial plant nutrient developed using agricultural waste containing extracts of tobacco debris and cabbage leaves, and SH soil amendment (Huang, 1992 ; Sun and Huang, 1985).
The application of B. licheniformis EC34-01 in liquid formulations can control mango anthracnose, cucumber damping-off, and cucumber Fusarium wilt (Table 1). Additionally, the application of B. licheniformis EC34-01 once per week for five weeks to 20-year-old tea plants in the field significantly enhanced tea yield to 277%, compared with untreated plants (Table 2).
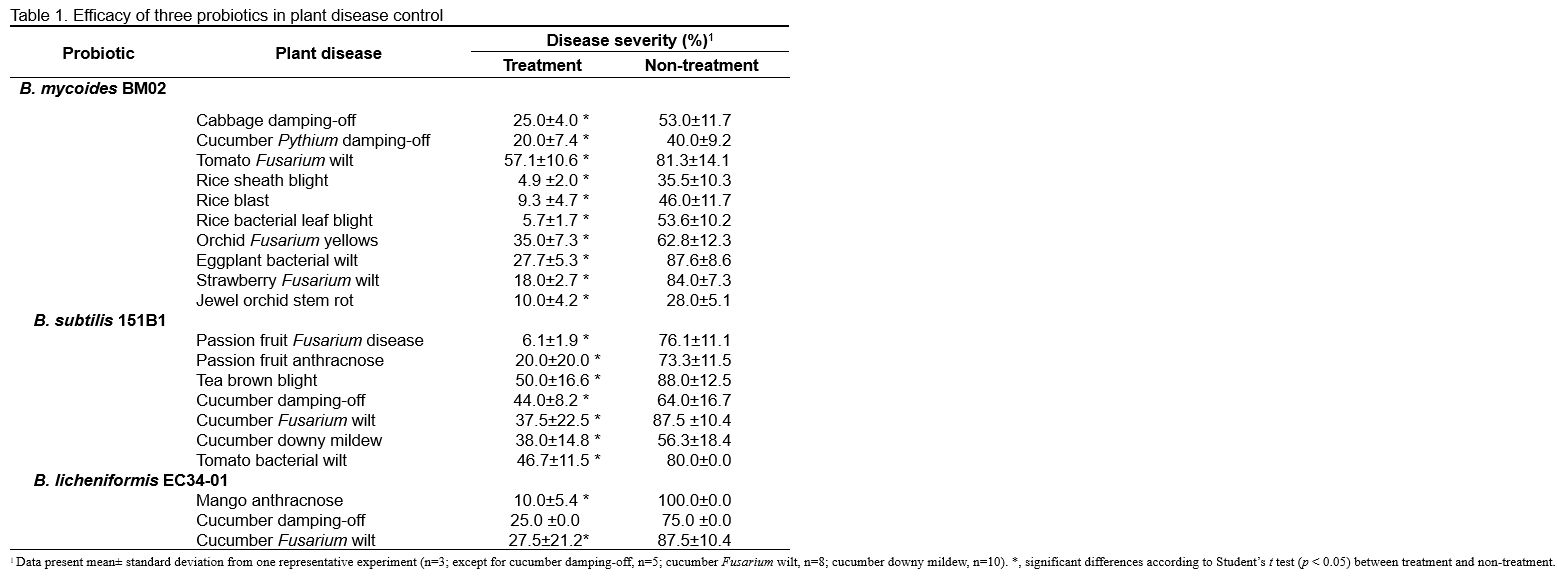
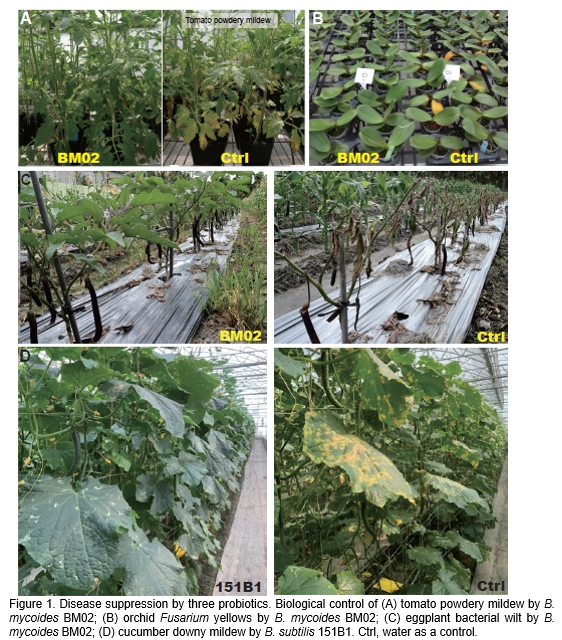
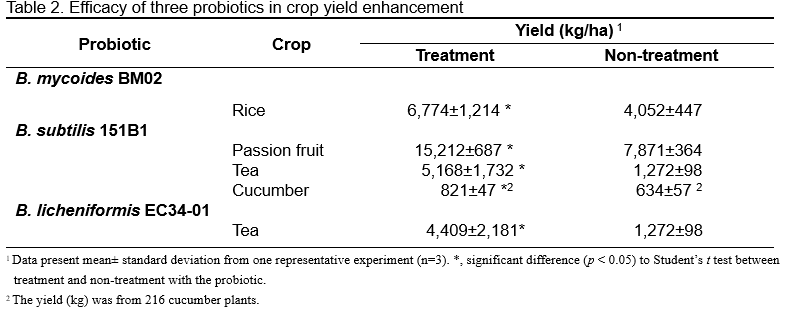
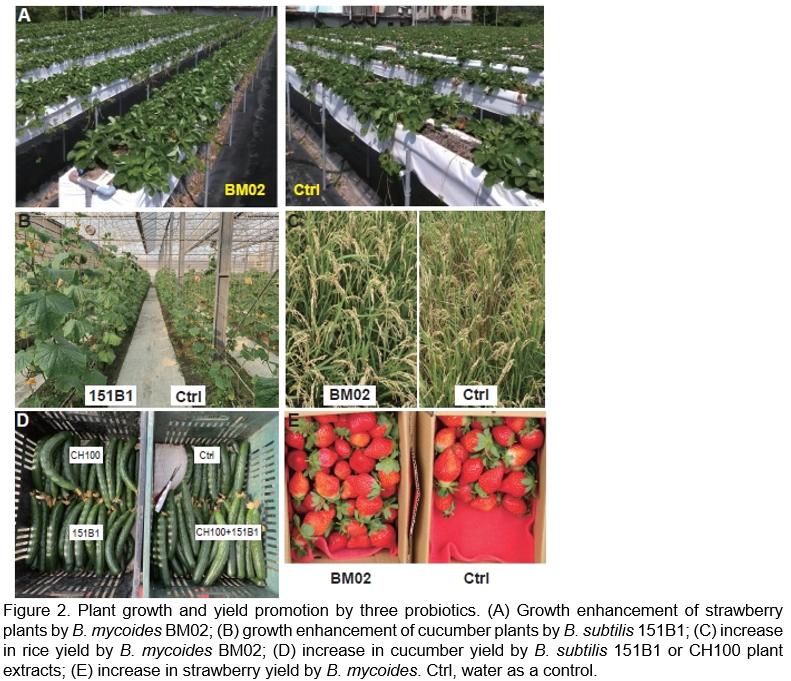
Mechanisms of disease control and plant growth promotion
The efficacy of B. mycoides BM02, B. subtilis 151B1, and B. licheniformis EC-34-01 applications on plant disease control and plant growth promotion have been demonstrated under laboratory, greenhouse, and field studies. The mechanisms of B. mycoides BM02 in plant health protection are: (i) B. mycoides BM02 could colonize plant roots, proliferate in plant tissues, and induce systemic resistance in plants (Figure 3); (ii) B. mycoides BM02 could secrete plant growth hormones, e.g., indole-acetic acid (IAA) to promote root growth; (iii) B. mycoides BM02 could produce dimethyl-disulfide, phenylacetic acid, methylphenyl acetate, and surfactin, which can effectively disrupt the pathogens' cell membranes and inhibit the infection of plant pathogens (Huang et al., 2018; Wu et al., 2020). Our research indicated that B. subtilis 151B1 possessed plant growth-promoting traits (e.g., displaying activities in production of protease, amylase, cellulase, lipase, and IAA, as well as phosphorus solubilizing), thus suggesting its potential as a biofertilizer (Huang et al., unpublished data). B. subtilis 151B1 also could produce C14 and C15 family surfactins, as well as C14 and C15 family iturins (Chen et al., 2021). It has been suggested that the surfactins produced by B. subtilis 151B1 may trigger apoptotic-like cell death, reducing mitochondrial membrane potential and interfering with the energy metabolism of the plant pathogens (Chen et al., 2021). Additionally, B. subtilis 151B1 was shown to enhance the expression of plant defense genes, including the phenylalanine ammonia-lyase (PAL), peroxidase (POX), and pathogenesis-related (PR1a) genes, in cucumber seedlings after 24 h of inoculation, compared with control (water-treated) seedlings (Huang et al., unpublished data). Thus, B. subtilis 151B1 exhibits multiple mechanisms to control various plant diseases caused by pathogens and is suitable as a biopesticide. Acting as a biocontrol agent for plant diseases, B. licheniformis EC-34-01 was found to inhibit the mycelial growth of various fungal pathogens, including Colletotrichum gloeosporioides, Colletotrichum higginsianum, Colletotrichum orbiculare, and Alternaria brassicicola (Chiang, 2011), which may be attributed to the induction of the plant’s defense response. In contrast to B. subtilis 151B1, B. licheniformis EC-34-01 induces the expression of PAL, POX, and PR1a only after the cucumber seedlings are challenged with pathogens (Huang et al., unpublished data).
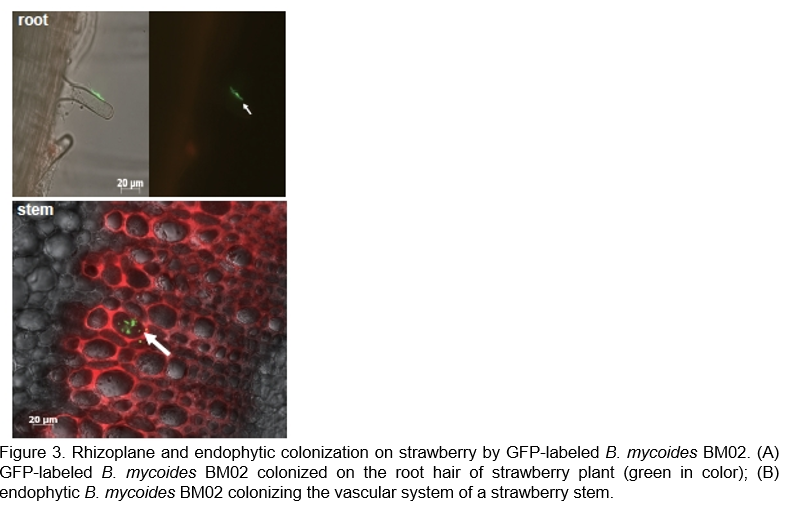
Applications of Bacillus-based probiotic products in the livestock farming and aquaculture
Due to concerns about antimicrobial resistance to global public health threats and the imbalance of ecosystem, as well as to achieve the Sustainable Development Goals, a reduction in the use of antibiotics to promote growth or prevent diseases in healthy animals was suggested by the World Health Organization (2020) and World Organization for Animal Health (2015). Bacillus-based probiotics are also used as potential alternatives to antibiotics in animal agriculture to improve animal health, growth efficiency, and meat quality (Al-Shawi et al., 2020; Verschuere et al., 2000). In our studies, microbial agents of B. subtilis 151B1 and B. licheniformis EC34-01 in powder formulations and spray-coating granules were assessed for their efficacy as feed additives in swine raising, as well as milkfish and shrimp farming (Huang, 2021). Our findings indicated that using B. subtilis 151B1 as a feed additive in the hogs’ diet increased their daily food intake by 6.1% and daily weight gain by 3.4%, as well as shortened the feeding time by 3 days, compared to control animals. It also improved the weight of pork tenderloin and the texture of short fillet (Figure 4A; (Huang, 2021); Lin et al., unpublished data). Additionally, B. subtilis 151B1 as a feed additive decreased the H2S and thiol contents in the hog feces by 25% and 81%, respectively, (i.e., 17.75 ppm and 0.15 ppm, respectively), compared with untreated control (23.50 ppm H2S and 0.79 ppm thiol contents), suggesting its potential for improving the farm environment (Lin et al., unpublished data). Our results also showed that applications of B. subtilis 151B1 and B. licheniformis EC34-01 as feed additives improved the feed conversion ratio and enhanced the growth of milkfish, with the treatment with strain EC34-01 being the most effective (Figure 4B; (Huang, 2021); Lu et al., unpublished data). Moreover, they reduced the populations of pathogenic Vibrio and improved the water quality of fish and shrimp breeding farms by reducing the ammonia, nitrate-nitrogen, and nitrite-nitrogen contents (Lu et al., unpublished data). These results suggested that both strains had excellent potential to be used as feed additives to promote the productive performance of livestock and aquatic animals.
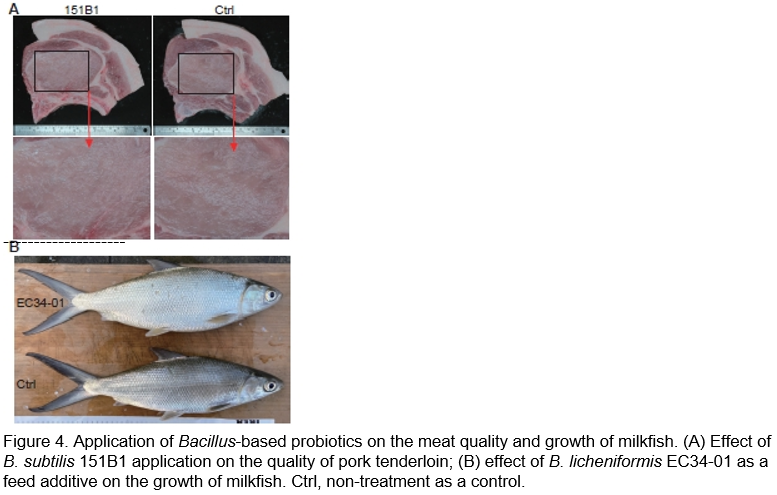
Together with that of other researchers, our work demonstrated the use of B. subtilis and B. licheniformis exert numerous beneficial effects on animals (Al-Shawi et al., 2020; Verschuere et al., 2000). These two probiotics are good alternatives for improving feed utilization, leading to enhanced growth performance, stress response, immune response, disease resistance, and meat and water quality for sustainable livestock farming and aquaculture (Al-Shawi et al., 2020; Kuebutornye et al., 2019). The proposed mechanisms of these two probiotics may be attributed to enhance the digestive enzyme activity, antioxidant enzyme activity, and expression of immune-related as well as stress-related genes, produce antimicrobial compounds (organic acids, hydrogen peroxidase, bacteriocins, and biosurfactants), and modulate the gut microbiota (Kuebutornye et al., 2019; Ahmadnia Motlagh et al., 2012). For example, B. subtilis and B. licheniformis improved the growth of brine shrimp (Artemia urmiana) by increasing digestive enzyme activities such as protease and amylase activities and enhancing the Bacillus population in the gut (Ahmadnia Motlagh et al., 2012). Tilapia fed with mixed products of B. subtilis and B. licheniformis showed improved growth and immune response, and were resistant to Streptococcus agalactiae infections (Abarike et al., 2018). Our results showed B. subtilis 151B1 and B. licheniformis EC34-01 could modulate the microbiota in chicken litters and the intestine of milkfish (discussed in a later section). B. subtilis 151B1 can produce protease, cellulase, lipase, amylase, and biosurfactants (C14 and C15 family surfactins, as well as C14 and C15 family iturin A) (Chen et al., 2021). Additionally, we also detected enhanced IL-2 and IFN-γ levels in the serum of piglets (Lin et al., unpublished data). Our unpublished data indicated that B. licheniformis EC34-01 produced genistein. Genistein is a compound commonly found in soybean-based fermentation broths containing B. licheniformis and is known to function as an antioxidant and anthelmintic (Yang et al., 2013). Whether genistein also plays a role in improving the growth performance, stress response, immune response, and disease resistance of milkfish treated with B. licheniformis EC34-01 remains to be determined.
Degradation of agricultural pollutants
Agricultural pollutants may come from wastes generated from plant cultivation, livestock raising, and fisheries. For instance, chemical pesticides are often found in agricultural wastes, which, if left untreated before being used as compost, may raise safety concerns about its recycling. The manure obtained from livestock raising is also a concern for the farming environment. Furthermore, the nitrogen contents greatly impact the water quality of aquaculture farms. Microorganisms could play important roles in the bioremediation of pollutants (Sarker et al., 2021; Hlordzi et al., 2020). Here, we found that B. subtilis 151B1 and B. licheniformis E34-01 could degrade chemical insecticides (e.g., marathon and deltamethrin) and a fungicide (e.g., tricyclazole) (Figure 5)—a property that could solve the problem of potential pesticide residue when recycling agricultural by-products. The H2S and thiol contents in the feces of hogs fed with the B. subtilis 151B1 additive were decreased (17.75 and 0.15 ppm, respectively) compared to the feces of control animals (23.50 and 0.79 ppm, respectively). The concentrations of ammonium-nitrogen, nitrite-nitrogen, and nitrate-nitrogen in the water of milkfish raising farms were reduced following treatment with B. subtilis 151B1 and B. licheniformis E34-01, compared to the control (untreated) farm water (data not shown). These data suggested that adding B. subtilis 151B1 and B. licheniformis E34-01 to the livestock or fish feeds may improve the farm environment and water quality.
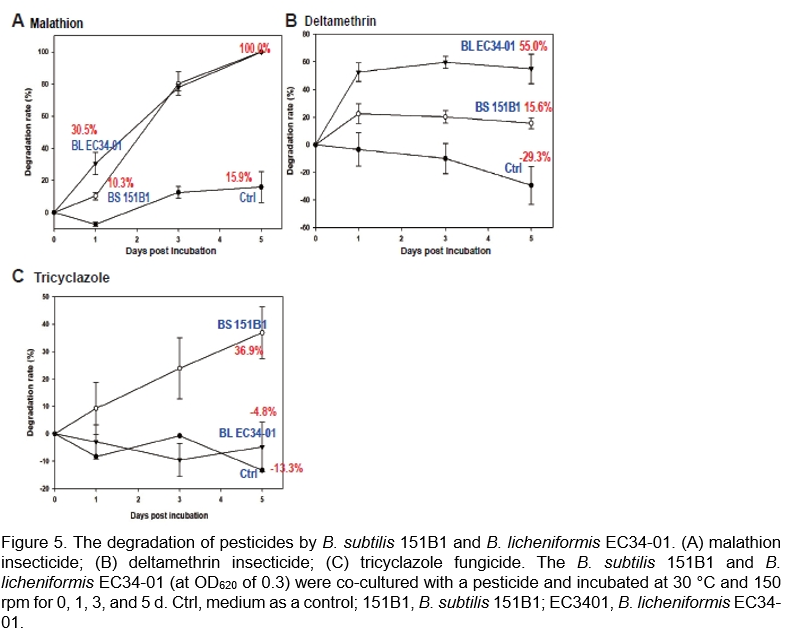
Modulation of microbiomes by the probiotics
Probiotics can improve the intestinal microbial balance, consequently improving feed utilization, promoting growth, strengthening the immune response, and suppressing pathogen infections in humans and animals (Fuller, 1989; Saarela et al., 2000). Understanding the interactions between microorganisms and hosts could offer insights into the beneficial applications of utilizing and manipulating microbes (Mahmud et al., 2021; Trivedi et al., 2020; Trivedi et al., 2021). The rhizosphere microbiome is referred to as the second or extended genome for maintaining plant health (Berendsen et al., 2012). Using next-generation sequencing analysis, we found shifts in the bacterial communities of the passion fruit rhizosphere following the introduction of the B. subtilis strain 151B1. Comparing the enriched bacterial genera identified in the rhizosphere applying strain 151B1 with the control environment, we detected Streptomyces spp. (10.3% vs. 6.6%), Bacillus spp. (7.1% vs. 3.2%), Pyrinomonadaceae RB41 (5.4% vs. 2.8%), Paraburkholderia spp. (6.4% vs. 2.0%), Nocardioides spp. (4.8% vs. 2.8%), Nitrospira spp. (4.2% vs. 1.9%), Bradyrhizobium spp. (3.8% vs. 1.6%), and Nitrosomonadaceae MND1 (4.1% vs. 1.3%); by contrast, the reduced bacterial genera included Chujaibacter spp. (12.1% vs. 20.3%), Rhodanobacter spp. (3.8% vs. 7.6%), Alcanivorax spp. (0.5% vs. 8.8%), Candidimonas spp. (2.6% vs. 4.2%), and Arachidicoccus spp. (1.4% vs. 4.4%) (Figure 6A). Among the enriched bacterial genera, most of the known Streptomyces spp., Bacillus spp., Paraburkholderia spp., and Bradyrhizobium spp. harbored plant growth-promoting features (Ormeño-Orrillo and Martínez-Romero, 2019; Berg, 2009). The Nitrosomonadaceae family comprises Nitrosomonas and Nitrosospira spp.; the latter are ammonia oxidizers, i.e., they oxidize ammonia to nitrite, which is subsequently oxidized by bacterial nitrite oxidizers to nitrate (Daims et al., 2015). Nocardioides strains play an important role in bioremediation (Yoon and Park, 2006).
Similarly, a decrease in the pathogenic Fusobacteriales and Vibrionales populations and an increase in the beneficial Lactobacillales in the gut microbiota of milkfish treated with B. subtilis 151B1 and B. licheniformis EC34-01 strains were found, suggesting the potential of these strains in reducing the incidence of fish diseases (Figure 6B). Additionally, B. subtilis and B. licheniformis as feed additives improve the water quality of aquaculture farms and modulate the diversity of macroalgae (the source of fish food) in the farming water (data not shown).
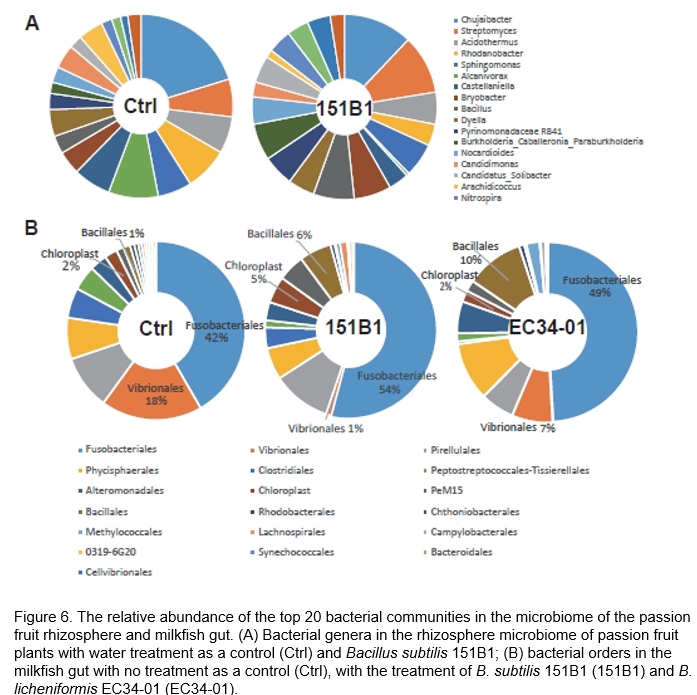
FUTURE PERSPECTIVES
The concept of biological control originated from managing soil suppressiveness using natural microorganisms. Biological control using introduced antagonists has become a common strategy nowadays (Cook, 1990). However, there are technical, regulatory, and marketing hurdles to overcome for the commercialization, application, and global sale of microbial biocontrol agents. From our experience developing Bacillus-based products, teamwork with transdisciplinary experts from academic and private sectors could accelerate the research, development, and commercialization of biocontrol products. Using a pilot plant facility to mass-produce the biocontrol agents and having the efficacy of product prototypes evaluated by experts in their fields of study would not only minimize the gap between laboratory research and commercialization but also validate their efficacy and provide the scientific-based technical dossiers for product registration and application. Furthermore, because the regulatory policy and dossier requirements to register biocontrol agents are different in each country, the involvement of government departments to harmonize the regulations and quarantine measures would facilitate the global market sale of the biocontrol products.
Next-generation sequencing technologies have allowed researchers to access and explore the diversity and complexity of the rhizosphere, environmental, and gut microbiomes. By comparing bacterial communities with and without treatment of probiotics in the rhizospheres of passion fruit, chicken litter, and milkfish gut, we found that the introduction of B. subtilis 151B1 and B. licheniformis EC34-01 did not affect bacterial diversity but modulated the composition of bacterial microbiomes by enhancing the beneficial population and reducing the potentially pathogenic population. These findings further suggested that the introduction of probiotics in crop fields could maintain the balance of the microbial ecosystem, similar to our findings, as well as those of other studies, in the animal system. Thus, probiotics are excellent candidates as alternatives to chemical pesticides, fertilizers, and feed additives for sustainable agriculture.
Probiotic bacteria could serve as an alternative solution to control diseases and pests, particularly in, but not limited to, organic farming. A selection of compatible chemical pesticides containing probiotics that target specific modes of action would expand the use of probiotic products in conventional farming practices. The use of probiotics in integrated pest management systems could serve as a resistance management tool and ensure the safety of agricultural products, especially those with short pre-harvest and re-entry intervals, and without the issue of maximum residue limits. Additionally, based on our data, probiotic bacteria could further serve as a pesticide degrader, which is important to reduce pesticide residues in agricultural products and by-products. Because probiotics are live organisms, choosing the right kind at the right time to use in the integrated pest management system is a critical process to ensure their highest efficacy. Input of professional knowledge from Plant Doctor, Pest Control Advisor, or Plant Protection practitioner to work with the growers would advance the application practice of probiotics and in turns maximizing the efficacy.
ACKNOWLEDGMENTS
The research was supported by and the Ministry of Science and Technology, Taiwan (MOST 110-2321-B-005-006), and the “Innovation and Development Center of Sustainable Agriculture” from the Featured Area Research Center Program within the framework of the Higher Education Sprout Project by the Ministry of Education in Taiwan. The authors thank Miss Yu-Hsuan Chen in Dept. Plant Pathology, National Chung Hsing University for designing graphical abstract.
CONFLICT OF INTEREST
The authors declare no conflict of interest.
ORCID
Tzu-Pi Huang https://orcid.org/0000-0003-2904-3373
Jenn-Wen Huang https://orcid.org/0000-0003-0798-4332
Chuan-Shun Lin https://orcid.org/0000-0002-9879-6101
Wen-Hsin Chung https://orcid.org/0000-0002-8517-6368
REFERENCCES
Abarike E. D., Cai J., Lu Y., Yu H., Chen L., Jian J., Tang J., Jun L., Kuebutornye F. K. A. (2018). Effects of a commercial probiotic BS containing Bacillus subtilis and Bacillus licheniformis on growth, immune response and disease resistance in Nile tilapia, Oreochromis niloticus. Fish & Shellfish Immunology, 82, 229-238.
Ahmadnia Motlagh H. R., Farhangi M., Rafiee G., Noori F. (2012). Modulating gut microbiota and digestive enzyme activities of Artemia urmiana by administration of different levels of Bacillus subtilis and Bacillus licheniformis. Aquacult. International, 20, 693-705.
Al-Shawi S. G., Dang D. S., Yousif A. Y., Al-Younis Z. K., Najm T. A., Matarneh S. K. (2020). The potential use of probiotics to improve animal health, efficiency, and meat quality: a review. Agriculture, 10, 452.
Baker C. J., Stavely J. R. (1985). Biocontrol of bean rust by Bacillus under field conditions. Plant Disease, 69, 770-772.
Bargabus R. L., Zidack N. K., Sherwood J. E., Jacobsen B. J. (2002). Characterisation of systemic resistance in sugar beet elicited by a non-pathogenic, phyllosphere-colonizing Bacillus mycoides, biological control agent. Physiological and Molecular Plant Pathology, 61, 289-298.
Berendsen R. L., Pieterse C. M. J., Bakker P. A. H. M. (2012). The rhizosphere microbiome and plant health. Trends in Plant Science, 17, 478-486.
Berg G. (2009). Plant–microbe interactions promoting plant growth and health: perspectives for controlled use of microorganisms in agriculture. Applied Microbiology and Biotechnology, 84, 11-18.
Buyer J. S. (1995). A soil and rhizosphere microorganism isolation and enumeration medium that inhibits Bacillus mycoides. Applied and Environmental Microbiology, 61, 1839-1842.
Chen Y.-H., Lee P.-C., Huang T.-P. (2021). Biological control of collar rot on passion fruits via induction of apoptosis in the collar rot pathogen by Bacillus subtilis. Phytopathology, 111, 627-638.
Chiang C.-J. (2011) Identification for biocontrol agents of mango anthracnose and design of their pilot-scale fermenting nutrient recipes for the disease control Ms. Sci. Master thesis, National Chung Hsing University.
Chojnacka K. (2015). Innovative bio-products for agriculture. Open Chemistry, 13, 932-937.
Cook R. J. (1990). Twenty-five years of progress towards biological control. In: Biological Control of Soil-Borne Plant Pathogens, pp. 1-14 Ed D. Hornby. Wallingford, UK.: CAB International
Daims H., Lebedeva E. V., Pjevac P., Han P., Herbold C., Albertsen M., Jehmlich N., Palatinszky M., Vierheilig J., Bulaev A., Kirkegaard R. H., von Bergen M., Rattei T., Bendinger B., Nielsen P. H., Wagner M. (2015). Complete nitrification by Nitrospira bacteria. Nature, 528, 504-509.
Di Franco C., Beccari E., Santini T., Pisaneschi G., Tecce G. (2002). Colony shape as a genetic trait in the pattern-forming Bacillus mycoides. BMC Microbiology, 2, 33.
Fritze D. (2004). Taxonomy of the genus Bacillus and related genera: the aerobic endospore-forming bacteria. Phytopathology, 94, 1245-1248.
Fuller R. (1989). Probiotics in man and animals. J. Appl. Bacteriol., 66, 365-378.
Garrett S. D. (1965). Toward biological control of soil-borne plant pathogens. In: Ecology of Soil-Borne Plant Pathogens Prelude to Biological Control, pp. 423-435 Eds K. F. Baker & W. C. Snyder. Berkeley, California, USA: University of California Press.
Hlordzi V., Kuebutornye F. K. A., Afriyie G., Abarike E. D., Lu Y., Chi S., Anokyewaa M. A. (2020). The use of Bacillus species in maintenance of water quality in aquaculture: A review. Aquaculture Reports, 18, 100503.
Huang H.-C. (2003). Biocontrol of plant disease: research and application. In: Advances in Plant Disease Management 2003, pp. 1-18 Eds H.-C. Huang & S. N. Acharya. Trivandrum, India: Research Signpost.
Huang J.-S., Peng Y.-H., Chung K.-R., Huang J.-W. (2018). Suppressive efficacy of volatile compounds produced by Bacillus mycoides on damping-off pathogens of cabbage seedlings. The Journal of Agricultural Science, 156, 795-809.
Huang J. W. (1992 ). Integrated management of vegetable seedling pests with a formulated plant nutrition. Plant Protection Bulletin, 34, 54-63.
Huang T.-P. (2021) Probiotic microbiomes for agriculture safety and green gold creation. In: MOST Center for Golobal Affairs and Science Engagement (GASE) Newsletter - Taiwan Research Highlight, p. https://trh.gase.most.ntnu.edu.tw/en/article/content/207. Taipei, Taiwan: Ministry of Science and Technology.
Kuebutornye F. K. A., Abarike E. D., Lu Y. (2019). A review on the application of Bacillus as probiotics in aquaculture. Fish & Shellfish Immunology, 87, 820-828.
Mahmud K., Missaoui A., Lee K., Ghimire B., Presley H. W., Makaju S. (2021). Rhizosphere microbiome manipulation for sustainable crop production. Current Plant Biology, 27, 100210.
Olmos J., Paniagua-Michel J. (2014). Bacillus subtilis a potential probiotic bacterium to formulate functional feeds for aquaculture. Journal of Microbial & Biochemical Technology, 6, 361-365.
Ormeño-Orrillo E., Martínez-Romero E. (2019). A genomotaxonomy view of the Bradyrhizobium genus. Frontiers in Microbiology, 10.
Saarela M., Mogensen G., Fondén R., Mättö J., Mattila-Sandholm T. (2000). Probiotic bacteria: safety, functional and technological properties. Journal of Biotechnology, 84, 197-215.
Sarker A., Nandi R., Kim J.-E., Islam T. (2021). Remediation of chemical pesticides from contaminated sites through potential microorganisms and their functional enzymes: Prospects and challenges. Environmental Technology & Innovation, 23, 101777.
Sorokulova I. B., Pinchuk I. V., Denayrolles M., Osipova I. G., Huang J. M., Cutting S. M., Urdaci M. C. (2008). The safety of two Bacillus probiotic strains for human use. Diggestive Diseases and Sciences, 53, 954-963.
Sun S. K., Huang J. W. (1985). Formulated soil amendment for controlling Fusarium wilt and other soilbrone diseases=. Plant Disease, 69, 917-920.
Trivedi P., Leach J. E., Tringe S. G., Sa T., Singh B. K. (2020). Plant–microbiome interactions: from community assembly to plant health. Nature Review of Microbiology, 18, 607-621.
Trivedi P., Mattupalli C., Eversole K., Leach J. E. (2021). Enabling sustainable agriculture through understanding and enhancement of microbiomes. New Phytologist, 230, 2129-2147.
Verschuere L., Rombaut G., Sorgeloos P., Verstraete W. (2000). Probiotic bacteria as biological control agents in aquaculture. Microbiology and Molecular Biology Reviews, 64, 655-671.
World Health Organization (2020) World Health Organization antimicrobial resistance fact sheet. In.
World Organization for Animal Health (2015) Antimicrobial resistance fact sheets [Online]. Available: https://www.oie.int/fileadmin/Home/eng/Media_Center/docs/pdf/Fact_sheets/ANTIBIO_EN.pdf [Accessed].
Wu J.-J., Huang J.-W., Deng W.-L. (2020). Phenylacetic acid and methylphenyl acetate from the biocontrol bacterium Bacillus mycoides BM02 suppress spore germination in Fusarium oxysporum f. sp. lycopersici. Frontiers in Microbiology, 11, 569263 doi: 569210.563389/fmicb.562020.569263.
Yang H. J., Kwon D. Y., Moond N. R., Kimb M. J., Kang H. J., Jungc D. Y., Park S. (2013). Soybean fermentation with Bacillus licheniformis increases insulin sensitizing and insulinotropic activity. Food & Function, 4, 1675-1684.
Yoon J.-H., Park Y.-H. (2006). The Genus Nocardioides. Prokaryotes, 3, 1099-1113.
Multiple Functions of Bacillus Biocontrol Agents for Agricultural Production
DOI: https://doi.org/10.56669/CIZI6125
ABSTRACT
Finding a solution to combat plant disease and control pests in organic farming remains a challenge. Biological control is becoming an indispensable tool in crop protection and maintaining plant health care. Biocontrol agents such as Bacillus species have multiple functions that can suppress plant pathogens, promote plant growth, and induce plant resistance to abiotic factors in the agroecosystem. They have been developed as biopesticides, biofertilizers, and biostimulants to date. In Taiwan, our transdisciplinary team aimed to devise a solution to eliminate the bottleneck effect during the mass production of biocontrol agents and expand their applications. We discovered three probiotics as biocontrol agents (Bacillus mycoides BM02, Bacillus licheniformis EC34-01, and Bacillus subtilis 151B1), established a standard protocol of their fermentation and formulation technology using the pilot plant and industrial-scale facilities, and determined their application potential in crop protection, livestock farming, aquaculture, and agricultural pollutants. Among these probiotics, B. mycoides BM02 products are commercialized and licensed as biopesticides and biofertilizers. The influence of introducing these Bacillus-based probiotics on the microbiome of the plant rhizosphere and fish guts will also be discussed in this paper.
Keywords: Bacillus licheniformis, Bacillus mycoides, Bacillus subtilis, aquafeeds, biopesticides, biofertilizers, feed additives, next-generation sequencing, probiotics, microbiome
INTRODUCTION
With the growing demand for organic cultivation, food safety, and a sustainable environment, it has become necessary to develop biological control agents as alternatives to chemical pesticides in organic farming and as a part of integrated pest management strategies. The research on biological control and its application using introduced probiotics has been investigated and developed for more than half a century (Cook, 1990); however, progress in the commercialization of biocontrol products has been slow, implicating a gap between research and product development (Huang, 2003). Baker and Stavely (1985) first reported the application of a probiotic bacterium (Bacillus subtilis) in the field of agriculture to control bean rust. Their findings indicated that the bean rust severity was reduced by at least 75% following once per week for three applications of B. subtilis isolates PPL-3 and APPL-1 (Baker and Stavely, 1985). Additionally, isolate PPL-3 had a stimulatory effect on plant growth (Baker and Stavely, 1985). However, the first commercial B. subtilis FZB24® product (ABiTEP, GmbH, Berlin) for seed potato coating was unavailable until 1999. Another phyllosphere-inhabiting Bacillus specie, Bacillus mycoides isolate Bac J, was found to induce systemic resistance in sugar beet, and reduce Cercospora leaf spot (Bargabus et al., 2002). The B. mycoides isolate Bac J product was commercialized by Certis Biologicals (Columbia, MD, USA) as a biopesticide, and was granted registration till the year of 2016 by the U.S Environmental Protection Agency and Health Canada's Pest Management Regulatory Agency. With the market driven factors such as the technical advance in mass production, the increasing efficacy in biocontrol products, lower raw material costs, fast regulatory approvals, and favorable government initiatives to promote the adoption of biocontrol agents, the microbial agent market for agriculture is projected to grow (Huang, 2003). According to the 2021 Research and Markets report, the global biopesticide market reached a value of USD 3.96 billion in 2020 and is expected to exhibit strong growth from 2021 to 2026.
Probiotic microorganisms have multiple functions. Their significant beneficial effects on plants include protecting plants from pathogen infections, promoting the absorption and utilization of plant nutrients, and enhancing plant vigor (Chojnacka, 2015). Thus, they could be commercialized as biopesticides, biofertilizers, and biostimulants (Chojnacka, 2015). Probiotics such as well-known lactic acid bacteria also exhibit the potential to improve animal health, efficiency, and meat quality (Al-Shawi et al., 2020). In Taiwan, our transdisciplinary team with expertise in plant pathology, pesticide analysis, aquatic management, livestock production, and microbial fermentation aimed to develop a solution to eliminate the bottleneck effect during the mass production of biocontrol products and to expand the applications of probiotic products. We jointly discovered three probiotics (Bacillus mycoides BM02, Bacillus licheniformis EC34-01, and Bacillus subtilis 151B1), established a standard protocol of the fermentation and formulation technology using the pilot plant and industrial-scale facilities, and demonstrated their high potential for application in crop protection, circular agriculture, livestock farming, and aquaculture. Among the three probiotics, B. mycoides BM02-based products registered as biopesticides or biofertilizers are currently commercialized by several enterprises through technology transfer from the Intellectual Property Center of the National Chung-Hsing University. The mass production and application technology of B. subtilis 151B1 was also transferred to two enterprises for commercialization. The efficacy of these three probiotics and their potential mechanisms in plant protection is also discussed in this paper.
The biological control of plant diseases with introduced antagonists is a common strategy. However, the effect of modifying the balance of the flora and microbial ecology by augmenting a specific population can only be temporary (Garrett, 1965). (Huang, 2003) suggested that the ecological effect of biocontrol organisms on the environment should be considered to improve the efficacy of introduced biocontrol agents. Technical advances in next-generation sequencing have allowed us to understand the compositions of microbial communities associated with plants or animals. The effect of introducing Bacillus-based probiotics on the microbiome of the plant rhizosphere, hog feces, and fish guts is also discussed in the latter part of the paper.
Combating disease and pests without suitable and enough resources is always a challenge in organic cultivation. Here, we describe our experience in developing biological control agents through the stages of laboratory research to commercialization, from basic mechanism studies to field applications in Taiwan. B. mycoides BM02 products are licensed as biopesticides and biofertilizers and effectively control diseases and promote the growth of several crops. Additionally, our transdisciplinary team—led by Professor Jenn-Wen Huang—collaborated to explore and develop B. subtilis 151B1 and B. licheniformis agents EC34-01 (originally designed for plant protection) for multi-industry applications in sectors including crop health care, livestock farming, aquaculture, and agricultural pollutants.
BACILLUS-BASED PROBIOTIC PRODUCTS, APPLICATIONS, AND MECHANISMS
Bacillus-based probiotic products
B. mycoides is commonly found in soils and the plant rhizosphere or phyllosphere, exhibits rhizoid colony morphology on nutrient agar, and forms stress-resistant endospores (Buyer, 1995; Di Franco et al., 2002). B. mycoides BM02 isolated from a tomato rhizosphere in central Taiwan was selected from more than 1,000 isolated strains for their effects on crop growth promotion and disease control (Huang et al., 2018). Strain BM02 enhanced the growth of various plants, including tomato, lettuce, edible rape, cabbage, and asparagus bean, and effectively controlled tomato Fusarium wilt, cucumber damping-off, powdery mildew of tomato and cucumber, strawberry gray mold, cabbage clubroot, rice blast, scallion rust, and strawberry mites (Table 1; (Huang et al., 2018). The research results related to B. mycoides were granted two invention patents (Taiwan Patent Numbers I510619 and I486444) non-exclusively licensed to six enterprises. B. mycoides BM02 products with different formulations and processes are commercialized and licensed as biofertilizers (e.g., “Shen Zen Shui No. 2” and “Syun Meng Nong”, and biopesticides (e.g., “Jhih Huang Ye”) for the control of orchid Fusarium yellows in Taiwan.
B. subtilis and B. licheniformis are also endospore-forming bacteria. They are phylogenetically and phenotypically related and are, therefore, taxonomically arranged in the same “B. subtilis group” (Fritze, 2004). Both Bacillus species are certified as “Generally Recognized As Safe (GRAS)” bacteria by the US Food and Drug Administration. Moreover, they feature in the “Qualified Presumption of Safety (QPS)” list by the European Food Safety Authority and in the feed additives list by the Council of Agriculture, Executive Yuan in Taiwan, suggesting that they are safe for agriculture and human use (Sorokulova et al., 2008; Olmos and Paniagua-Michel, 2014). Here, we obtained B. subtilis 151B1 and B. licheniformis EC-34-01 from the rhizosphere of tea plants and soil-planted water chestnut in Taiwan, respectively (Chen et al., 2021; Chiang, 2011). Both strains possessed antagonistic activities against various plant pathogens, protected crops from pathogen infections, and promoted plant growth (Tables 1 and 2; Figures 2 and 3). The microbial agents of B. subtilis 151B1 and B. licheniformis EC34-01 were developed in liquid and powder formulations and spray-coating granules using a pilot plant and industrial-scale facilities to assess their efficacy in plant protection, pesticide degradation, livestock production, and aquaculture applications.
Plant disease suppression and growth promotion by three probiotics
Applications of B. mycoides BM02 products in the fields displayed a reduction in the disease severity (ranging from 18% to 66%) of various plant diseases, including cabbage damping-off, cucumber Pythium damping-off, tomato Fusarium wilt, rice sheath blight, rice blast, rice bacterial leaf blight, orchid Fusarium yellows, eggplant bacterial wilt, strawberry Fusarium wilt, and jewel orchid stem rot, compared to non-treated fields (Table 1; Figures 1A, B, and C). B. mycoides BM02 products not only suppressed plant diseases but also promoted the growth of strawberry and rice plants, as well as enhanced the yield of rice and strawberry fruits by up to 67% (Table 2; Figures 2A, C, and E).
B. subtilis 151B1 in liquid formulations was effective in suppressing passion fruit Fusarium disease, passion fruit anthracnose, tea brown blight, cucumber damping-off, cucumber Fusarium wilt, cucumber downy mildew, and tomato bacterial wilt (Table 1; Figure 1D). Application of B. subtilis 151B1 in the field promoted the growth of cucumber plants (a twofold increase in height was observed in treated plants, compared with untreated controls) and increased the yields of passion fruit, tea, and cucumber (Table 2; Figures 2B and D). Compared with untreated plants, the average yield of cucumber per day increased to 29.6% in plants treated with B. subtilis 151B1 liquid formulations (Table 2; Figure 2D). The cucumber yield further increased to 40.3% when a combination of B. subtilis 151B1 and CH100 plant extracts was used (Figure 2 D). CH100 is a commercial plant nutrient developed using agricultural waste containing extracts of tobacco debris and cabbage leaves, and SH soil amendment (Huang, 1992 ; Sun and Huang, 1985).
The application of B. licheniformis EC34-01 in liquid formulations can control mango anthracnose, cucumber damping-off, and cucumber Fusarium wilt (Table 1). Additionally, the application of B. licheniformis EC34-01 once per week for five weeks to 20-year-old tea plants in the field significantly enhanced tea yield to 277%, compared with untreated plants (Table 2).
Mechanisms of disease control and plant growth promotion
The efficacy of B. mycoides BM02, B. subtilis 151B1, and B. licheniformis EC-34-01 applications on plant disease control and plant growth promotion have been demonstrated under laboratory, greenhouse, and field studies. The mechanisms of B. mycoides BM02 in plant health protection are: (i) B. mycoides BM02 could colonize plant roots, proliferate in plant tissues, and induce systemic resistance in plants (Figure 3); (ii) B. mycoides BM02 could secrete plant growth hormones, e.g., indole-acetic acid (IAA) to promote root growth; (iii) B. mycoides BM02 could produce dimethyl-disulfide, phenylacetic acid, methylphenyl acetate, and surfactin, which can effectively disrupt the pathogens' cell membranes and inhibit the infection of plant pathogens (Huang et al., 2018; Wu et al., 2020). Our research indicated that B. subtilis 151B1 possessed plant growth-promoting traits (e.g., displaying activities in production of protease, amylase, cellulase, lipase, and IAA, as well as phosphorus solubilizing), thus suggesting its potential as a biofertilizer (Huang et al., unpublished data). B. subtilis 151B1 also could produce C14 and C15 family surfactins, as well as C14 and C15 family iturins (Chen et al., 2021). It has been suggested that the surfactins produced by B. subtilis 151B1 may trigger apoptotic-like cell death, reducing mitochondrial membrane potential and interfering with the energy metabolism of the plant pathogens (Chen et al., 2021). Additionally, B. subtilis 151B1 was shown to enhance the expression of plant defense genes, including the phenylalanine ammonia-lyase (PAL), peroxidase (POX), and pathogenesis-related (PR1a) genes, in cucumber seedlings after 24 h of inoculation, compared with control (water-treated) seedlings (Huang et al., unpublished data). Thus, B. subtilis 151B1 exhibits multiple mechanisms to control various plant diseases caused by pathogens and is suitable as a biopesticide. Acting as a biocontrol agent for plant diseases, B. licheniformis EC-34-01 was found to inhibit the mycelial growth of various fungal pathogens, including Colletotrichum gloeosporioides, Colletotrichum higginsianum, Colletotrichum orbiculare, and Alternaria brassicicola (Chiang, 2011), which may be attributed to the induction of the plant’s defense response. In contrast to B. subtilis 151B1, B. licheniformis EC-34-01 induces the expression of PAL, POX, and PR1a only after the cucumber seedlings are challenged with pathogens (Huang et al., unpublished data).
Applications of Bacillus-based probiotic products in the livestock farming and aquaculture
Due to concerns about antimicrobial resistance to global public health threats and the imbalance of ecosystem, as well as to achieve the Sustainable Development Goals, a reduction in the use of antibiotics to promote growth or prevent diseases in healthy animals was suggested by the World Health Organization (2020) and World Organization for Animal Health (2015). Bacillus-based probiotics are also used as potential alternatives to antibiotics in animal agriculture to improve animal health, growth efficiency, and meat quality (Al-Shawi et al., 2020; Verschuere et al., 2000). In our studies, microbial agents of B. subtilis 151B1 and B. licheniformis EC34-01 in powder formulations and spray-coating granules were assessed for their efficacy as feed additives in swine raising, as well as milkfish and shrimp farming (Huang, 2021). Our findings indicated that using B. subtilis 151B1 as a feed additive in the hogs’ diet increased their daily food intake by 6.1% and daily weight gain by 3.4%, as well as shortened the feeding time by 3 days, compared to control animals. It also improved the weight of pork tenderloin and the texture of short fillet (Figure 4A; (Huang, 2021); Lin et al., unpublished data). Additionally, B. subtilis 151B1 as a feed additive decreased the H2S and thiol contents in the hog feces by 25% and 81%, respectively, (i.e., 17.75 ppm and 0.15 ppm, respectively), compared with untreated control (23.50 ppm H2S and 0.79 ppm thiol contents), suggesting its potential for improving the farm environment (Lin et al., unpublished data). Our results also showed that applications of B. subtilis 151B1 and B. licheniformis EC34-01 as feed additives improved the feed conversion ratio and enhanced the growth of milkfish, with the treatment with strain EC34-01 being the most effective (Figure 4B; (Huang, 2021); Lu et al., unpublished data). Moreover, they reduced the populations of pathogenic Vibrio and improved the water quality of fish and shrimp breeding farms by reducing the ammonia, nitrate-nitrogen, and nitrite-nitrogen contents (Lu et al., unpublished data). These results suggested that both strains had excellent potential to be used as feed additives to promote the productive performance of livestock and aquatic animals.
Together with that of other researchers, our work demonstrated the use of B. subtilis and B. licheniformis exert numerous beneficial effects on animals (Al-Shawi et al., 2020; Verschuere et al., 2000). These two probiotics are good alternatives for improving feed utilization, leading to enhanced growth performance, stress response, immune response, disease resistance, and meat and water quality for sustainable livestock farming and aquaculture (Al-Shawi et al., 2020; Kuebutornye et al., 2019). The proposed mechanisms of these two probiotics may be attributed to enhance the digestive enzyme activity, antioxidant enzyme activity, and expression of immune-related as well as stress-related genes, produce antimicrobial compounds (organic acids, hydrogen peroxidase, bacteriocins, and biosurfactants), and modulate the gut microbiota (Kuebutornye et al., 2019; Ahmadnia Motlagh et al., 2012). For example, B. subtilis and B. licheniformis improved the growth of brine shrimp (Artemia urmiana) by increasing digestive enzyme activities such as protease and amylase activities and enhancing the Bacillus population in the gut (Ahmadnia Motlagh et al., 2012). Tilapia fed with mixed products of B. subtilis and B. licheniformis showed improved growth and immune response, and were resistant to Streptococcus agalactiae infections (Abarike et al., 2018). Our results showed B. subtilis 151B1 and B. licheniformis EC34-01 could modulate the microbiota in chicken litters and the intestine of milkfish (discussed in a later section). B. subtilis 151B1 can produce protease, cellulase, lipase, amylase, and biosurfactants (C14 and C15 family surfactins, as well as C14 and C15 family iturin A) (Chen et al., 2021). Additionally, we also detected enhanced IL-2 and IFN-γ levels in the serum of piglets (Lin et al., unpublished data). Our unpublished data indicated that B. licheniformis EC34-01 produced genistein. Genistein is a compound commonly found in soybean-based fermentation broths containing B. licheniformis and is known to function as an antioxidant and anthelmintic (Yang et al., 2013). Whether genistein also plays a role in improving the growth performance, stress response, immune response, and disease resistance of milkfish treated with B. licheniformis EC34-01 remains to be determined.
Degradation of agricultural pollutants
Agricultural pollutants may come from wastes generated from plant cultivation, livestock raising, and fisheries. For instance, chemical pesticides are often found in agricultural wastes, which, if left untreated before being used as compost, may raise safety concerns about its recycling. The manure obtained from livestock raising is also a concern for the farming environment. Furthermore, the nitrogen contents greatly impact the water quality of aquaculture farms. Microorganisms could play important roles in the bioremediation of pollutants (Sarker et al., 2021; Hlordzi et al., 2020). Here, we found that B. subtilis 151B1 and B. licheniformis E34-01 could degrade chemical insecticides (e.g., marathon and deltamethrin) and a fungicide (e.g., tricyclazole) (Figure 5)—a property that could solve the problem of potential pesticide residue when recycling agricultural by-products. The H2S and thiol contents in the feces of hogs fed with the B. subtilis 151B1 additive were decreased (17.75 and 0.15 ppm, respectively) compared to the feces of control animals (23.50 and 0.79 ppm, respectively). The concentrations of ammonium-nitrogen, nitrite-nitrogen, and nitrate-nitrogen in the water of milkfish raising farms were reduced following treatment with B. subtilis 151B1 and B. licheniformis E34-01, compared to the control (untreated) farm water (data not shown). These data suggested that adding B. subtilis 151B1 and B. licheniformis E34-01 to the livestock or fish feeds may improve the farm environment and water quality.
Modulation of microbiomes by the probiotics
Probiotics can improve the intestinal microbial balance, consequently improving feed utilization, promoting growth, strengthening the immune response, and suppressing pathogen infections in humans and animals (Fuller, 1989; Saarela et al., 2000). Understanding the interactions between microorganisms and hosts could offer insights into the beneficial applications of utilizing and manipulating microbes (Mahmud et al., 2021; Trivedi et al., 2020; Trivedi et al., 2021). The rhizosphere microbiome is referred to as the second or extended genome for maintaining plant health (Berendsen et al., 2012). Using next-generation sequencing analysis, we found shifts in the bacterial communities of the passion fruit rhizosphere following the introduction of the B. subtilis strain 151B1. Comparing the enriched bacterial genera identified in the rhizosphere applying strain 151B1 with the control environment, we detected Streptomyces spp. (10.3% vs. 6.6%), Bacillus spp. (7.1% vs. 3.2%), Pyrinomonadaceae RB41 (5.4% vs. 2.8%), Paraburkholderia spp. (6.4% vs. 2.0%), Nocardioides spp. (4.8% vs. 2.8%), Nitrospira spp. (4.2% vs. 1.9%), Bradyrhizobium spp. (3.8% vs. 1.6%), and Nitrosomonadaceae MND1 (4.1% vs. 1.3%); by contrast, the reduced bacterial genera included Chujaibacter spp. (12.1% vs. 20.3%), Rhodanobacter spp. (3.8% vs. 7.6%), Alcanivorax spp. (0.5% vs. 8.8%), Candidimonas spp. (2.6% vs. 4.2%), and Arachidicoccus spp. (1.4% vs. 4.4%) (Figure 6A). Among the enriched bacterial genera, most of the known Streptomyces spp., Bacillus spp., Paraburkholderia spp., and Bradyrhizobium spp. harbored plant growth-promoting features (Ormeño-Orrillo and Martínez-Romero, 2019; Berg, 2009). The Nitrosomonadaceae family comprises Nitrosomonas and Nitrosospira spp.; the latter are ammonia oxidizers, i.e., they oxidize ammonia to nitrite, which is subsequently oxidized by bacterial nitrite oxidizers to nitrate (Daims et al., 2015). Nocardioides strains play an important role in bioremediation (Yoon and Park, 2006).
Similarly, a decrease in the pathogenic Fusobacteriales and Vibrionales populations and an increase in the beneficial Lactobacillales in the gut microbiota of milkfish treated with B. subtilis 151B1 and B. licheniformis EC34-01 strains were found, suggesting the potential of these strains in reducing the incidence of fish diseases (Figure 6B). Additionally, B. subtilis and B. licheniformis as feed additives improve the water quality of aquaculture farms and modulate the diversity of macroalgae (the source of fish food) in the farming water (data not shown).
FUTURE PERSPECTIVES
The concept of biological control originated from managing soil suppressiveness using natural microorganisms. Biological control using introduced antagonists has become a common strategy nowadays (Cook, 1990). However, there are technical, regulatory, and marketing hurdles to overcome for the commercialization, application, and global sale of microbial biocontrol agents. From our experience developing Bacillus-based products, teamwork with transdisciplinary experts from academic and private sectors could accelerate the research, development, and commercialization of biocontrol products. Using a pilot plant facility to mass-produce the biocontrol agents and having the efficacy of product prototypes evaluated by experts in their fields of study would not only minimize the gap between laboratory research and commercialization but also validate their efficacy and provide the scientific-based technical dossiers for product registration and application. Furthermore, because the regulatory policy and dossier requirements to register biocontrol agents are different in each country, the involvement of government departments to harmonize the regulations and quarantine measures would facilitate the global market sale of the biocontrol products.
Next-generation sequencing technologies have allowed researchers to access and explore the diversity and complexity of the rhizosphere, environmental, and gut microbiomes. By comparing bacterial communities with and without treatment of probiotics in the rhizospheres of passion fruit, chicken litter, and milkfish gut, we found that the introduction of B. subtilis 151B1 and B. licheniformis EC34-01 did not affect bacterial diversity but modulated the composition of bacterial microbiomes by enhancing the beneficial population and reducing the potentially pathogenic population. These findings further suggested that the introduction of probiotics in crop fields could maintain the balance of the microbial ecosystem, similar to our findings, as well as those of other studies, in the animal system. Thus, probiotics are excellent candidates as alternatives to chemical pesticides, fertilizers, and feed additives for sustainable agriculture.
Probiotic bacteria could serve as an alternative solution to control diseases and pests, particularly in, but not limited to, organic farming. A selection of compatible chemical pesticides containing probiotics that target specific modes of action would expand the use of probiotic products in conventional farming practices. The use of probiotics in integrated pest management systems could serve as a resistance management tool and ensure the safety of agricultural products, especially those with short pre-harvest and re-entry intervals, and without the issue of maximum residue limits. Additionally, based on our data, probiotic bacteria could further serve as a pesticide degrader, which is important to reduce pesticide residues in agricultural products and by-products. Because probiotics are live organisms, choosing the right kind at the right time to use in the integrated pest management system is a critical process to ensure their highest efficacy. Input of professional knowledge from Plant Doctor, Pest Control Advisor, or Plant Protection practitioner to work with the growers would advance the application practice of probiotics and in turns maximizing the efficacy.
ACKNOWLEDGMENTS
The research was supported by and the Ministry of Science and Technology, Taiwan (MOST 110-2321-B-005-006), and the “Innovation and Development Center of Sustainable Agriculture” from the Featured Area Research Center Program within the framework of the Higher Education Sprout Project by the Ministry of Education in Taiwan. The authors thank Miss Yu-Hsuan Chen in Dept. Plant Pathology, National Chung Hsing University for designing graphical abstract.
CONFLICT OF INTEREST
The authors declare no conflict of interest.
ORCID
Tzu-Pi Huang https://orcid.org/0000-0003-2904-3373
Jenn-Wen Huang https://orcid.org/0000-0003-0798-4332
Chuan-Shun Lin https://orcid.org/0000-0002-9879-6101
Wen-Hsin Chung https://orcid.org/0000-0002-8517-6368
REFERENCCES
Abarike E. D., Cai J., Lu Y., Yu H., Chen L., Jian J., Tang J., Jun L., Kuebutornye F. K. A. (2018). Effects of a commercial probiotic BS containing Bacillus subtilis and Bacillus licheniformis on growth, immune response and disease resistance in Nile tilapia, Oreochromis niloticus. Fish & Shellfish Immunology, 82, 229-238.
Ahmadnia Motlagh H. R., Farhangi M., Rafiee G., Noori F. (2012). Modulating gut microbiota and digestive enzyme activities of Artemia urmiana by administration of different levels of Bacillus subtilis and Bacillus licheniformis. Aquacult. International, 20, 693-705.
Al-Shawi S. G., Dang D. S., Yousif A. Y., Al-Younis Z. K., Najm T. A., Matarneh S. K. (2020). The potential use of probiotics to improve animal health, efficiency, and meat quality: a review. Agriculture, 10, 452.
Baker C. J., Stavely J. R. (1985). Biocontrol of bean rust by Bacillus under field conditions. Plant Disease, 69, 770-772.
Bargabus R. L., Zidack N. K., Sherwood J. E., Jacobsen B. J. (2002). Characterisation of systemic resistance in sugar beet elicited by a non-pathogenic, phyllosphere-colonizing Bacillus mycoides, biological control agent. Physiological and Molecular Plant Pathology, 61, 289-298.
Berendsen R. L., Pieterse C. M. J., Bakker P. A. H. M. (2012). The rhizosphere microbiome and plant health. Trends in Plant Science, 17, 478-486.
Berg G. (2009). Plant–microbe interactions promoting plant growth and health: perspectives for controlled use of microorganisms in agriculture. Applied Microbiology and Biotechnology, 84, 11-18.
Buyer J. S. (1995). A soil and rhizosphere microorganism isolation and enumeration medium that inhibits Bacillus mycoides. Applied and Environmental Microbiology, 61, 1839-1842.
Chen Y.-H., Lee P.-C., Huang T.-P. (2021). Biological control of collar rot on passion fruits via induction of apoptosis in the collar rot pathogen by Bacillus subtilis. Phytopathology, 111, 627-638.
Chiang C.-J. (2011) Identification for biocontrol agents of mango anthracnose and design of their pilot-scale fermenting nutrient recipes for the disease control Ms. Sci. Master thesis, National Chung Hsing University.
Chojnacka K. (2015). Innovative bio-products for agriculture. Open Chemistry, 13, 932-937.
Cook R. J. (1990). Twenty-five years of progress towards biological control. In: Biological Control of Soil-Borne Plant Pathogens, pp. 1-14 Ed D. Hornby. Wallingford, UK.: CAB International
Daims H., Lebedeva E. V., Pjevac P., Han P., Herbold C., Albertsen M., Jehmlich N., Palatinszky M., Vierheilig J., Bulaev A., Kirkegaard R. H., von Bergen M., Rattei T., Bendinger B., Nielsen P. H., Wagner M. (2015). Complete nitrification by Nitrospira bacteria. Nature, 528, 504-509.
Di Franco C., Beccari E., Santini T., Pisaneschi G., Tecce G. (2002). Colony shape as a genetic trait in the pattern-forming Bacillus mycoides. BMC Microbiology, 2, 33.
Fritze D. (2004). Taxonomy of the genus Bacillus and related genera: the aerobic endospore-forming bacteria. Phytopathology, 94, 1245-1248.
Fuller R. (1989). Probiotics in man and animals. J. Appl. Bacteriol., 66, 365-378.
Garrett S. D. (1965). Toward biological control of soil-borne plant pathogens. In: Ecology of Soil-Borne Plant Pathogens Prelude to Biological Control, pp. 423-435 Eds K. F. Baker & W. C. Snyder. Berkeley, California, USA: University of California Press.
Hlordzi V., Kuebutornye F. K. A., Afriyie G., Abarike E. D., Lu Y., Chi S., Anokyewaa M. A. (2020). The use of Bacillus species in maintenance of water quality in aquaculture: A review. Aquaculture Reports, 18, 100503.
Huang H.-C. (2003). Biocontrol of plant disease: research and application. In: Advances in Plant Disease Management 2003, pp. 1-18 Eds H.-C. Huang & S. N. Acharya. Trivandrum, India: Research Signpost.
Huang J.-S., Peng Y.-H., Chung K.-R., Huang J.-W. (2018). Suppressive efficacy of volatile compounds produced by Bacillus mycoides on damping-off pathogens of cabbage seedlings. The Journal of Agricultural Science, 156, 795-809.
Huang J. W. (1992 ). Integrated management of vegetable seedling pests with a formulated plant nutrition. Plant Protection Bulletin, 34, 54-63.
Huang T.-P. (2021) Probiotic microbiomes for agriculture safety and green gold creation. In: MOST Center for Golobal Affairs and Science Engagement (GASE) Newsletter - Taiwan Research Highlight, p. https://trh.gase.most.ntnu.edu.tw/en/article/content/207. Taipei, Taiwan: Ministry of Science and Technology.
Kuebutornye F. K. A., Abarike E. D., Lu Y. (2019). A review on the application of Bacillus as probiotics in aquaculture. Fish & Shellfish Immunology, 87, 820-828.
Mahmud K., Missaoui A., Lee K., Ghimire B., Presley H. W., Makaju S. (2021). Rhizosphere microbiome manipulation for sustainable crop production. Current Plant Biology, 27, 100210.
Olmos J., Paniagua-Michel J. (2014). Bacillus subtilis a potential probiotic bacterium to formulate functional feeds for aquaculture. Journal of Microbial & Biochemical Technology, 6, 361-365.
Ormeño-Orrillo E., Martínez-Romero E. (2019). A genomotaxonomy view of the Bradyrhizobium genus. Frontiers in Microbiology, 10.
Saarela M., Mogensen G., Fondén R., Mättö J., Mattila-Sandholm T. (2000). Probiotic bacteria: safety, functional and technological properties. Journal of Biotechnology, 84, 197-215.
Sarker A., Nandi R., Kim J.-E., Islam T. (2021). Remediation of chemical pesticides from contaminated sites through potential microorganisms and their functional enzymes: Prospects and challenges. Environmental Technology & Innovation, 23, 101777.
Sorokulova I. B., Pinchuk I. V., Denayrolles M., Osipova I. G., Huang J. M., Cutting S. M., Urdaci M. C. (2008). The safety of two Bacillus probiotic strains for human use. Diggestive Diseases and Sciences, 53, 954-963.
Sun S. K., Huang J. W. (1985). Formulated soil amendment for controlling Fusarium wilt and other soilbrone diseases=. Plant Disease, 69, 917-920.
Trivedi P., Leach J. E., Tringe S. G., Sa T., Singh B. K. (2020). Plant–microbiome interactions: from community assembly to plant health. Nature Review of Microbiology, 18, 607-621.
Trivedi P., Mattupalli C., Eversole K., Leach J. E. (2021). Enabling sustainable agriculture through understanding and enhancement of microbiomes. New Phytologist, 230, 2129-2147.
Verschuere L., Rombaut G., Sorgeloos P., Verstraete W. (2000). Probiotic bacteria as biological control agents in aquaculture. Microbiology and Molecular Biology Reviews, 64, 655-671.
World Health Organization (2020) World Health Organization antimicrobial resistance fact sheet. In.
World Organization for Animal Health (2015) Antimicrobial resistance fact sheets [Online]. Available: https://www.oie.int/fileadmin/Home/eng/Media_Center/docs/pdf/Fact_sheets/ANTIBIO_EN.pdf [Accessed].
Wu J.-J., Huang J.-W., Deng W.-L. (2020). Phenylacetic acid and methylphenyl acetate from the biocontrol bacterium Bacillus mycoides BM02 suppress spore germination in Fusarium oxysporum f. sp. lycopersici. Frontiers in Microbiology, 11, 569263 doi: 569210.563389/fmicb.562020.569263.
Yang H. J., Kwon D. Y., Moond N. R., Kimb M. J., Kang H. J., Jungc D. Y., Park S. (2013). Soybean fermentation with Bacillus licheniformis increases insulin sensitizing and insulinotropic activity. Food & Function, 4, 1675-1684.
Yoon J.-H., Park Y.-H. (2006). The Genus Nocardioides. Prokaryotes, 3, 1099-1113.