ABSTRACT
Silicon (Si), an element useful to plants, is abundant in soil, mostly in the form of insoluble silicates. Monosilicic acid, which is soluble, is the only form of Si that plants can take up. The bioavailability of Si reduces several biotic and abiotic stressors to plants and enhances their growth and productivity, while a deficiency of Si leads to abnormalities in plant growth and development. Synthetic silica-based fertilizers are expensive to produce, while the continuous and long-term application of silicate fertilizers can have harmful effects on the soil, as hazardous substances may deposit in the soil in addition to Si. In contrast, the application of biofertilizers based on silicate-solubilizing microbes could be an effective way not only to increase the bioavailability of Si but also to reap the benefits of Si for plants. In this context, this review addresses the distribution, isolation and characterization of silicate solubilizing microorganisms (SSMs), as well as the mechanism of Si solubilization and the promotion of plant growth by SSMs. In addition, crop production and protection of SSM-based biofertilizers are summarized, and problems and potential solutions for the use of SSM-based biofertilizers in agriculture are discussed. The findings presented here will be useful to better explore the potential of SSM-based biofertilizers in sustainable agriculture.
Keywords: Si-based fertilizer; Si bioavailability; phosphate solubilizing microbes; sustainable agriculture
INTRODUCTION
Silicon (Si) is the second most abundant element (~27%) in the Earth’s crust and occurs almost exclusively in the form of silicon dioxide (SiO2) in association with a wide range of Si-bearing minerals in crystalline, weakly crystalline, and amorphous phases. Although abundant in the Earth’s crust, Si is mostly in insoluble forms that are difficult for plants to absorb. Silicic acid (H4SiO4) is most common available form of Si in the soil. It becomes available mainly through the biogenic weathering and dissolution of the primary and secondary minerals (Sharma et al., 2022).
The Si uptake ability of plants is genetically controlled. According to the Si uptake ability, plants are divided into three categories: high accumulators (> 5% Si on a dry weight basis, such as rice, sugarcane, maize, bamboo, and sorghum), intermediate accumulators (about 1% Si, such as wheat, cucumber, chrysanthemum, and pumpkin), and low accumulators (< 1% Si, such as tomato and sunflower) (Meena et al., 2014). There has been a great deal of debate about the essentiality of Si to plants due to its abundance in soil, its accumulation pattern, and its observed positive effects on plants. Due to the lack of compelling data demonstrating its direct contribution to plant metabolism, it was ultimately excluded from the category of essential elements (Sharma et al., 2022). However, there is mounting evidence that Si application in agricultural fields can alleviate biotic and abiotic stress in plants and improve plant growth and yield (Raturi et al., 2021; Sharma et al., 2022). As a result, Si has been designated as a "quasi-essential" element and recognized as a beneficial nutrient. The International Plant Nutrition Institute (IPNI, www.ipni.net/nutrifacts), considering Si's numerous advantageous impacts on plants, has acknowledged Si as a beneficial nutrient for plant (Sharma et al., 2022).
Si fertilizer use may benefit both plants and soil in complementary ways. For the plant system, increased bioavailability of Si increases the deposition of Si in plant tissues, improves tissue rigidity, and helps the plant tolerate biotic stress (pest attack and pathogens) and abiotic stress (salinity, drought, nutrient imbalance, heavy metal and metalloid toxicity, heat and cold stress, and radiation stress) (Shivaraj et al., 2022). For the soil system, adequate availability of Si can improve the availability of other nutrients, especially phosphorus (P) and potassium (K), improve soil physicochemical properties (e.g., soil pH) and biological properties, and thus improve overall soil health and fertility (Das et al., 2019; Etesami and Schaller, 2023). Increasing bioavailability of Si has been reported to enhance photosynthesis and carbon (C) assimilation (Meena et al., 2014). The use of Si fertilizers in agricultural fields can minimize the use of pesticides (by 40–70%), mineral fertilizers (10–40%) and irrigation water (30–40%) without affecting crop productivity (Sharma et al., 2022). The beneficial effects of Si on the soil-plant system have been documented at the genetic level. For instance, there has been a growing body of evidence suggesting that Si plays multiple roles in the regulation of genes involved in plant photosynthesis, secondary metabolism, polyamine biosynthesis, transcription, and water absorption (Manivannan and Ahn, 2017). Physiological improvements in plant growth and recovery from stress were induced by molecular-level modulations triggered by Si supplementation (Manivannan and Ahn, 2017). Also, for the soil system, Si fertilization was reported to significantly change the composition of the bacterial and fungal community in the soil, increasing the abundance of functional genes involved in labile C degradation, C and N fixation, phosphorus utilization, CH4 oxidation, and metal detoxification, while decreasing genes involved in CH4 production and denitrification. The changes in the taxonomic and functional structure of microbial communities as a result of Si fertilization were mainly controlled by the changes in soil pH, plant photosynthesis, and Si nutrient availability induced by Si fertilization (Das et al., 2019, 2020a, 2021).
A variety of Si fertilizers, including natural, synthetic, liquid, and solid fertilizers, as well as various industrial by-products, are currently on the market. There are various types of Si fertilizers that are commercially available, including slag-based silicate fertilizers (calcium silicate fertilizers), fused magnesium phosphate fertilizers, fused potassium magnesium silicate fertilizers, porous hydrated calcium silicate fertilizers, silica gel, soluble silicates like sodium, potassium, and calcium silicates, and natural resources like wollastonite, olivine, diatomaceous earth, natural zeolite, and pyroxene (Das et al., 2020b; Raturi et al., 2021; Sharma et al., 2022). However, the most commonly used silicate fertilizers, water-soluble sodium silicate fertilizers and water-soluble potassium silicate fertilizers, are still too expensive to use in traditional agriculture. To benefit from Si's numerous advantages in agriculture, less expensive Si sources should be used. The application of Si fertilizers also causes numerous problems, including increased soil pH, declined digestibility of straw, and issue related to increased production costs (Thorne et al., 2020). In contrast, biofertilizers produced from competent silicate-solubilizing microorganisms (SSMs) signify a more environmentally sound and economically viable alternative to improve Si availability and hence agricultural output. Therefore, this review aims to evaluate the latest knowledge on SSMs, distribution, characterization and isolation of SSMs, the mechanism of Si solubilization by SSMs, the role of SSMs in crop production and protection, and limitations and future research priorities.
SILICATE-SOLUBILIZING MICROORGANISMS (SSMS): DISTRIBUTION, ISOLATION, AND CHARACTERIZATION
There have been reports that species belonging to the bacterial genera Bacillus, Paenibacillus, Acidobacillus, Pseudomonas, Proteus, Rhizobia, Burkholderia, Aminobacter, Enterobacter, and Sphingobacterium, and fungal genera such as Aspergillus, Trichoderma, and Penicillium can solubilize a variety of silicates, including magnesium silicate, quartz, feldspar, and others (Table 1).
Table 1. Silicate solubilizing microbes, their isolation source, characterization and effect on plant growth.
Silicate solubilizing microbes
|
Isolation source
|
Solubilized insoluble Si
|
PGP traits
|
Effects on plant
|
References
|
Burkholderia eburnea CS4-2
|
Rice rhizosphere
|
Magnesium trisilicate
|
Indole-3-acetic acid (IAA) production
|
Increased rice growth and yield
|
Kang et al. 2017
|
Enterobacter ludwigii GAK2
|
Paddy soil
|
Magnesium trisilicate
|
Phosphate (P) solubilization and production of IAA and gibberellic acid
|
Promoted rice seed germination and increased root length, shoot length, fresh biomass, and chlorophyll content of rice plants
|
Lee et al. 2019
|
Serratia marcescens and
Pseudomonas aeruginosa
|
Rice rhizosphere
|
Magnesium trisilicate
|
Phosphate solubilization and production of IAA
|
Increased rice growth and yield
|
Ng et al. 2016
|
Rhizobium sp. IIRR-1
|
Rice rhizosphere
|
Inorganic (Ca, Al, K and Mg), diatomaceous earth, siliceous earth, rice husk and, rice straw
|
Solubilization of phosphate, zinc, and potassium, and production of IAA and 1-aminocyclopropane-1-carboxylate deaminase
|
Improved rice seedling vigor
|
Chandrakala et al. 2019
|
Bacillus sp.
|
clay substrate of a mountain territory
|
Sodium metasilicate
|
-
|
Increased photosynthesis and total N, P, and K content in the leaves of Brassica. Juncea
|
Maleva et al. 2017
|
Pseudomonas and Bacillus sp., B. amyloliquefaciens, Sphingobacterium sp.
|
Rhizosphere soils
|
Magnesium trisilicate, talc, and feldspar
|
Production of organic acids (gluconic, succinic, fumaric, tartaric, and maleic acid)
|
Increased Si uptake, reduced disease severity, modulation of cell wall degrading and antioxidative enzyme activities, and induced defense responsive gene expression in rice plant
|
Bist et al. 2020
|
Aeromonas, Bacillus, Cellvibrio, Ensifer, Flavobacterium, Microbacterium, Paracoccus, Pseudomonas, Rhizobium, and Streptomyces
|
Gut and surrounding soil of earthworm
|
feldspar and quartz powder
|
-
|
Enhanced Si uptake and accumulation in maize plants and promoted seedling growth
|
Hu et al. 2018
|
Kosakonia sp.
|
Bryophyte Hypnum plumaeforme rhizoids
|
feldspar and quartz powder
|
-
|
Improved Si uptake and accumulation in maize plants, and promoted seedling growth
|
Hu et al. 2019
|
Rhizobium tropici
|
Weathered rocks
|
Feldspar and biotite
|
Production of organic acids and polysaccharides
|
-
|
Wang et al. 2015
|
Pseudomonas sp., P. frederiksbergensis, P. baetica,
P. stutzeri,
P. cremoricolorata, P. moorei,
P. plecoglossicida,
P. koreensis,
P. teessidea,
P. monteilii, Aeromonas sp.,
A. encheleia,
A. jandaei,
Bacillus pumilus, B. marisflavi,
B. ginsengisoli, and Enterobacter ludwigii
|
Rice rhizosphere
|
Magnesium trisilicate
|
Production of indole-3- acetic acid and 1-aminocyclopropane-1-carboxylate deaminase, N2 fixation, and the solubilization of phosphate
|
-
|
Cruz et al. 2022
|
Bacillus mucilaginosus
|
Rhizosphere soil of rice
|
Magnesium trisilicate
|
-
|
-
|
Vijayapriya et al. 2019
|
Bacillus flexus,
B. mucilaginosus, B. megaterium and Pseudomonas fluorescens
|
Soil samples from red soil, plantation soil, sea sand, pond sediment, sea water
|
Magnesium trisilicate, feldspar, calcium aluminosilicate, sodium aluminosilicate, talc, muscovite, illite and quartz
|
-
|
-
|
Vasanthi et al. 2018
|
Bacillus species (i.e., B. safensis, B. subtilis, B. caldolyticus, B. globisporus, B. stratosphericus, and B. cereus) and Pseudomonas species (i.e., P. fluorescens, P. alcaliphila, P. chengduensis, P. nitroreducens, P. veronii, and P. stutzeri) are the dominated silicate solubilizer found in environments. Silicon-solubilizing fungi (SSFs) include Aspergillus niger, Trichoderma sp., T. polysporum, T. viride, and T. pseudokoningii (Sharma et al. 2023). As a central component of the biogeochemical cycling of Si, SSMs are ubiquitous and primarily found in soils, water, aquatic sediment, mineral ores, weathered rocks, and the rhizospheres of plants. As rice and sugarcane require high levels of Si, the rhizospheres of these plants have been extensively investigated for silicate-solubilizing bacteria (SSBs). Silicon cycling and Si dynamics in forest ecosystems are reported to be responsible for Si deposition in aquatic environments such as rivers, streams, lakes, and oceans. However, isolation of SSMs from forest soils has not received much attention. Bamboo forests, for example, are considered a major source of bioavailable Si in rivers due to the hyper accumulative behavior of the bamboo plant. However, little effort has been devoted to identify SSMs from bamboo soils (Raturi et al., 2021). Deshmukh et al., (2020) documented 456 different plants that can uptake Si at high levels. This suggests that isolation of SSMs from the rhizosphere of these plants could be facilitated using such a comparative data set.
Although SSBs are ubiquitous, their numbers are very small compared to the large number of bacteria. Isolation of SSB involves several steps, such as collecting samples, purifying SSB, screening for microorganisms that dissolve insoluble silicate, and identifying the bacteria. Environments rich in Si, such as the rhizosphere of Si-accumulating plants (e.g., rice and sugarcane), Si-containing minerals, and Si-rich sediments are ideal for isolating SSB. After the sample (soil, sediment, or water) is collected, it is serially diluted in saline (typically 0.85%) and then an aliquot (0.1 mL) of the appropriate dilution is inoculated into the nutrient broth. A variety of growth media can be used to isolate SSB, such as culture medium enriched in magnesium trisilicate (Mg2O8Si3, 0.25%) to distinguish SSB from other bacteria (Raturi et al., 2021). In order to isolate SSB, researchers also employed glucose agar medium that contained insoluble silicate. A modified Bunt and Rovira medium supplemented with 0.25% magnesium trisilicate was used by several researchers to culture SSB. A nutrient medium ‘NBRISSM’, which contains magnesium trisilicate in concentrations ranging from 26.63 to 37.50 g ml-1 as a source of Si, can distinguish between SSB and other phosphate solubilizing bacteria. Zone formation on agar plates cultured for 7 days at 28 °C is often the basis for screening bacterial isolates for Si-solubilization. In addition, the Si solubilization potential of bacteria in broth medium can also be estimated by quantifying the Si released. A modified method of Elliott and Snyder (1991) described by Bist et al., (2020) is commonly used to screen SSB, where three volumes of 2.5% boric acid and an equal volume of 5.4% ammonium molybdate were added to the supernatant of 1, 3, 5, 7, and 10-day-old cultures in Si medium and then incubated for 5 min. 20% tartaric acid and 0.5% ascorbic acid sodium salt were added to the total volume of 1 ml and absorbance was measured spectrophotometrically at 650 nm (Bist et al., 2020).
To characterize SSB, polyphasic taxonomic approaches have been used, including physiological and biochemical tests. SSB colonies can be preliminarily examined for phenotypic characteristics such as cell shape, morphology, size, cell arrangement, Gram staining, and sporulation. The biochemical tests that can be performed to characterize SSB include catalase production, H2S production, motility, acid fast, glucose, fructose, sucrose, starch, and mannitol fermentability, urease testing, nitrate reduction, citrate utilization, organic acid quantification, and growth activity at various pH levels and temperatures. Various temperatures can be used to test the growth and activity of SSB. Most of the SSB strains grow well at temperatures ranging from 4°C to 45°C. Furthermore, the identification of SSBs can be accomplished by sequencing the 16S rRNA gene, which can be utilized to determine their phylogenetic relationships. An understanding of the mechanism of Si solubilization can be gained by quantifying the production of organic acid and IAA by SSB. Phylogenetic analysis of SSB will be supported by morphological, biochemical, and molecular characterizations.
MECHANISM OF SI SOLUBILIZATION AND PLANT GROWTH PROMOTION BY SSMS
SSMs use a number of interrelated methods to dissolve and solubilize silicates, including: (i) decreasing pH by organic and inorganic acid production; (ii) the production of chelating metabolites and ligands; and (iii) nucleophilic attack and exchange reactions (Etesami and Schaller, 2023). The most prevalent of these mechanisms is the production of acids by SSMs for solubilizing silica. Due to the acidic conditions created by SSMs, the dissolving rate of silicates is shifted out of equilibrium, resulting in an accelerated process of silicate solubilization. The kind of organic acid produced is influenced by the diversity of the SSB; it has been found that succinic, citric, maleic, gluconic, fumaric, acetic, tartaric, and hydroxy propionic acids are involved in silicate solubilization in media containing feldspar, quartz, and magnesium trisilicates as Si sources (Raturi et al., 2021). Microbial metabolites, such as organic acids, extracellular enzymes, chelates, and siderophores weaken the insoluble silicate framework by forming complexes with cations on the mineral surface, and hence play a significant role in the solubilization of silicates. Various polymers produced by SSM, such as extracellular proteins and polysaccharides, can form biofilms and help microorganisms attach to mineral surfaces, thereby affecting mineral dissolution. Indirectly, biofilms prevent protons, ligands, organic acids and other ions from escaping the interface between minerals and water, which not only increases water retention capabilities but also enhances mineral weathering (Sharma et al., 2023). Other than exopolymers, elements of bacterial cell membranes such lipopolysaccharides, peptidoglycan, and teichoic acids can bind to inorganic silicate ions to aid solubilization. A study conducted recently by Bist et al., (2020) demonstrated a functional association between the formation of organic acids and the activity of acidic phosphatases in the solubilization of Si. Recent developments in cutting-edge next generation sequencing (NGS) allow for the prediction of genes implicated in the metabolic pathways of silicate solubilizing ligands, acids, exopolymers, and membrane transporters. For silicate mineral weathering, these topics can be further investigated.
The potential of SSMs to improve crop growth and yield is based on the ability of SSMs to release Si and other nutrients such as phosphorus (P) and potassium (K), PGP traits (e.g., phytohormone synthesis, phosphate solubilization, siderophore production, and N2 fixation), and biocontrol traits of SSMs that support plant survival under adverse conditions and improve plant growth and yield. In a recent review, Etesami and Schaller (2023) reported that: (i) Si availability increases dissolution of iron phosphates and release of P into soil solution by replacing phosphate at the surface of amorphous and crystalline iron phases (decreased P sorption by soil minerals); (ii) Si increases P availability in paddy soils by increasing the fraction of Fe (II) (e.g., vivianite) compared to the fraction of Fe(III) (e. g., goethite, ferrihydrite, lepidocrocite, or hematite), which have a higher tendency to bind P; (iii) Si increases the root organic anion (e.g., citric, acetic, aconitic, malic, lactic, oxalic, succinic, and fumaric acids) to mobilize inorganic phosphate in the rhizosphere by competing with P to form complexes with Al3+, Ca2+, and Fe3+ and lowering rhizosphere pH; and (iv) Si availability enhances P utilization in rice by reducing iron and manganese uptake (e.g., by down-regulating the expression of Mn transporter gene that have the high affinity to bind phosphates in plants). Studies also show that Si increases K content in shoots and roots of wheat plants and enhances survival of barley and sugarcane plants against salt stress by minimizing K production, although the underlying mechanism is unknown (Raturi et al., 2021). Brindavathy et al., (2012) reported that the application of a SSB (Bacillus edaphicus) with different Si sources improves sugarcane yield due to the continuous supply of Si by the SSB. According to Kang et al., (2017), Burkholderia eburnea CS4-2, which was isolated from the roots of japonica rice, has the capacity to produce IAA and solubilize Si, and when applied to rice plants, it improves growth characteristics and yield. Lee et al., (2019) reported that Enterobacter sp. isolated from a healthy rubber plantation produced organic acids (citric, acetic, and lactic acids), indole-3-acetic acid, and gibberellic acids (GA1 and GA3), solubilized Si, P, and K, and possessed an antagonist against Rigidoporous microporus (causal agent of white root rot of rubber trees) (Table 1).
CROP PRODUCTION AND PROTECTION WITH SSM-BASED BIOFERTILIZERS
SSM-based biofertilizers can improve crop production and protection by improving both soil and plant properties, such as nutrient availability (P, K, Si), soil properties (pH, CEC, etc.), root exudate production, root oxidative capacity, photosynthetic activity, water status, phytohormone biosynthesis, nutrient uptake, phenolic compound metabolism, antioxidant potential, lodging, pest and pathogen resistance, tolerance to extreme temperatures (heat and cold), drought, salinity, oxidative damage, UV-B protection, and metal and metalloid stress. (Figure 1).
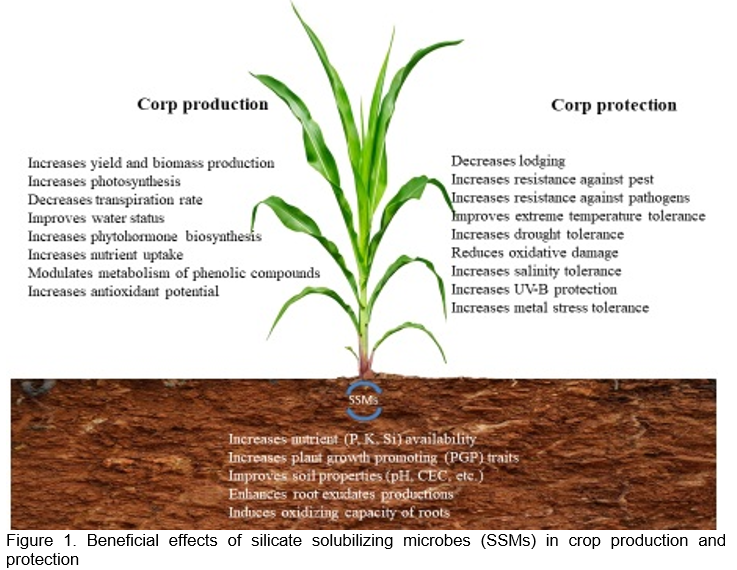
Fertilization with SSMs is a suitable approach for an integrated nutrient balance and a circular economy-model for managing agricultural waste, especially when combined with natural Si sources like grain husk and straw. A few Si-based biofertilizers have been developed and applied, but they are rare in practice. SSB and farmyard manure (FYM), with or without varying levels of fly ash, have been shown to increase Si uptake and concentrations in the straw and grain tissues in rice and the grain yield (Vasanthi et al., 2018). The release of cations (Ca2+ and Mg2+) as well as the volume of plastid material and photosynthetic pigments in leaves were all positively influenced by the use of SSM-based biofertilizers in combination with clay substrates in Brassica juncea (Indian mustard) and other crops (Maleva et al., 2017) (Table 1). According to reports, SSMs boost maize, wheat, tomato, and potato yields and rhizospheric microbial population (Raturi et al., 2021; Sharma et al., 2022). Therefore, SSM-based biofertilizer can be used as an efficient and environmentally benign fertilizer in organic and sustainable agriculture to improve crop production and reclaim infertile or damaged soils.
Silicon-based biofertilizers have been shown to reduce biotic as well as abiotic stresses like drought, high temperatures, ultraviolet radiation, lodging, freezing, salinity, nutrients imbalance, metal toxicity, pest and phytopathogen infestations like rice blast, powdery mildew, brown spot, stem rot, and sheath brown rot (Raturi et al., 2021; Sharma et al., 2022). The SSM controls abiotic stress by regulating antioxidant enzymes and decreasing free radicals to protect the oxidative defense system. After application of Si (80 kg ha-1) and chitosan (30 kg ha-1), Younas et al., (2021) found a significant increase in antioxidant/defense enzymes such as superoxide dismutase (SOD), peroxidase (POD), and catalase (CAT), indicating that Si fertilization eliminates reactive oxygen species (ROS) and reduces the severity of structural and functional damage in plants. Silicon availability has been shown to modify the physical properties of plant cell walls, such as loosening cells and cell processes to store more water and produce more stable lipids that help plants cope with drought, heat, and salt stress. In addition, Si accumulation can trigger specific defense responses, such as the formation of a cuticle-Si bilayer in epidermal cells, the formation of papillae, and the production of fungitoxic phenolic compounds such as flavonoids that protect plants from pest attack (Younas et al., 2021). Physical barriers prevent the spread of host-infecting toxins and lytic enzymes secreted by pathogens. It was shown that Si modify specific genes in a number of host-pathogen relationships, including those involving rice and Magnaporthe oryzae (Brunings et al., 2009), tomato and Rhizoctonia solani (Ghareeb et al., 2011), and wheat and Buleria graminis (Belanger et al., 2007). The wide range of prophylactic traits provided by SSMs reduce a wide range of abiotic stresses, such as drought, high temperature, and metal toxicity, and biotic stresses, such as rice blast, powdery mildew, brown spot, stem rot, rice yellow stem borer, and sheath brown rot, while promoting P, Mg, K, Fe, Cu, and Zn uptake by rice plants (Sharma et al., 2022). A nano-biofertilizer containing Si has been recently reported to improve nutrient (Mn, S, P, and K) translocation from soil to plant, minimize heavy metal uptake in food crops, and reduce UV stress. Silicon nano-biofertilizers containing SSBs such as Mesorhizobium sp. and Pseudomonas sp. have been shown to increase plant growth, soil structure, and fertility, and have been proposed as inventive Si sources with a variety of applications, including increasing plant tolerance to biotic and abiotic stressors, wastewater treatment, food processing, nano-additives in the paper industry, biosensors, and biomarkers (Sharma et al., 2022).
USE OF SSM-BASED BIOFERTILIZER IN AGRICULTURE: CONCERNS AND POTENTIAL SOLUTIONS
In order to introduce biofertilizer into the field, including SSM-based biofertilizer, a number of hurdles first have to be eliminated, including those related to survival, establishment, colonization, and plant interaction (for example, parasitic/symbiotic behavior, PGP performance) (Mitter et al., 2021). This is especially concerning when moving from the lab to the field since it frequently happens that a microbial strain that performed well in vitro may perform poorly in greenhouse and/or field trials. Because we generally consider and control a limited number of variables, we don't often take into account their complicated interactions when predicting inoculation outcomes. Possible solutions for large-scale production and success of biofertilizers in the field include: (i) pre-adapted microorganisms (e.g., locally isolated), isolation and screening with emphasis on PGP and ecological traits; (ii) mixed inoculants with functional redundancy but a wide range of environmental adaptations; (iii) personalized biofertilizers for a specific farm (e.g.., for a specific soil, crop, and management); (iv) biofertilizers based on an optimal performance spectrum; (v) genotype-specific inoculants (e.g., compatible microorganisms, pre-adapted microbiome); and (vi) optimized application timing.
CONCLUSIONS
The application of SSM-based biofertilizers to the soil may open new opportunities to increase crop production and transition to sustainable agriculture. It is currently unclear what molecular process enables different SSMs to solubilize Si. SSMs belonging to various genera and occurring in diverse environments suggest that different mechanisms of Si solubilization are involved. Future studies should focus on the biochemical and molecular basis of the mechanism of silicate solubilization. In addition to silicate solubilization, SSMs can also dissolve P and K. They may be beneficial to plants through the synthesis of organic acids such as IAA and their antagonistic activity against pathogens. SSMs can accelerate the biodegradation or composting of silicate-containing crop residues and convert them to a bioavailable form of Si. Therefore, SSM-mediated increase in Si bioavailability will contribute to reduced pesticide use and improved plant health and will be a milestone in sustainable agriculture.
REFERENCES
Bélanger, R.R., Benhamou, N., Menzies, J.G., 2003. Cytological evidence of an active role of silicon in wheat resistance to powdery mildew (Blumeria graminis f. sp. tritici). Phytopathology 93: 402-412.
Bist, V., Niranjan, A., Ranjan, M., Lehri, A., Seem, K., Srivastava, S., 2020. Silicon-solubilizing media and its implication for characterization of bacteria to mitigate biotic stress. Front. Plant Sci. 11: 28.
Brindavathy, R., Dhara, N., Rajasundari, K., 2012b. Biodissolution of silica by silicon bacteria in sugarcane rhizosphere. Res. J. Agr. Sci 3: 1042–1044.
Brunings, A.M., Datnoff, L.E., Ma, J.F., Mitani, N., Nagamura, Y., Rathinasabapathi, B., Kirst, M., 2009. Differential gene expression of rice in response to silicon and rice blast fungus Magnaporthe oryzae. Ann. Appl. Biol. 155: 161-170.
Chandrakala, C., Voleti, S.R., Bandeppa, S., Sunil Kumar, N., Latha, P.C., 2019. Silicate solubilization and plant growth promoting potential of Rhizobium sp. Isolated from rice rhizosphere. Silicon 11: 2895–2906.
Cruz, J.A., Tubana, B.S., Fultz, L.M., Dalen, M.S., Ham, J.H., 2022. Identification and profiling of silicate-solubilizing bacteria for plant growth-promoting traits and rhizosphere competence. Rhizosphere 23: 100566.
Das, S., Galgo, S.J., Alam, M.A., Lee, J.G., Hwang, H.Y., Lee, C.H., Kim, P.J., 2020b. Recycling of ferrous slag in agriculture: Potentials and challenges. Crit. Rev. Environ. Sci. Technol. 52: 1247-1281.
Das, S., Gwon, H.S., Khan, M.I., Jeong, S.T., Kim, P.J., 2020a. Steel slag amendment impacts on soil microbial communities and activities of rice (Oryza sativa L.). Sci. Rep. 10: 6746.
Das, S., Gwon, H.S., Khan, M.I., Van Nostrand, J.D., Alam, M.A., Kim, P.J., 2019. Taxonomic and functional responses of soil microbial communities to slag-based fertilizer amendment in rice cropping systems. Environ. Int. 127: 531-539.
Das, S., Hwang, H.Y., Song, H.J., Cho, S.R., Van Nostrand, J.D., Kim, P.J., 2021. Soil microbial response to silicate fertilization reduces bioavailable arsenic in contaminated paddies. Soil Biol. Biochem. 159: 108307.
Deshmukh, R., Sonah, H., Belanger, R.R., 2020. New evidence defining the evolutionary path of aquaporins regulating silicon uptake in land plants. J. Exp. Bot. 71: 6775-6788.
Elliott, C., Snyder, G.H., 1991. Autoclave-induced digestion for the colorimetric determination of silicon in rice straw. J. Agric. Food Chem. 39: 1118–1119.
Etesami, H., Schaller, J., 2023. Improving phosphorus availability to rice through silicon management in paddy soils: A review of the role of silicate-solubilizing bacteria. Rhizosphere, 100749.
Ghareeb, H., Bozsó, Z., Ott, P.G., Repenning, C., Stahl, F., Wydra, K., 2011. Transcriptome of silicon-induced resistance against Ralstonia solanacearum in the silicon non-accumulator tomato implicates priming effect. Physiol. Mol. Plant Pathol. 75: 83-89.
Hu, L., Xia, M., Lin, X., Xu, C., Li, W., Wang, J., Zeng, R., Song, Y., 2018. Earthworm gut bacteria increase silicon bioavailability and acquisition by maize. Soil Biol. Biochem. 125: 215–221.
Kang, S.M., Waqas, M., Shahzad, R., You, Y.H., Asaf, S., Khan, M.A., Lee, K.E., Joo, G. J., Kim, S.J., Lee, I.J., 2017. Isolation and characterization of a novel silicate-solubilizing bacterial strain Burkholderia eburnea CS4-2 that promotes growth of japonica rice (Oryza sativa L. cv. Dongjin). Soil Sci. Plant Nutr. 63: 233–241.
Lee, K.E., Adhikari, A., Kang, S.M., You, Y.H., Joo, G.J., Kim, J.H., Kim, S.J., Lee, I.J., 2019. Isolation and characterization of the high silicate and phosphate solubilizing novel strain Enterobacter ludwigii GAK2 that promotes growth in rice plants. Agronomy 9: 144.
Maleva, M., Borisova, G., Koshcheeva, O., Sinenko, O., 2017. Biofertilizer based on silicate solubilizing bacteria improves photosynthetic function of Brassica juncea. Agro. Sur. 2.
Manivannan, A., Ahn, Y.K., 2017. Silicon regulates potential genes involved in major physiological processes in plants to combat stress. Front. Plant Sci. 8: 1346.
Meena, V.D., Dotaniya, M.L., Coumar, V., Rajendiran, S., Ajay, Kundu, S., Subba Rao, A., 2014. A case for silicon fertilization to improve crop yields in tropical soils. Proceedings of the National Academy of Sciences, India Sec. B: Biol. Sci. 84: 505-518.
Mitter, E.K., Tosi, M., Obregón, D., Dunfield, K.E., Germida, J.J., 2021. Rethinking crop nutrition in times of modern microbiology: innovative biofertilizer technologies. Front. Sustain. Food Sys. 606815.
Ng, L.C., Anuar, S.N.A., Jong, J.W., Elham, M.S.H., 2016. Phytobeneficial and plant growth-promotion properties of silicon-solubilizing rhizobacteria on the growth and control of rice sheath blight disease. Asian J. Plant Sci. 15: 92–100.
Raturi, G., Sharma, Y., Rana, V., Thakral, V., Myaka, B., Salvi, P., Singh, M., Dhar, H., Deshmukh, R., 2021. Exploration of silicate solubilizing bacteria for sustainable agriculture and silicon biogeochemical cycle. Plant Physiol. Biochem. 166: 827-838.
Sharma, B., Kumawat, K.C., Tiwari, S., Kumar, A., Dar, R.A., Singh, U., Cardinale, M., 2022. Silicon and plant nutrition: Dynamics, mechanisms of transport, and role of silicon solubilizer microbiomes in sustainable agriculture. Pedosphere 33: 534-555.
Shivaraj, S.M., Mandlik, R., Bhat, J.A., Raturi, G., Elbaum, R., Alexander, L., Tripathi, D.K., Deshmukh, R., Sonah, H., 2022. Outstanding questions on the beneficial role of silicon in crop plants. Plant Cell Physiol. 63: 4-18.
Thorne, S.J., Hartley, S.E, Maathuis, F.J., 2020. Is silicon a panacea for alleviating drought and salt stress in crops?. Front. Plant Sci. 1221.
Vasanthi, N., Saleena, L., Raj, S.A., 2018. Silica solubilization potential of certain bacterial species in the presence of different silicate minerals. Silicon 10: 267–275.
Vijayapriya, M., Mahalakshmi, S., Prabudoss, V., Pandeeswari, N., 2019. Natural Efficiency of Bacillus Mucilaginosus on the Solubilization of Silicates. JPP.
Wang, R.R., Wang, Q., He, L.Y., Qiu, G., Sheng, X.F., 2015. Isolation and the interaction between a mineral-weathering Rhizobium tropici Q34 and silicate minerals. World J. Microbiol. Biotechnol. 31: 747–753.
Younas HS, Abid M, Shaaban M, Ashraf M. 2021. Influence of silicon and chitosan on growth and physiological attributes of maize in a saline field. Physiol. Mol. Biol. Plants. 27: 387–397.
Biofertilizers Based on Silicate-solubilizing Microbes for Crop Production and Protection
ABSTRACT
Silicon (Si), an element useful to plants, is abundant in soil, mostly in the form of insoluble silicates. Monosilicic acid, which is soluble, is the only form of Si that plants can take up. The bioavailability of Si reduces several biotic and abiotic stressors to plants and enhances their growth and productivity, while a deficiency of Si leads to abnormalities in plant growth and development. Synthetic silica-based fertilizers are expensive to produce, while the continuous and long-term application of silicate fertilizers can have harmful effects on the soil, as hazardous substances may deposit in the soil in addition to Si. In contrast, the application of biofertilizers based on silicate-solubilizing microbes could be an effective way not only to increase the bioavailability of Si but also to reap the benefits of Si for plants. In this context, this review addresses the distribution, isolation and characterization of silicate solubilizing microorganisms (SSMs), as well as the mechanism of Si solubilization and the promotion of plant growth by SSMs. In addition, crop production and protection of SSM-based biofertilizers are summarized, and problems and potential solutions for the use of SSM-based biofertilizers in agriculture are discussed. The findings presented here will be useful to better explore the potential of SSM-based biofertilizers in sustainable agriculture.
Keywords: Si-based fertilizer; Si bioavailability; phosphate solubilizing microbes; sustainable agriculture
INTRODUCTION
Silicon (Si) is the second most abundant element (~27%) in the Earth’s crust and occurs almost exclusively in the form of silicon dioxide (SiO2) in association with a wide range of Si-bearing minerals in crystalline, weakly crystalline, and amorphous phases. Although abundant in the Earth’s crust, Si is mostly in insoluble forms that are difficult for plants to absorb. Silicic acid (H4SiO4) is most common available form of Si in the soil. It becomes available mainly through the biogenic weathering and dissolution of the primary and secondary minerals (Sharma et al., 2022).
The Si uptake ability of plants is genetically controlled. According to the Si uptake ability, plants are divided into three categories: high accumulators (> 5% Si on a dry weight basis, such as rice, sugarcane, maize, bamboo, and sorghum), intermediate accumulators (about 1% Si, such as wheat, cucumber, chrysanthemum, and pumpkin), and low accumulators (< 1% Si, such as tomato and sunflower) (Meena et al., 2014). There has been a great deal of debate about the essentiality of Si to plants due to its abundance in soil, its accumulation pattern, and its observed positive effects on plants. Due to the lack of compelling data demonstrating its direct contribution to plant metabolism, it was ultimately excluded from the category of essential elements (Sharma et al., 2022). However, there is mounting evidence that Si application in agricultural fields can alleviate biotic and abiotic stress in plants and improve plant growth and yield (Raturi et al., 2021; Sharma et al., 2022). As a result, Si has been designated as a "quasi-essential" element and recognized as a beneficial nutrient. The International Plant Nutrition Institute (IPNI, www.ipni.net/nutrifacts), considering Si's numerous advantageous impacts on plants, has acknowledged Si as a beneficial nutrient for plant (Sharma et al., 2022).
Si fertilizer use may benefit both plants and soil in complementary ways. For the plant system, increased bioavailability of Si increases the deposition of Si in plant tissues, improves tissue rigidity, and helps the plant tolerate biotic stress (pest attack and pathogens) and abiotic stress (salinity, drought, nutrient imbalance, heavy metal and metalloid toxicity, heat and cold stress, and radiation stress) (Shivaraj et al., 2022). For the soil system, adequate availability of Si can improve the availability of other nutrients, especially phosphorus (P) and potassium (K), improve soil physicochemical properties (e.g., soil pH) and biological properties, and thus improve overall soil health and fertility (Das et al., 2019; Etesami and Schaller, 2023). Increasing bioavailability of Si has been reported to enhance photosynthesis and carbon (C) assimilation (Meena et al., 2014). The use of Si fertilizers in agricultural fields can minimize the use of pesticides (by 40–70%), mineral fertilizers (10–40%) and irrigation water (30–40%) without affecting crop productivity (Sharma et al., 2022). The beneficial effects of Si on the soil-plant system have been documented at the genetic level. For instance, there has been a growing body of evidence suggesting that Si plays multiple roles in the regulation of genes involved in plant photosynthesis, secondary metabolism, polyamine biosynthesis, transcription, and water absorption (Manivannan and Ahn, 2017). Physiological improvements in plant growth and recovery from stress were induced by molecular-level modulations triggered by Si supplementation (Manivannan and Ahn, 2017). Also, for the soil system, Si fertilization was reported to significantly change the composition of the bacterial and fungal community in the soil, increasing the abundance of functional genes involved in labile C degradation, C and N fixation, phosphorus utilization, CH4 oxidation, and metal detoxification, while decreasing genes involved in CH4 production and denitrification. The changes in the taxonomic and functional structure of microbial communities as a result of Si fertilization were mainly controlled by the changes in soil pH, plant photosynthesis, and Si nutrient availability induced by Si fertilization (Das et al., 2019, 2020a, 2021).
A variety of Si fertilizers, including natural, synthetic, liquid, and solid fertilizers, as well as various industrial by-products, are currently on the market. There are various types of Si fertilizers that are commercially available, including slag-based silicate fertilizers (calcium silicate fertilizers), fused magnesium phosphate fertilizers, fused potassium magnesium silicate fertilizers, porous hydrated calcium silicate fertilizers, silica gel, soluble silicates like sodium, potassium, and calcium silicates, and natural resources like wollastonite, olivine, diatomaceous earth, natural zeolite, and pyroxene (Das et al., 2020b; Raturi et al., 2021; Sharma et al., 2022). However, the most commonly used silicate fertilizers, water-soluble sodium silicate fertilizers and water-soluble potassium silicate fertilizers, are still too expensive to use in traditional agriculture. To benefit from Si's numerous advantages in agriculture, less expensive Si sources should be used. The application of Si fertilizers also causes numerous problems, including increased soil pH, declined digestibility of straw, and issue related to increased production costs (Thorne et al., 2020). In contrast, biofertilizers produced from competent silicate-solubilizing microorganisms (SSMs) signify a more environmentally sound and economically viable alternative to improve Si availability and hence agricultural output. Therefore, this review aims to evaluate the latest knowledge on SSMs, distribution, characterization and isolation of SSMs, the mechanism of Si solubilization by SSMs, the role of SSMs in crop production and protection, and limitations and future research priorities.
SILICATE-SOLUBILIZING MICROORGANISMS (SSMS): DISTRIBUTION, ISOLATION, AND CHARACTERIZATION
There have been reports that species belonging to the bacterial genera Bacillus, Paenibacillus, Acidobacillus, Pseudomonas, Proteus, Rhizobia, Burkholderia, Aminobacter, Enterobacter, and Sphingobacterium, and fungal genera such as Aspergillus, Trichoderma, and Penicillium can solubilize a variety of silicates, including magnesium silicate, quartz, feldspar, and others (Table 1).
Table 1. Silicate solubilizing microbes, their isolation source, characterization and effect on plant growth.
Silicate solubilizing microbes
Isolation source
Solubilized insoluble Si
PGP traits
Effects on plant
References
Burkholderia eburnea CS4-2
Rice rhizosphere
Magnesium trisilicate
Indole-3-acetic acid (IAA) production
Increased rice growth and yield
Kang et al. 2017
Enterobacter ludwigii GAK2
Paddy soil
Magnesium trisilicate
Phosphate (P) solubilization and production of IAA and gibberellic acid
Promoted rice seed germination and increased root length, shoot length, fresh biomass, and chlorophyll content of rice plants
Lee et al. 2019
Serratia marcescens and
Pseudomonas aeruginosa
Rice rhizosphere
Magnesium trisilicate
Phosphate solubilization and production of IAA
Increased rice growth and yield
Ng et al. 2016
Rhizobium sp. IIRR-1
Rice rhizosphere
Inorganic (Ca, Al, K and Mg), diatomaceous earth, siliceous earth, rice husk and, rice straw
Solubilization of phosphate, zinc, and potassium, and production of IAA and 1-aminocyclopropane-1-carboxylate deaminase
Improved rice seedling vigor
Chandrakala et al. 2019
Bacillus sp.
clay substrate of a mountain territory
Sodium metasilicate
-
Increased photosynthesis and total N, P, and K content in the leaves of Brassica. Juncea
Maleva et al. 2017
Pseudomonas and Bacillus sp., B. amyloliquefaciens, Sphingobacterium sp.
Rhizosphere soils
Magnesium trisilicate, talc, and feldspar
Production of organic acids (gluconic, succinic, fumaric, tartaric, and maleic acid)
Increased Si uptake, reduced disease severity, modulation of cell wall degrading and antioxidative enzyme activities, and induced defense responsive gene expression in rice plant
Bist et al. 2020
Aeromonas, Bacillus, Cellvibrio, Ensifer, Flavobacterium, Microbacterium, Paracoccus, Pseudomonas, Rhizobium, and Streptomyces
Gut and surrounding soil of earthworm
feldspar and quartz powder
-
Enhanced Si uptake and accumulation in maize plants and promoted seedling growth
Hu et al. 2018
Kosakonia sp.
Bryophyte Hypnum plumaeforme rhizoids
feldspar and quartz powder
-
Improved Si uptake and accumulation in maize plants, and promoted seedling growth
Hu et al. 2019
Rhizobium tropici
Weathered rocks
Feldspar and biotite
Production of organic acids and polysaccharides
-
Wang et al. 2015
Pseudomonas sp., P. frederiksbergensis, P. baetica,
P. stutzeri,
P. cremoricolorata, P. moorei,
P. plecoglossicida,
P. koreensis,
P. teessidea,
P. monteilii, Aeromonas sp.,
A. encheleia,
A. jandaei,
Bacillus pumilus, B. marisflavi,
B. ginsengisoli, and Enterobacter ludwigii
Rice rhizosphere
Magnesium trisilicate
Production of indole-3- acetic acid and 1-aminocyclopropane-1-carboxylate deaminase, N2 fixation, and the solubilization of phosphate
-
Cruz et al. 2022
Bacillus mucilaginosus
Rhizosphere soil of rice
Magnesium trisilicate
-
-
Vijayapriya et al. 2019
Bacillus flexus,
B. mucilaginosus, B. megaterium and Pseudomonas fluorescens
Soil samples from red soil, plantation soil, sea sand, pond sediment, sea water
Magnesium trisilicate, feldspar, calcium aluminosilicate, sodium aluminosilicate, talc, muscovite, illite and quartz
-
-
Vasanthi et al. 2018
Bacillus species (i.e., B. safensis, B. subtilis, B. caldolyticus, B. globisporus, B. stratosphericus, and B. cereus) and Pseudomonas species (i.e., P. fluorescens, P. alcaliphila, P. chengduensis, P. nitroreducens, P. veronii, and P. stutzeri) are the dominated silicate solubilizer found in environments. Silicon-solubilizing fungi (SSFs) include Aspergillus niger, Trichoderma sp., T. polysporum, T. viride, and T. pseudokoningii (Sharma et al. 2023). As a central component of the biogeochemical cycling of Si, SSMs are ubiquitous and primarily found in soils, water, aquatic sediment, mineral ores, weathered rocks, and the rhizospheres of plants. As rice and sugarcane require high levels of Si, the rhizospheres of these plants have been extensively investigated for silicate-solubilizing bacteria (SSBs). Silicon cycling and Si dynamics in forest ecosystems are reported to be responsible for Si deposition in aquatic environments such as rivers, streams, lakes, and oceans. However, isolation of SSMs from forest soils has not received much attention. Bamboo forests, for example, are considered a major source of bioavailable Si in rivers due to the hyper accumulative behavior of the bamboo plant. However, little effort has been devoted to identify SSMs from bamboo soils (Raturi et al., 2021). Deshmukh et al., (2020) documented 456 different plants that can uptake Si at high levels. This suggests that isolation of SSMs from the rhizosphere of these plants could be facilitated using such a comparative data set.
Although SSBs are ubiquitous, their numbers are very small compared to the large number of bacteria. Isolation of SSB involves several steps, such as collecting samples, purifying SSB, screening for microorganisms that dissolve insoluble silicate, and identifying the bacteria. Environments rich in Si, such as the rhizosphere of Si-accumulating plants (e.g., rice and sugarcane), Si-containing minerals, and Si-rich sediments are ideal for isolating SSB. After the sample (soil, sediment, or water) is collected, it is serially diluted in saline (typically 0.85%) and then an aliquot (0.1 mL) of the appropriate dilution is inoculated into the nutrient broth. A variety of growth media can be used to isolate SSB, such as culture medium enriched in magnesium trisilicate (Mg2O8Si3, 0.25%) to distinguish SSB from other bacteria (Raturi et al., 2021). In order to isolate SSB, researchers also employed glucose agar medium that contained insoluble silicate. A modified Bunt and Rovira medium supplemented with 0.25% magnesium trisilicate was used by several researchers to culture SSB. A nutrient medium ‘NBRISSM’, which contains magnesium trisilicate in concentrations ranging from 26.63 to 37.50 g ml-1 as a source of Si, can distinguish between SSB and other phosphate solubilizing bacteria. Zone formation on agar plates cultured for 7 days at 28 °C is often the basis for screening bacterial isolates for Si-solubilization. In addition, the Si solubilization potential of bacteria in broth medium can also be estimated by quantifying the Si released. A modified method of Elliott and Snyder (1991) described by Bist et al., (2020) is commonly used to screen SSB, where three volumes of 2.5% boric acid and an equal volume of 5.4% ammonium molybdate were added to the supernatant of 1, 3, 5, 7, and 10-day-old cultures in Si medium and then incubated for 5 min. 20% tartaric acid and 0.5% ascorbic acid sodium salt were added to the total volume of 1 ml and absorbance was measured spectrophotometrically at 650 nm (Bist et al., 2020).
To characterize SSB, polyphasic taxonomic approaches have been used, including physiological and biochemical tests. SSB colonies can be preliminarily examined for phenotypic characteristics such as cell shape, morphology, size, cell arrangement, Gram staining, and sporulation. The biochemical tests that can be performed to characterize SSB include catalase production, H2S production, motility, acid fast, glucose, fructose, sucrose, starch, and mannitol fermentability, urease testing, nitrate reduction, citrate utilization, organic acid quantification, and growth activity at various pH levels and temperatures. Various temperatures can be used to test the growth and activity of SSB. Most of the SSB strains grow well at temperatures ranging from 4°C to 45°C. Furthermore, the identification of SSBs can be accomplished by sequencing the 16S rRNA gene, which can be utilized to determine their phylogenetic relationships. An understanding of the mechanism of Si solubilization can be gained by quantifying the production of organic acid and IAA by SSB. Phylogenetic analysis of SSB will be supported by morphological, biochemical, and molecular characterizations.
MECHANISM OF SI SOLUBILIZATION AND PLANT GROWTH PROMOTION BY SSMS
SSMs use a number of interrelated methods to dissolve and solubilize silicates, including: (i) decreasing pH by organic and inorganic acid production; (ii) the production of chelating metabolites and ligands; and (iii) nucleophilic attack and exchange reactions (Etesami and Schaller, 2023). The most prevalent of these mechanisms is the production of acids by SSMs for solubilizing silica. Due to the acidic conditions created by SSMs, the dissolving rate of silicates is shifted out of equilibrium, resulting in an accelerated process of silicate solubilization. The kind of organic acid produced is influenced by the diversity of the SSB; it has been found that succinic, citric, maleic, gluconic, fumaric, acetic, tartaric, and hydroxy propionic acids are involved in silicate solubilization in media containing feldspar, quartz, and magnesium trisilicates as Si sources (Raturi et al., 2021). Microbial metabolites, such as organic acids, extracellular enzymes, chelates, and siderophores weaken the insoluble silicate framework by forming complexes with cations on the mineral surface, and hence play a significant role in the solubilization of silicates. Various polymers produced by SSM, such as extracellular proteins and polysaccharides, can form biofilms and help microorganisms attach to mineral surfaces, thereby affecting mineral dissolution. Indirectly, biofilms prevent protons, ligands, organic acids and other ions from escaping the interface between minerals and water, which not only increases water retention capabilities but also enhances mineral weathering (Sharma et al., 2023). Other than exopolymers, elements of bacterial cell membranes such lipopolysaccharides, peptidoglycan, and teichoic acids can bind to inorganic silicate ions to aid solubilization. A study conducted recently by Bist et al., (2020) demonstrated a functional association between the formation of organic acids and the activity of acidic phosphatases in the solubilization of Si. Recent developments in cutting-edge next generation sequencing (NGS) allow for the prediction of genes implicated in the metabolic pathways of silicate solubilizing ligands, acids, exopolymers, and membrane transporters. For silicate mineral weathering, these topics can be further investigated.
The potential of SSMs to improve crop growth and yield is based on the ability of SSMs to release Si and other nutrients such as phosphorus (P) and potassium (K), PGP traits (e.g., phytohormone synthesis, phosphate solubilization, siderophore production, and N2 fixation), and biocontrol traits of SSMs that support plant survival under adverse conditions and improve plant growth and yield. In a recent review, Etesami and Schaller (2023) reported that: (i) Si availability increases dissolution of iron phosphates and release of P into soil solution by replacing phosphate at the surface of amorphous and crystalline iron phases (decreased P sorption by soil minerals); (ii) Si increases P availability in paddy soils by increasing the fraction of Fe (II) (e.g., vivianite) compared to the fraction of Fe(III) (e. g., goethite, ferrihydrite, lepidocrocite, or hematite), which have a higher tendency to bind P; (iii) Si increases the root organic anion (e.g., citric, acetic, aconitic, malic, lactic, oxalic, succinic, and fumaric acids) to mobilize inorganic phosphate in the rhizosphere by competing with P to form complexes with Al3+, Ca2+, and Fe3+ and lowering rhizosphere pH; and (iv) Si availability enhances P utilization in rice by reducing iron and manganese uptake (e.g., by down-regulating the expression of Mn transporter gene that have the high affinity to bind phosphates in plants). Studies also show that Si increases K content in shoots and roots of wheat plants and enhances survival of barley and sugarcane plants against salt stress by minimizing K production, although the underlying mechanism is unknown (Raturi et al., 2021). Brindavathy et al., (2012) reported that the application of a SSB (Bacillus edaphicus) with different Si sources improves sugarcane yield due to the continuous supply of Si by the SSB. According to Kang et al., (2017), Burkholderia eburnea CS4-2, which was isolated from the roots of japonica rice, has the capacity to produce IAA and solubilize Si, and when applied to rice plants, it improves growth characteristics and yield. Lee et al., (2019) reported that Enterobacter sp. isolated from a healthy rubber plantation produced organic acids (citric, acetic, and lactic acids), indole-3-acetic acid, and gibberellic acids (GA1 and GA3), solubilized Si, P, and K, and possessed an antagonist against Rigidoporous microporus (causal agent of white root rot of rubber trees) (Table 1).
CROP PRODUCTION AND PROTECTION WITH SSM-BASED BIOFERTILIZERS
SSM-based biofertilizers can improve crop production and protection by improving both soil and plant properties, such as nutrient availability (P, K, Si), soil properties (pH, CEC, etc.), root exudate production, root oxidative capacity, photosynthetic activity, water status, phytohormone biosynthesis, nutrient uptake, phenolic compound metabolism, antioxidant potential, lodging, pest and pathogen resistance, tolerance to extreme temperatures (heat and cold), drought, salinity, oxidative damage, UV-B protection, and metal and metalloid stress. (Figure 1).
Fertilization with SSMs is a suitable approach for an integrated nutrient balance and a circular economy-model for managing agricultural waste, especially when combined with natural Si sources like grain husk and straw. A few Si-based biofertilizers have been developed and applied, but they are rare in practice. SSB and farmyard manure (FYM), with or without varying levels of fly ash, have been shown to increase Si uptake and concentrations in the straw and grain tissues in rice and the grain yield (Vasanthi et al., 2018). The release of cations (Ca2+ and Mg2+) as well as the volume of plastid material and photosynthetic pigments in leaves were all positively influenced by the use of SSM-based biofertilizers in combination with clay substrates in Brassica juncea (Indian mustard) and other crops (Maleva et al., 2017) (Table 1). According to reports, SSMs boost maize, wheat, tomato, and potato yields and rhizospheric microbial population (Raturi et al., 2021; Sharma et al., 2022). Therefore, SSM-based biofertilizer can be used as an efficient and environmentally benign fertilizer in organic and sustainable agriculture to improve crop production and reclaim infertile or damaged soils.
Silicon-based biofertilizers have been shown to reduce biotic as well as abiotic stresses like drought, high temperatures, ultraviolet radiation, lodging, freezing, salinity, nutrients imbalance, metal toxicity, pest and phytopathogen infestations like rice blast, powdery mildew, brown spot, stem rot, and sheath brown rot (Raturi et al., 2021; Sharma et al., 2022). The SSM controls abiotic stress by regulating antioxidant enzymes and decreasing free radicals to protect the oxidative defense system. After application of Si (80 kg ha-1) and chitosan (30 kg ha-1), Younas et al., (2021) found a significant increase in antioxidant/defense enzymes such as superoxide dismutase (SOD), peroxidase (POD), and catalase (CAT), indicating that Si fertilization eliminates reactive oxygen species (ROS) and reduces the severity of structural and functional damage in plants. Silicon availability has been shown to modify the physical properties of plant cell walls, such as loosening cells and cell processes to store more water and produce more stable lipids that help plants cope with drought, heat, and salt stress. In addition, Si accumulation can trigger specific defense responses, such as the formation of a cuticle-Si bilayer in epidermal cells, the formation of papillae, and the production of fungitoxic phenolic compounds such as flavonoids that protect plants from pest attack (Younas et al., 2021). Physical barriers prevent the spread of host-infecting toxins and lytic enzymes secreted by pathogens. It was shown that Si modify specific genes in a number of host-pathogen relationships, including those involving rice and Magnaporthe oryzae (Brunings et al., 2009), tomato and Rhizoctonia solani (Ghareeb et al., 2011), and wheat and Buleria graminis (Belanger et al., 2007). The wide range of prophylactic traits provided by SSMs reduce a wide range of abiotic stresses, such as drought, high temperature, and metal toxicity, and biotic stresses, such as rice blast, powdery mildew, brown spot, stem rot, rice yellow stem borer, and sheath brown rot, while promoting P, Mg, K, Fe, Cu, and Zn uptake by rice plants (Sharma et al., 2022). A nano-biofertilizer containing Si has been recently reported to improve nutrient (Mn, S, P, and K) translocation from soil to plant, minimize heavy metal uptake in food crops, and reduce UV stress. Silicon nano-biofertilizers containing SSBs such as Mesorhizobium sp. and Pseudomonas sp. have been shown to increase plant growth, soil structure, and fertility, and have been proposed as inventive Si sources with a variety of applications, including increasing plant tolerance to biotic and abiotic stressors, wastewater treatment, food processing, nano-additives in the paper industry, biosensors, and biomarkers (Sharma et al., 2022).
USE OF SSM-BASED BIOFERTILIZER IN AGRICULTURE: CONCERNS AND POTENTIAL SOLUTIONS
In order to introduce biofertilizer into the field, including SSM-based biofertilizer, a number of hurdles first have to be eliminated, including those related to survival, establishment, colonization, and plant interaction (for example, parasitic/symbiotic behavior, PGP performance) (Mitter et al., 2021). This is especially concerning when moving from the lab to the field since it frequently happens that a microbial strain that performed well in vitro may perform poorly in greenhouse and/or field trials. Because we generally consider and control a limited number of variables, we don't often take into account their complicated interactions when predicting inoculation outcomes. Possible solutions for large-scale production and success of biofertilizers in the field include: (i) pre-adapted microorganisms (e.g., locally isolated), isolation and screening with emphasis on PGP and ecological traits; (ii) mixed inoculants with functional redundancy but a wide range of environmental adaptations; (iii) personalized biofertilizers for a specific farm (e.g.., for a specific soil, crop, and management); (iv) biofertilizers based on an optimal performance spectrum; (v) genotype-specific inoculants (e.g., compatible microorganisms, pre-adapted microbiome); and (vi) optimized application timing.
CONCLUSIONS
The application of SSM-based biofertilizers to the soil may open new opportunities to increase crop production and transition to sustainable agriculture. It is currently unclear what molecular process enables different SSMs to solubilize Si. SSMs belonging to various genera and occurring in diverse environments suggest that different mechanisms of Si solubilization are involved. Future studies should focus on the biochemical and molecular basis of the mechanism of silicate solubilization. In addition to silicate solubilization, SSMs can also dissolve P and K. They may be beneficial to plants through the synthesis of organic acids such as IAA and their antagonistic activity against pathogens. SSMs can accelerate the biodegradation or composting of silicate-containing crop residues and convert them to a bioavailable form of Si. Therefore, SSM-mediated increase in Si bioavailability will contribute to reduced pesticide use and improved plant health and will be a milestone in sustainable agriculture.
REFERENCES
Bélanger, R.R., Benhamou, N., Menzies, J.G., 2003. Cytological evidence of an active role of silicon in wheat resistance to powdery mildew (Blumeria graminis f. sp. tritici). Phytopathology 93: 402-412.
Bist, V., Niranjan, A., Ranjan, M., Lehri, A., Seem, K., Srivastava, S., 2020. Silicon-solubilizing media and its implication for characterization of bacteria to mitigate biotic stress. Front. Plant Sci. 11: 28.
Brindavathy, R., Dhara, N., Rajasundari, K., 2012b. Biodissolution of silica by silicon bacteria in sugarcane rhizosphere. Res. J. Agr. Sci 3: 1042–1044.
Brunings, A.M., Datnoff, L.E., Ma, J.F., Mitani, N., Nagamura, Y., Rathinasabapathi, B., Kirst, M., 2009. Differential gene expression of rice in response to silicon and rice blast fungus Magnaporthe oryzae. Ann. Appl. Biol. 155: 161-170.
Chandrakala, C., Voleti, S.R., Bandeppa, S., Sunil Kumar, N., Latha, P.C., 2019. Silicate solubilization and plant growth promoting potential of Rhizobium sp. Isolated from rice rhizosphere. Silicon 11: 2895–2906.
Cruz, J.A., Tubana, B.S., Fultz, L.M., Dalen, M.S., Ham, J.H., 2022. Identification and profiling of silicate-solubilizing bacteria for plant growth-promoting traits and rhizosphere competence. Rhizosphere 23: 100566.
Das, S., Galgo, S.J., Alam, M.A., Lee, J.G., Hwang, H.Y., Lee, C.H., Kim, P.J., 2020b. Recycling of ferrous slag in agriculture: Potentials and challenges. Crit. Rev. Environ. Sci. Technol. 52: 1247-1281.
Das, S., Gwon, H.S., Khan, M.I., Jeong, S.T., Kim, P.J., 2020a. Steel slag amendment impacts on soil microbial communities and activities of rice (Oryza sativa L.). Sci. Rep. 10: 6746.
Das, S., Gwon, H.S., Khan, M.I., Van Nostrand, J.D., Alam, M.A., Kim, P.J., 2019. Taxonomic and functional responses of soil microbial communities to slag-based fertilizer amendment in rice cropping systems. Environ. Int. 127: 531-539.
Das, S., Hwang, H.Y., Song, H.J., Cho, S.R., Van Nostrand, J.D., Kim, P.J., 2021. Soil microbial response to silicate fertilization reduces bioavailable arsenic in contaminated paddies. Soil Biol. Biochem. 159: 108307.
Deshmukh, R., Sonah, H., Belanger, R.R., 2020. New evidence defining the evolutionary path of aquaporins regulating silicon uptake in land plants. J. Exp. Bot. 71: 6775-6788.
Elliott, C., Snyder, G.H., 1991. Autoclave-induced digestion for the colorimetric determination of silicon in rice straw. J. Agric. Food Chem. 39: 1118–1119.
Etesami, H., Schaller, J., 2023. Improving phosphorus availability to rice through silicon management in paddy soils: A review of the role of silicate-solubilizing bacteria. Rhizosphere, 100749.
Ghareeb, H., Bozsó, Z., Ott, P.G., Repenning, C., Stahl, F., Wydra, K., 2011. Transcriptome of silicon-induced resistance against Ralstonia solanacearum in the silicon non-accumulator tomato implicates priming effect. Physiol. Mol. Plant Pathol. 75: 83-89.
Hu, L., Xia, M., Lin, X., Xu, C., Li, W., Wang, J., Zeng, R., Song, Y., 2018. Earthworm gut bacteria increase silicon bioavailability and acquisition by maize. Soil Biol. Biochem. 125: 215–221.
Kang, S.M., Waqas, M., Shahzad, R., You, Y.H., Asaf, S., Khan, M.A., Lee, K.E., Joo, G. J., Kim, S.J., Lee, I.J., 2017. Isolation and characterization of a novel silicate-solubilizing bacterial strain Burkholderia eburnea CS4-2 that promotes growth of japonica rice (Oryza sativa L. cv. Dongjin). Soil Sci. Plant Nutr. 63: 233–241.
Lee, K.E., Adhikari, A., Kang, S.M., You, Y.H., Joo, G.J., Kim, J.H., Kim, S.J., Lee, I.J., 2019. Isolation and characterization of the high silicate and phosphate solubilizing novel strain Enterobacter ludwigii GAK2 that promotes growth in rice plants. Agronomy 9: 144.
Maleva, M., Borisova, G., Koshcheeva, O., Sinenko, O., 2017. Biofertilizer based on silicate solubilizing bacteria improves photosynthetic function of Brassica juncea. Agro. Sur. 2.
Manivannan, A., Ahn, Y.K., 2017. Silicon regulates potential genes involved in major physiological processes in plants to combat stress. Front. Plant Sci. 8: 1346.
Meena, V.D., Dotaniya, M.L., Coumar, V., Rajendiran, S., Ajay, Kundu, S., Subba Rao, A., 2014. A case for silicon fertilization to improve crop yields in tropical soils. Proceedings of the National Academy of Sciences, India Sec. B: Biol. Sci. 84: 505-518.
Mitter, E.K., Tosi, M., Obregón, D., Dunfield, K.E., Germida, J.J., 2021. Rethinking crop nutrition in times of modern microbiology: innovative biofertilizer technologies. Front. Sustain. Food Sys. 606815.
Ng, L.C., Anuar, S.N.A., Jong, J.W., Elham, M.S.H., 2016. Phytobeneficial and plant growth-promotion properties of silicon-solubilizing rhizobacteria on the growth and control of rice sheath blight disease. Asian J. Plant Sci. 15: 92–100.
Raturi, G., Sharma, Y., Rana, V., Thakral, V., Myaka, B., Salvi, P., Singh, M., Dhar, H., Deshmukh, R., 2021. Exploration of silicate solubilizing bacteria for sustainable agriculture and silicon biogeochemical cycle. Plant Physiol. Biochem. 166: 827-838.
Sharma, B., Kumawat, K.C., Tiwari, S., Kumar, A., Dar, R.A., Singh, U., Cardinale, M., 2022. Silicon and plant nutrition: Dynamics, mechanisms of transport, and role of silicon solubilizer microbiomes in sustainable agriculture. Pedosphere 33: 534-555.
Shivaraj, S.M., Mandlik, R., Bhat, J.A., Raturi, G., Elbaum, R., Alexander, L., Tripathi, D.K., Deshmukh, R., Sonah, H., 2022. Outstanding questions on the beneficial role of silicon in crop plants. Plant Cell Physiol. 63: 4-18.
Thorne, S.J., Hartley, S.E, Maathuis, F.J., 2020. Is silicon a panacea for alleviating drought and salt stress in crops?. Front. Plant Sci. 1221.
Vasanthi, N., Saleena, L., Raj, S.A., 2018. Silica solubilization potential of certain bacterial species in the presence of different silicate minerals. Silicon 10: 267–275.
Vijayapriya, M., Mahalakshmi, S., Prabudoss, V., Pandeeswari, N., 2019. Natural Efficiency of Bacillus Mucilaginosus on the Solubilization of Silicates. JPP.
Wang, R.R., Wang, Q., He, L.Y., Qiu, G., Sheng, X.F., 2015. Isolation and the interaction between a mineral-weathering Rhizobium tropici Q34 and silicate minerals. World J. Microbiol. Biotechnol. 31: 747–753.
Younas HS, Abid M, Shaaban M, Ashraf M. 2021. Influence of silicon and chitosan on growth and physiological attributes of maize in a saline field. Physiol. Mol. Biol. Plants. 27: 387–397.